Recent progress in radioactive seed implantation brachytherapy of non-small cell lung cancer: a narrative review
Introduction
Lung cancer is the malignancy with the highest mortality rate in the male population, and approximately 85% of lung cancer patients have non-small cell lung cancer (NSCLC) (1). For such patients, radiotherapy is usually used as an important alternative treatment method (2). External beam radiotherapy (EBRT), as a conventional radiation therapy, has been one of the optional therapies for the treatment of NSCLC. Although EBRT has some advantages, such as mature technology, and more convenient. However, due to the limitation of therapeutic mechanism, EBRT needs to pass the accelerated photon or electron beam through normal tissues before it can reach the tumour tissues. On the one hand, this causes additional damage to normal tissues; on the other hand, in order to achieve the therapeutic dose, EBRT needs to increase the irradiation dose, which in turn exacerbates the damage to the tissues in the irradiation path (3). However, brachytherapy has some advantages in the above problems. Since the irradiation comes from inside the tumour, the irradiation to the surrounding tissues can be effectively reduced. The efficacy of brachytherapy depends on the direct irradiation of tumours with high doses of radiation from a radiation source. Brachytherapy has a considerable dose-dependent advantage of radiation dose gradients over conventional external irradiation techniques. In fact, if the tumour moves during radiation, the radiation source also moves, because the radiation source is inside the tumour. This property differs from conventional EBRT, which requires an additional margin to account for setup and organ motion uncertainties (4-6). In this review, we describe a list based on the current literature and present promising clinical data regarding radioactive seed implantation brachytherapy (RSI-BT) in NSCLC treatment (Table 1). We present this article in accordance with the Narrative Review reporting checklist (available at https://jtd.amegroups.com/article/view/10.21037/jtd-23-1600/rc).
Table 1
Therapy | NSCLC stages | Year | Content | Outcome (positive & negative) |
---|---|---|---|---|
RSI-BT alone | Early-stage | 2020 | Safety and efficiency | 11 CR, 9 PR, 2 PD cases, OS of 11.7±7.6 months (7) |
3 CR, 1 PR and 1 PD cases (8) | ||||
Advanced | 2019 | Retrospective clinical study | Get better ORR, slight fever (9). Inhibit local tumour growth 4 cases of pneumothorax, 3 cases of hemoptysis, and 2 cases of particle displacement (10) | |
2020 | RSI-BT vs. microwave ablation | Longer OS and DFS (11) | ||
2021 | Malignant airway compression | Relieves compression, include radiation-related pulmonary reactions 13.33%; skin reactions 13.33%; radiation-related esophageal reactions 6.67%, and leukopenia 10% (12) | ||
2022 | Safety and efficiency | Better LCR, acute grade 1–2 toxicity was identified in 4 patients (13) | ||
2023 | A randomized trial, a phase 2 clinical trial | Safe and effective, distant disease, corresponding to 40% of patients and 65.2% of all failures. 2.7% patients developed grade 1 acute pneumonitis. Grade 2 and 3 acute esophagitis occurred in 14.7% and 5.3% patients, respectively (14,15) | ||
RSI-BT + EBRT | Advanced | 2018 | RSI-BT vs. EBRT | Higher quality of life scores, no significant differences were observed for complications (16) |
Early-stage | 2020 | RSI-BT vs. SART | Higher LCR and 1-year survival rate, the indicator of radioactive pneumonia V20 and V30 in Groups A and B was 6.06% and 4.207%, and 11.32% and 7.111%, respectively (17) | |
RSI-BT + chemotherapy | Advanced | 2020 | RSI-BT vs. second-line chemotherapy | Higher DCR, the incidence of hematologic toxicity in group A was significantly lower than that in group B (18) |
2021 | RSI-BT + BACE | Better LRR, no serious complications occurred in the two groups (19) | ||
2022 | Longer PFS and OS, the main adverse events included fever (100%), pain (65.2%), liver function impairment (65.2%), fatigue (56.5%), and nausea and vomiting (52.2%) (20) | |||
RSI-BT + targeted therapy | Advanced | 2021 | RSI-BT + EGFR-TKIs | Higher ORR, DCR and 2-year OS rate longer PFS (21) |
NSCLC, non-small cell lung cancer; RSI-BT, radioactive seed implantation brachytherapy; CR, complete response; PR, partial response; PD, progressive disease; OS, overall survival; ORR, objective response rate; DFS, disease-free survival; LCR, local control rate; EBRT, external beam radiotherapy; SART, stereotactic ablative radiotherapy; DCR, disease control rate; BACE, bronchial artery chemoembolization; LRR, local response rate; PFS, progression-free survival; EGFR, epidermal growth factor receptor; TKI, tyrosine kinase inhibitor.
