Left ventricular assist devices—current state and perspectives
Introduction
Worldwide a constant decline of heart transplantations (HTx) is observed. While the numbers of patients awaiting HTx doubled within the last 15 years, available donor organs dropped by a third. In 2015, 790 patients in Germany were listed for HTx with only 286 hearts to be transplanted. A decline in one-year survival after HTx from 85% to 76% in the EuroTransplant region is observed, presumably because of increasing donor age and recipient comorbidity (1). About 15% of patients listed for transplantation die before an organ is available and more than 30% of patients awaiting HTx need mechanical circulatory support with left ventricular assist devices (LVAD) as bridge to transplant (BTT) (2). Therefore, the need for permanent mechanical circulatory support assist devices has increased. In the last decade LVAD systems underwent substantial progress in size, durability, reliability and noise emission. LVAD implantation became a new treatment option for end stage heart failure as destination therapy (DT) for patients either too old or not suitable for transplantation due to other medical conditions (3). As a result, an exponential increase of LVAD implantations took place within the last five years. Some devices have already been implanted over 10,000 times.
Development of LVAD
The history of mechanical circulatory support began in 1953, as the first heart lung machine enabled surgeons to perform complex open-heart surgery (4). For treatment of low cardiac output after operations with cardiopulmonary bypass, simple pumps for temporary circulatory support were developed (5). In 1964 funding for the “Artificial Heart Program” by the National Heart, Lung, and Blood Institute in the US began to support development of devices for long-term clinical use. In 1966, DeBakey and colleagues implanted the first pneumatically driven LVAD (6). In 1969, Denton A. Cooley implanted the first total artificial heart (TAH) intended as a BTT in a patient awaiting HTx (7). In the 1970s, focus shifted to develop more biocompatible systems for long-term therapy. For the first time in 1982 the JARVIK-7 TAH was implanted as intended permanent treatment, but after 112 days the patient died of severe sepsis resulting in multi organ failure (8). Due to the high rate of complications, the concept of TAH was never established as a real alternative and to date the proportion of TAH in mechanical assist devices is below 1% (2).
First generation ventricular assist devices
A shift from the concept of TAH as heart replacement, towards the development of single chamber pumps as cardiac support, initiated the area of ventricular assist devices (VAD). These VADs generated additional blood flow in parallel with the particular ventricle. First generation VADs were either pneumatically or electrically driven membrane pumps, generating pulsatile flow with artificial heart valves as inlet and outlet. Examples are Berlin Heart EXCOR (Berlin Heart, Berlin, Germany), Thoratec PVAD and XVE (Thoratec, Pleasanton, CA, USA). Connected to the heart via cannulas, these pumps can be used either as isolated left-, right- or biventricular assist devices. If used for biventricular support, pump chambers have to be positioned extracorporeal due to size. For simple left ventricular support intracorporeal placement is possible, depending on the type of VAD (Figure 1A).
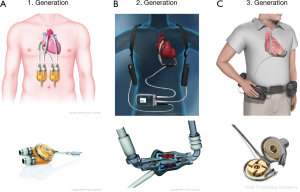
Initially these systems were designed only as BTT. The first successful transplantation after LVAD-implantation was performed in 1984 (9). Over the years, miniaturization of the devices created new possibilities: more patients could be discharged on VAD, still being listed and awaiting transplantation. However, first generation VADs had several disadvantages: large size, noise emission, infections of cannulas and malfunction induced by tears in the membrane or degradation of valves made everyday life difficult and sometimes caused fatal complications.
Second generation LVAD
In the 1990’s development of continuous flow centrifugal pump devices improved patient outcome by reducing size and susceptibility for infections (Figure 1B). In addition, significant noise reduction enhanced quality of life. Designed exclusively for intrathoracic implantation, only utilization as LVAD was possible, as the devices were too large to be used as BIVAD. The most frequently used second generation LVAD is the Heartmate II (Thoratec, Pleasanton, CA, USA). The device consists of a propeller surrounded by a metal case, referred to as impeller. The combined mechanical and magnetical positioning of the impeller increases the durability up to a minimum of five years (10). Since FDA approval in 2008, the Heartmate II can be used either as BTT or since 2010 as DT. This provides patients with a better quality of life, including good mobility and restoration of endorgan function, in some cases even allowing them to return to work.