Methods
The PubMed database was initially searched on March 02, 2023. Table 2 highlights the search conducted using the combination of the Medical Subject Headings (MeSH) terms: “Brachytherapy”, and “Lung Neoplasms”. And we integrated clinical studies including “radioactive seed implantation brachytherapy” to make it more accurate.
Table 2
Items | Specification |
---|---|
Date of search | 03/02/2023 to 03/05/2023 |
Databases searched | PubMed |
Search terms used | Search terms: “Brachytherapy [MeSH]” and “Lung Neoplasms [MeSH]”. Filters: English |
Timeframe | 2018 to 2023 |
Inclusion and exclusion criteria | Inclusion criteria: experimental design; discussed brachytherapy (mainly radioactive seed implantation brachytherapy) and its application in lung neoplasms (mainly non-small cell lung cancer) treatment; mentioned the effects of therapeutics on early-stage and advanced non-small cell lung cancer |
Exclusion criteria: literature reviews; case studies; only mentioned brachytherapy and lung neoplasms without description of specific therapeutic effects | |
Selection process | The selection process was conducted independently by the authors and was approved by a board-certified pulmonologist. The search produced 53 articles that were examined according to the inclusion and exclusion criteria; this reduced the total to 15 articles. Then, we narrowed the list to those that discussed the specific radioactive seed implantation brachytherapy |
Technical background
The mechanisms of RSI-BT for cancer lesions include direct irradiation to promote apoptosis of cancer cells and inhibition of vascular growth in cancerous tissues (22-24). DNA strands are the primary target of therapeutic irradiation, and radiation-induced DNA damage can lead directly to cell death, cell cycle redistribution and microenvironmental changes without reparations. The therapeutic index of radiation reflects the differential response between tumour and normal tissues because tumour cells have a lower capacity for DNA repair than normal tissue cells. The ideal technique should be able to deliver a therapeutic dose to the tumour while minimizing the dose to the organs at risk (25).
Iridium-192 (Ir-192), cobalt-60 (Co60), iodine-125 (I-125) and palladium-103 (Pd-103) are most commonly used in modern brachytherapy (26,27). I-125, whose efficacy and safety in RSI-BT have been established, is the principal particle utilized in clinical practice (4). Especially for elderly NSCLC patients who have lost the chance for surgery and are in poor physical condition, brachytherapy can control tumour progression and improve quality of life.
The total dose can be obtained by (I) continuous low-dose-rate (LDR) irradiation; (II) repeating low-intensity pulses hourly for several days (pulsed-dose-rate irradiation); or (III) delivering several portions of a high dose at a time [high-dose-rate (HDR) irradiation] (4,26). Radioactive seed implantation brachytherapy is commonly used in LDR brachytherapy (LDR-BT), while HDR brachytherapy (HDR-BT) and pulsed-dose-rate brachytherapy (PDR-BT) have shown advantages in the treatment of internal bronchial lesions (4). As an example, Ir-192, which is commonly used as a source for HDR-BT, typically delivers at a rate of ≥12 Gy/h, compared to 0.4–2.0 Gy/h of LDR-BT. The linear quadratic (LQ) equation can be used to calculate the total biologically effective dose (BED), which can be used to assess the frequency that HDR-BT/LDR-BT is received (28). At the same time, more precise treatment planning systems (TPSs) and the use of high-resolution computed tomography (CT) have made dose control easier.
The standard treatment planning procedure for 3D volumetric image-based brachytherapy involves three main steps: (I) anatomic contouring, (II) applicator digitization, and (III) dosimetric planning and optimization, using a dedicated TPS (29). Steps (I) and (II) would be accomplished by using TPS, but step (III) usually needs more BT teams’ effort and time. Accurate delineation of the clinical target volume (CTV) and organ at risk (OAR) is a crucial step in radiotherapy treatment planning (12,30). However, it is still a labor-intensive process to manually contour the CTV and OARs in a 3D image volume with some guidelines exist to help define the contours. Deep-learning-based image processing has demonstrated tremendous potential to help this (31). Additionally, dose calculations based on the AAPM Task Group 43 formalism are currently the mainstream (32). Some model-based calculation methods are increasingly being used to improve efficiency, such as Monte Carlo simulation or solving the Boltzmann transport equation (33).
In recent years, 3D noncoplanar templates, polylactic acid templates, and automatic needles have gradually been applied in clinical treatment (34-36). They enhance RSI-BT in numerous ways, including particle selection, needle insertion, template selection and design, making brachytherapy more precise and less expensive. A study found that particle implantation using a 3D noncoplanar template requires fewer probes, less rib injury, and a higher dose in the target organ region than a conventional 3D coplanar template to achieve the same therapeutic dose, making RSI-BT for NSCLC more individualized and precise (34). Additionally, polylactic acid-based templates can reduce the cost of 3D noncoplanar templates, making RSI-BT cheaper (35). Automated probes have effectively posed a challenge to conventional manual probes in terms of probe implantation, and investigations have revealed that the average misses in probe angle and depth are 2° and 1 mm, respectively, and the average segmentation time per needle is 0.238 s, which is already useful (36).
Clinical applications
RSI-BT alone for NSCLC
Early-stage NSCLC
The location of the tumour lesion, the tumour’s clinical stage, and other factors influence the specific strategy used for RSI-BT of NSCLC. RSI-BT can be effective under a variety of circumstances.
Zhao et al. demonstrated that CT-guided brachytherapy with I-125 implantation improved local control rates, decreased side effects, and improved patient quality of life. Of the 26 patients, 11 patients had complete response (CR) and 9 patients had partial response (PR), with an overall survival (OS) of 11.7±7.6 months (7). A study including six patients also demonstrated the safety and efficacy of CT-guided I-125 implantation for BT, with only one patient progressing in the 12-month postoperative follow-up (8). For patients with early-stage NSCLC who refuse to undergo surgery, the disease can be completely controlled or even eradicated by RSI-BT (Figure 1). However, RSI-BT was not linked to a lower risk of local or distant metastases or better outcomes in patients with clinical stage I NSCLC, according to a meta-analysis by Chen et al. (37). Regardless, it may reduce local recurrence (LR) when the BED is >100 Gy. In addition, a study comparing the effectiveness of brachytherapy and external beam therapy in 543 patients with limited resection for NSCLC found that while survival rates for patients with lung cancer with T1–T4N0M0 were comparable in the groups receiving RSI-BT and EBRT (compared to EBRT, brachytherapy was associated with a decreased risk of death for both OS and disease-specific survival (DSS) [hazard ratio (HR) 0.604; 95% confidence interval (CI): 0.380–0.961 and HR 0.524; 95% CI: 0.303–0.908, respectively), patients with T1 had higher survival rates after receiving brachytherapy (38). In conclusion, RSI-BT for early-stage NSCLC has been shown to be safe and therapeutically effective.