Third generation LVAD
With introduction of third generation LVADs another significant reduction in size could be achieved (Figure 1C). Leading example is the LVAD by HeartWare (HeartWare Inc., Framingham, MA, USA). Due to its reduced size, even a biventricular implantation is possible. Designed as radial pump with magnetic and hydraulic positioning, no wear-out is to be expected with an estimated durability of 10 years. Second and third generation VADs can be implanted without full sternotomy via bilateral thoracotomy (11). The devices mentioned above can produce a flow up to 10 L/min, taking over complete circulatory support. With the HeartMate 3 by Thoratec another 3rd generation LVAD is available for treatment of end stage heart failure (Figure 2). As a new feature, this LVAD can generate pulsatile flow patterns by regular changes of rotor speed. Thereby blood stasis in the left ventricle and the device shall be avoided, limiting the risk of hemorrhagic or thrombotic complications. In addition miniaturized systems with flow capacities of maximum 3 L/min can be used for partial circulatory support. Clinical example is the CircuLite-System, with an inflow-cannula positioned in the right atrium and an outflow-cannula draining in the right subclavian artery. Implantation could be performed trough a right-sided mini thoracotomy without use of a heart lung machine.
Indications and risk assessment
Successful long term results after implantations of LVADs are highly dependent on the timing of implantation. LVAD implantation is indicated in patients with end stage heart failure (12,13). The criteria for LVAD implantation are NYHA class 4 heart failure refractory to optimal medical therapy, LVEF less than 25%, systolic blood pressure <80 mmHg, pulmonary capillary wedge pressure >20 mmHg, cardiac index <2.0 L/min/m2 despite continuous intravenous inotropic therapy and intra-aortic counterpulsation (14,15). In addition, malignant cardiac arrhythmias as well as any patient on transplant waiting list can be considered for LVAD-therapy.
Patients with advanced congestive heart failure pose a challenge. Clinicians must monitor symptoms closely to identify the right timing for implantation. This greatly influences the patient outcome after implantation. If the LVAD is implanted too early, benefits of medical treatment with potential recovery of heart function are not fully exhausted. If the LVAD is implanted too late, the outcome may worsen due to secondary organ damage caused by prolonged heart failure. Studies showed that regardless of the age of the recipient, severity of left ventricular failure and severe deterioration of the general medical condition pose a high postoperative risk after LVAD-implantation. Secondary organ impairment can be regularly observed in patients with end-stage heart failure and should be carefully assessed during the selection process. Poor nutritional status, represented by low serum albumin, impaired liver- and renal function and markers of right heart failure are independent predictors for increased risk after implantation. Based on data from 280 patients with first generation LVAD, Lietz and colleagues identified nine factors (i.e., platelet number, serum albumin, INR) for risk stratification (Table 1) (16). In low risk patients, represented by low scores, one-year survival was more than 81%, in contrast to high-risk patients with high scores and a one-year survival of 10.7% (Table 2).
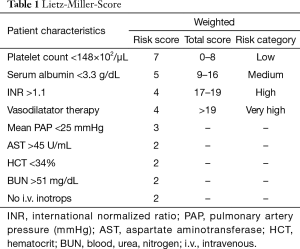
Full table
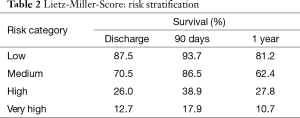
Full table
An additional challenge is to determine whether the patient needs right ventricular (RV) support as well. The sicker the patient, the higher the likelihood of right ventricular failure. Up to 20–40% of patients show RV failure after LVAD implantation. Cardiac index below 2.2 L/min/m2, RV stroke index below 0.25 mmHg/L/m2, severe RV dysfunction during preoperative assessment, preoperative creatinine above 1.9 mg/dL and previous cardiac surgery are strong predictors of RVAD need (15).
Contraindications for LVAD implantation are increased risk of bleeding, non-reversible damage of lung, liver and kidneys, cerebral ischemia, active infection, aortic regurgitation, prior implantation of a mechanical aortic valve prosthesis, severe right heart failure (for isolated LVAD) and non-compliance.
A Registry collecting data from patients receiving mechanical circulatory support from index hospitalization and follow-up evaluation after implantation is the Interagency Registry of Mechanical Assisted Circulatory Support (INTERMACS). It was established in 2005 and categorizes patients awaiting LVAD implantation on a scale from 1 (cardiogenic shock) to 7 (advanced NYHA III), as depicted in Table 3 (17). Long-term survival is best for patients with INTERMACS level 3 (‘stable with inotropic support’) and worst for patients with INTERMACS level 1 (18).