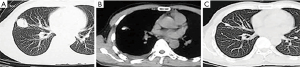
Advanced NSCLC
In advanced lung cancer, the therapeutic effect of RSI-BT is more limited and is more about relieving cancer symptoms and improving patients’ quality of life. The first topic covered is the function of CT-guided RSI-BT in the management of advanced NSCLC. I-125 RSI-BT alone significantly improved clinical outcomes and decreased the incidence of myelosuppression compared to chemotherapy, according to a meta-analysis by Zhang et al. (39). RSI-BT, however, might cause lung damage. To support the combined findings of complications, larger samples and higher quality randomized controlled studies are needed. The majority of studies on RSI-BT have demonstrated that the method is safe and reliable with controllable side effects and less harm to nearby organs while having the same or better local control rate, lower resource cost, and far lower overall radiation dose compared to conventional local treatment (10,13,24,40-45). However, the manageability of the difficulties associated with RSI-BT and issues with dose management have also been brought up by scholars in several studies (13,41,42). Moreover, advanced lung cancer-related airway constriction can be treated using bronchoscope-guided I-125 RSI-BT (Figure 2), and this therapy may also benefit patients with metastatic lung cancer (46,47).
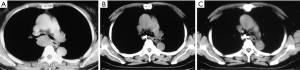
Radioactive stent implantation
A novel kind of radioactive particle stent implantation is being used in clinical practice in addition to the currently available BT procedure. A meta-analysis of radioactive and plain stent insertion for the treatment of malignant airway stenosis was performed by Meng et al. (48). It revealed that radioactive stent insertion was sufficient to reduce the rate of stent restenosis and extend the patient’s OS. New stents incorporating radioactive particles have also demonstrated therapeutic value in the treatment of inoperable malignant airway obstruction. Wang et al. discovered that from the second month after implantation, the group with radiographic stents had lower tracheal restenosis than the group with ordinary stents and a greater median survival (170 vs. 123 days, P<0.05). This study involved 66 patients with malignant airway obstruction (49). Moreover, radioactive stents can aid in increasing stent patency and OS and relieve superior vena cava stenosis caused by lung cancer (50). Although the safety has only been partially tested, a growing body of research points to the therapeutic potential of radioactive stents in the treatment of cavernous organs (51). However, additional reliable clinical trials are still needed.
Combining RSI-BT with other treatments
RSI-BT combined with EBRT
RSI-BT can be used not only alone in clinical treatment but also in combination with other existing therapies to improve treatment outcomes. One study found that brachytherapy can improve tissue radiosensitivity by increasing pO2 in the tumour microenvironment, providing evidence for the combination of RSI-brachytherapy and EBRT (52). Retrospective research by Li et al. further demonstrated the efficacy of repeated I-125 seed implantation in conjunction with EBRT in the management of LR and metastasis in stage III/IV NSCLC [the overall (complete + partial) response rate was 87.4%]. The local control rates after the first, second, and third years were 94.1%, 58.8% and 41.2%, respectively (53). Dual I-125 therapy is efficient in boosting the therapeutic dose to tumour lesions following EBRT failure. With an objective response rate (ORR) of 76%, 10 patients had CR, and 9 patients had PR after I-125 implantation in a retrospective study comprising 25 patients with HNSTS who underwent surgery and experienced recurrence after EBRT from 2006 to 2018 (54).
RSI-BT combined with chemotherapy
RSI-BT has been used in combination with chemotherapy in a relatively large number of studies. Addressing the mechanism behind their combination, a study by Rong et al. found that lobaplatin (LBP) inhibits the AKT/mTOR pathway, promoting the apoptotic and antiproliferative effects of I-125 in NSCLC cells (55). I-125 particle implantation in conjunction with chemotherapy can improve the short-term local control rate [risk ratio (RR) 1.34; 95% CI: 1.09–1.65%, P=0.005] and efficiency in early-stage lung cancer, but more clinical evidence is required (56). To control the progression of advanced lung cancer with less harmful side effects, which is crucial for elderly patients, RSI-BT can also be used in combination with second-line chemotherapeutic drugs, particularly after first-line chemotherapy fails (18,19,37,57). Moreover, bronchial artery embolization chemotherapy, a particular type of chemotherapy, may be used with RSI-BT. The combination can significantly slow tumour growth and enhance NSCLC prognosis (20,58-60). In a randomized controlled trial, patients who underwent bronchial artery chemoembolization (BACE) combined with I-125 RSI-BT achieved a better disease remission rate than those who underwent BACE alone (62.75% vs. 41.18%) (58). A pilot study, however, found no discernible difference in the effects of injectable medications used alone and in combination (61). Moreover, RSI-BT coupled with chemotherapy can ease severe airway obstruction caused by NSCLC. Jiang et al. effectively combined chemotherapy with the use of conventional transbronchial needle aspiration (C-TBNA) to implant radioactive particles into a bronchial lesion in a 51-year-old patient (62). Even though the majority of the currently used regimens of RSI-BT combined with chemotherapy have produced superior outcomes, further high-quality randomized controlled studies are still required to prove the combination therapy’s safety and effectiveness.
RSI-BT combined with targeted therapy
RSI-BT combined with targeted therapy has been applied in the treatment of NSCLC (63-66). According to Feng et al., overall effectiveness in the apatinib combined with RSI-BT group was higher than that in the control group, and no fatal consequences occurred, demonstrating the therapy’s safety and effectiveness (63). The elderly cases of combined apatinib and RSI-BT described by Zhang et al. can also be used to corroborate the findings of Feng et al. (64). In addition to clinical use, according to He et al. on A549 mice, RSI-BT combined with anlotinib prevented bone loss in tumour-bearing mice, but there was no significant difference between single and multiple particle implantations at the same total dose (65).
RSI-BT combined with immunotherapy
Clinical reports of immunotherapy combined with RSI-BT in recent years are less frequent, and some studies have focused more on the mechanistic aspect. A safety investigation of oncologic therapies, which included RSI-BT, revealed no unanticipated or unmanageable adverse effects when RSI-BT was combined with immune checkpoint inhibition therapy (67). The pembrolizumab and I-125 RSI-BT combination demonstrated promising outcomes in NSCLC, and PD-L1 can be employed as a prognostic biomarker, according to a study by Wang et al. (68). Furthermore, immunotherapy can alter the shape of radioactive particles that enter tumour cells, improving the effectiveness and precision of RSI-BT (69). Although the use of immunotherapy and RSI-BT in combination has shown some positive clinical results, further research on the underlying concepts is still needed. The delivery method for RSI-BT can even be changed entirely.
Limitations
There are still several side effects that limit RSI-BT in clinical treatment, such as radiation pneumonia, pneumothorax, and haemorrhage (70). The values of the incidence of haemorrhage, pneumothorax, and radiographic lung injury have been reported in the literature to be 0.14% (95% CI: 0.07–0.21%), 0.19% (95% CI: 0.11–0.28%), and 0.00% (95% CI: 0.00–0.03%), respectively (56). Meanwhile, RSI-BT can temporarily halt the progression of the disease. The lack of a unified standard industry practice and potential complications brought on by operator technical issues may impact the effectiveness of the treatment. According to research conducted in Belgium, there is a need for advancements in RSI-BT-related education, awareness, implementation, collaboration, and cooperation (71). Consequently, additional data support and standardized training of staff are still needed.
Conclusions
As technology advances, RSI-BT is an effective local oncologic intervention. It can be used alone or in conjunction with surgery, radiation, chemotherapy, immunotherapy, and targeted therapy to play a more complex role in clinical care. Its applications are promising, and RSI-BT plays a significant role in the treatment of digestive illnesses, prostate cancer, breast cancer, and lung cancer (72,73). To ensure treatment safety and efficacy, standardization of RSI-BT is needed. Meanwhile, multicentre, randomized clinical controlled trials are needed to validate the efficacy of minimally invasive interventions for tumours, including RSI-BT.
Acknowledgments
The authors thank Shanghai Municipal Hospital Respiratory and Critical Care Medicine Specialist Alliance.