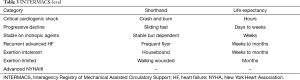
Full table
Outcome
Studies showed that overall chance of survival is increased with LVAD therapy compared to pharmacological treatment and approximates the results of HTx.
In 2001, Rose and colleagues published a randomized controlled trial (RCT) investigating the long-term use of a pulsatile left ventricular assist device for end-stage heart failure (REMATCH trial). In this trial they assigned a total of 129 patients either to treatment with LVAD (68 patients) or optimal medical therapy (61 patients). Comparing the rates of survival after one year, a significant improvement in survival could be shown for patients receiving treatment with LVAD (52%) compared to best medical treatment (25%). After two years of follow-up, survival rates were still significantly better in the LVAD group (23% vs. 8%). Although an increased incidence of serious adverse events like infection, stroke and malfunction of the device was observed in the LVAD group, quality of life was significantly improved in these patients (19).
Slaughter and coworkers compared in another RCT 1st generation devices with pulsatile flow and 2nd generation devices with continuous flow. 134 patients were assigned to receive a 2nd generation LVAD and 66 patients received a 1st generation device. Primary composite endpoint was survival and freedom from stroke or reoperation after two years. Survival, frequency of adverse events, quality of life and functional capacity defined the secondary endpoints. In both groups, quality of life and functional capacity improved significantly. With regard to primary and secondary endpoints, a superiority of second-generation devices was observed (46% vs. 11% and 58% vs. 24%, respectively) (12).
With 3rd generation LVADs, rates of survival and freedom from adverse events are still increasing. In the multi-center prospective ReVOLVE-trial a total of 254 patients received a HeartWare device and demonstrated good survival rates after six months (87%), one (85%), two (79%) and three years (73%) (20).
Similar results were reported for the new HeartMate III: 50 patients were enrolled between June and November 2014 in a multicenter, prospective trial to evaluate safety and efficacy of this 3rd generation LVAD. Survival rates were compared with a matched patient group, derived from the annual INTERMACS report. With a 6-month survival rate of 92% the device exceeded the performance goal with reexploration rates for bleeding (14%), driveline infections (10%), gastrointestinal bleeding (8%) and stroke (8%) (21).
The use of CircuLite for partial circulatory support for less sick patients was under investigation. In a small collective a hemodynamic impact was already demonstrated (22), but the device was withdrawn from the market due to technical issues. Technical refinements are carried out at the moment.
The most current data are annually presented in the INTERMACS report. Since registration in 2006, a total of 15,745 implantations of VAD’s were entered in the INTERMACS database until 2013. Today, the annual implantation of continuous-flow devices increased to 96.8%. Thirty percent of patients received VAD as BTT, 46% as DT and 23% as bridge to candidacy in patients with an anticipated possibility of listing. If listed for HTx at time of implantation, 30% received an organ within the first year.
For patients undergoing continuous-flow VAD-implantation between 2008 and 2014, one-year survival is 76% at INTERMACS-level 1, 80% for INTERMACS-level 2–3 and 82% for patients with INTERMACS-level 4–7. Two-year survival for patients in level 4–7 is 72% and 4-year survival still 49%. The one- and two-year survival rates of LVAD therapy are almost comparable to HTx (23).
Leading causes of death are neurological events and multi-organ failure, contributing to up to a third of all deaths. Noticeable, fatal device malfunction occurred in 3.5%. Most common adverse events are bleeding (7.8%), cardiac arrhythmia (4.1%), infection (7.3%), respiratory failure (2.7%) and stroke (1.6%) (23). Some of the problems are caused by the design of the devices, i.e., membrane tears in pulsatile flow devices and could be reduced with introduction of 2nd- and 3rd-generation continuous flow devices (10). Other problems were addressed by subtle changes in detail, i.e., minimized thrombogenity by titanium-coated surfaces or pulsatile flow-patterns in 3rd generation devices to reduce clot formation with the possibility to reduce target INR levels.
A major problem associated with LVAD therapy is infection, especially driveline infections. They occur in up to 20% of all patients within the first year after implantation (24). Optimization of peri- and post-operative management and adjustments in driveline design led to a significant reduction of infections, but the problem could not be solved completely (25). Therefore, the focus was on developing transcutaneous energy transfer (TET) with the goal to allow transcutaneous recharging of batteries. Requirements for TET are high efficacy in energy transfer, moderate heat production and safe application. The launching of a fully functional device can be expected within the next years with prototypes already being tested (26).
With costs of around $85,000 per device, LVAD therapy currently comes at a high price. To account the value of money for a medical intervention, quality-adjusted life years (QUALY) have been introduced as a generic measure of disease burden, including both quality and quantity of life lived. To evaluate cost-effectiveness of interventions, incremental cost effectiveness ratio (ICER) compare costs of an intervention with the next most effective option (27). Patients undergoing HTx benefit from a significant improvement in life expectancy and costs are below $100,000 per QALY (28). Rogers and coworkers demonstrated in their study that patients receiving LVAD therapy with 2nd or 3rd generation devices gained in average 1.87 QALY or 2.42 life years (LY) with a total cost of $360,407. Patients treated with optimal medical therapy gained 0.37 QALY/0.64 LY at a cost of $62,856. This results in an ICER for continuous-flow LVAD compared to best medical treatment of $198,184/QALY or $167,208/LY. However, compared to first generation LVAD’s a substantial reduction of cost was already achieved: with pulsatile LVAD’s, estimation of total costs were $391,906 and QALY of 0.76 with an ICER of $802,647. The authors contribute these reduced costs to continuous-flow LVAD with improved survival, reduced implant costs and persistent improvement in functional abilities (29). Pulikottil-Jacob and colleagues compared cost-effectiveness of the HeartWare and HeartMate II. Patients treated with a HeartWare acquired 4.99 QALY at a cost of $410,970 and patients with a HeartMate II 3.84 QALS at $368,048 with a deterministic ICER for HeartWare vs. HeartMate II of $37,349. Therefore they concluded that the HeartWare offers a better cost-effectiveness (30).
Conclusions
With decreasing numbers of HTx due to limited donor organs, the importance of mechanical circulatory support in the therapy of end stage heart failure is continuously increasing. Technical improvements resulted in significant size reduction, performance optimization and enhanced clinical applicability. The improved durability and almost wear free components of second and third generation LVAD do not only allow usage as BTT, but also as DT, thus offering another treatment option to patients not suitable for transplantation.
Acknowledgements
None.
Footnote
Conflicts of Interest: The authors have no conflicts of interest to declare.
References
- Smits JM. Actual situation in Eurotransplant regarding high urgent heat transplantation. Eur J Cardiothorac Surg 2012;42:609-11. [Crossref] [PubMed]
- ISHLT heart transplantation 2013 adult heart transplants. % of Patients Bridged with Mechanical Circulatory Support by Year and Device Type. The International Society for Heart & Lung Transplantation. Available online: http://www.ishlt.org/registries/slides.asp?slides=heartLungRegistry&year%20=2013
- Stewart GC, Givertz MM. Mechanical circulatory support for advanced heart failure: patients and technology in evolution. Circulation 2012;125:1304-15. [Crossref] [PubMed]
- Gibbon JH Jr. Application of a mechanical heart and lung apparatus to cardiac surgery. Minn Med 1954;37:171-85. [PubMed]
- Liotta D, Hall CW, Henly WS, et al. Prolonged assisted circulation during and after cardiac or aortic surgery. Prolonged partial left ventricular bypass by means of intracorporeal circulation. Am J Cardiol 1963;12:399-405. [Crossref] [PubMed]
- DeBakey ME. Left ventricular bypass pump for cardiac assistance. Clinical experience. Am J Cardiol 1971;27:3-11. [Crossref] [PubMed]
- Cooley DA, Liotta D, Hallman GL, et al. Orthotopic cardiac prosthesis for two-staged cardiac replacement. Am J Cardiol 1969;24:723-30. [Crossref] [PubMed]
- DeVries WC, Anderson JL, Joyce LD, et al. Clinical use of the total artificial heart. N Engl J Med 1984;310:273-8. [Crossref] [PubMed]
- Portner PM, Oyer PE, McGregor CG, et al. First human use of an electrically powered implantable ventricular assist system. Artif Organs 1985.936-41.
- Moazami N, Fukamachi K, Kobayashi M, et al. Axial and centrifugal continuous-flow rotary pumps: a translation from pump mechanics to clinical practice. J Heart Lung Transplant 2013;32:1-11. [Crossref] [PubMed]
- Schmitto JD, Molitoris U, Haverich A, et al. Implantation of a centrifugal pump as a left ventricular assist device through a novel, minimized approach: upper hemisternotomy combined with anterolateral thoracotomy. J Thorac Cardiovasc Surg 2012;143:511-3. [Crossref] [PubMed]
- Slaughter MS, Rogers JG, Milano CA, et al. Advanced heart failure treated with continuous-flow left ventricular assist device. N Engl J Med 2009;361:2241-51. [Crossref] [PubMed]
- Miller LW, Pagani FD, Russell SD, et al. Use of a continuous-flow device in patients awaiting heart transplantation. N Engl J Med 2007;357:885-96. [Crossref] [PubMed]
- Williams MR, Oz MC. Indications and patient selection for mechanical ventricular assistance. Ann Thorac Surg 2001;71:S86-91. [Crossref] [PubMed]
- Fitzpatrick JR 3rd, Frederick JR, Hsu VM, et al. Risk score derived from pre-operative data analysis predicts the need for biventricular mechanical circulatory support. J Heart Lung Transplant 2008;27:1286-92. [Crossref] [PubMed]
- Lietz K, Long JW, Kfoury AG, et al. Outcomes of left ventricular assist device implantation as destination therapy in the post-REMATCH era: implications for patient selection. Circulation 2007;116:497-505. [Crossref] [PubMed]
- Stevenson LW, Pagani FD, Young JB, et al. INTERMACS profiles of advanced heart failure: the current picture. J Heart Lung Transplant 2009;28:535-41. [Crossref] [PubMed]
- Slaughter MS, Pagani FD, Rogers JG, et al. Clinical management of continuous-flow left ventricular assist devices in advanced heart failure. J Heart Lung Transplant 2010;29:S1-39. [Crossref] [PubMed]
- Rose EA, Gelijns AC, Moskowitz AJ, et al. Long-term use of a left ventricular assist device for end-stage heart failure. N Engl J Med 2001;345:1435-43. [Crossref] [PubMed]
- Strueber M, Larbalestier R, Jansz P, et al. Results of the post-market Registry to Evaluate the HeartWare Left Ventricular Assist System (ReVOLVE). J Heart Lung Transplant 2014;33:486-91. [Crossref] [PubMed]
- Netuka I, Sood P, Pya Y, et al. Fully Magnetically Levitated Left Ventricular Assist System for Treating Advanced HF: A Multicenter Study. J Am Coll Cardiol 2015;66:2579-89. [Crossref] [PubMed]
- Klotz S, Meyns B, Simon A, et al. Partial mechanical long-term support with the CircuLite Synergy pump as bridge-to-transplant in congestive heart failure. Thorac Cardiovasc Surg 2010;58 Suppl 2:S173-8. [Crossref] [PubMed]
- Kirklin JK, Naftel DC, Pagani FD, et al. Seventh INTERMACS annual report: 15,000 patients and counting. J Heart Lung Transplant 2015;34:1495-504. [Crossref] [PubMed]
- Goldstein DJ, Naftel D, Holman W, et al. Continuous-flow devices and percutaneous site infections: clinical outcomes. J Heart Lung Transplant 2012;31:1151-7. [Crossref] [PubMed]
- Sharma V, Deo SV, Stulak JM, et al. Driveline infections in left ventricular assist devices: implications for destination therapy. Ann Thorac Surg 2012;94:1381-6. [Crossref] [PubMed]
- Slaughter MS, Myers TJ. Transcutaneous energy transmission for mechanical circulatory support systems: history, current status, and future prospects. J Card Surg 2010;25:484-9. [Crossref] [PubMed]
- Eichler HG, Kong SX, Gerth WC, et al. Use of cost-effectiveness analysis in health-care resource allocation decision-making: how are cost-effectiveness thresholds expected to emerge? Value Health 2004;7:518-28. [Crossref] [PubMed]
- Long EF, Swain GW, Mangi AA, et al. Comparative survival and cost-effectiveness of advanced therapies for end-stage heart failure. Circ Heart Fail 2014;7:470-8. [Crossref] [PubMed]
- Rogers JG, Bostic RR, Tong KB, et al. Cost-effectiveness analysis of continuous-flow left ventricular assist devices as destination therapy. Circ Heart Fail 2012;5:10-6. [Crossref] [PubMed]
- Pulikottil-Jacob R, Suri G, Connock M, et al. Comparative cost-effectiveness of the HeartWare versus HeartMate II left ventricular assist devices used in the United Kingdom National Health Service bridge-to-transplant program for patients with heart failure. J Heart Lung Transplant 2014;33:350-8. [Crossref] [PubMed]