Funding: This work was supported by
Footnote
Reporting Checklist: The authors have completed the Narrative Review reporting checklist. Available at https://jtd.amegroups.com/article/view/10.21037/jtd-23-1600/rc
Peer Review File: Available at https://jtd.amegroups.com/article/view/10.21037/jtd-23-1600/prf
Conflicts of Interest: All authors have completed the ICMJE uniform disclosure form (available at https://jtd.amegroups.com/article/view/10.21037/jtd-23-1600/coif). The authors have no conflicts of interest to declare.
Ethical Statement: The authors are accountable for all aspects of the work in ensuring that questions related to the accuracy or integrity of any part of the work are appropriately investigated and resolved.
Open Access Statement: This is an Open Access article distributed in accordance with the Creative Commons Attribution-NonCommercial-NoDerivs 4.0 International License (CC BY-NC-ND 4.0), which permits the non-commercial replication and distribution of the article with the strict proviso that no changes or edits are made and the original work is properly cited (including links to both the formal publication through the relevant DOI and the license). See: https://creativecommons.org/licenses/by-nc-nd/4.0/.
References
- Sung H, Ferlay J, Siegel RL, et al. Global Cancer Statistics 2020: GLOBOCAN Estimates of Incidence and Mortality Worldwide for 36 Cancers in 185 Countries. CA Cancer J Clin 2021;71:209-49. [Crossref] [PubMed]
- Araújo A, Barroso A, Parente B, et al. Unresectable stage III non-small cell lung cancer: Insights from a Portuguese expert panel. Pulmonology 2023;S2531-0437(22)00284-7.
- Tanderup K, Ménard C, Polgar C, et al. Advancements in brachytherapy. Adv Drug Deliv Rev 2017;109:15-25. [Crossref] [PubMed]
- Stewart A, Parashar B, Patel M, et al. American Brachytherapy Society consensus guidelines for thoracic brachytherapy for lung cancer. Brachytherapy 2016;15:1-11. [Crossref] [PubMed]
- Casutt A, Kinj R, Ozsahin EM, et al. Fiducial markers for stereotactic lung radiation therapy: review of the transthoracic, endovascular and endobronchial approaches. Eur Respir Rev 2022;31:210149. [Crossref] [PubMed]
- Costa R, Aires F, Rodrigues D, et al. Results of surgery versus stereotactic body radiotherapy for lung cancer. Pulmonology 2023;29:342-4. [Crossref] [PubMed]
- Zhao J, Zhi Z, Zhang H, et al. Efficacy and safety of CT-guided (125)I brachytherapy in elderly patients with non-small cell lung cancer. Oncol Lett 2020;20:183-92. [Crossref] [PubMed]
- Yang DY, Lin YP, Xue C, et al. CT-guided percutaneous implantation of (125)I particles in treatment of early lung cancer. J Thorac Dis 2020;12:5996-6009. [Crossref] [PubMed]
- Dai F, Wang J, An H, et al. Therapy of 125I particles implantation inhibited the local growth of advanced non-small cell lung cancer: a retrospective clinical study. Am J Transl Res 2019;11:3737-49.
- Kou F, Gao S, Liu S, et al. Preliminary clinical efficacy of iodine-125 seed implantation for the treatment of advanced malignant lung tumors. J Cancer Res Ther 2019;15:1567-73. [Crossref] [PubMed]
- Liu B, Wang Y, Tian S, et al. Salvage treatment of NSCLC recurrence after first-line chemotherapy failure: Iodine-125 seed brachytherapy or microwave ablation? Thorac Cancer 2020;11:697-703. [Crossref] [PubMed]
- Yoon SM, Suh R, Abtin F, et al. Outcomes with multi-disciplinary management of central lung tumors with CT-guided percutaneous high dose rate brachyablation. Radiat Oncol 2021;16:99. [Crossref] [PubMed]
- Xiang L, Ren PR, Li HX, et al. Effect of 3-Dimensional Interstitial High-Dose-Rate Brachytherapy With Regional Metastatic Lymph Node Intensity-Modulated Radiation Therapy in Locally Advanced Peripheral Non-Small Cell Lung Cancer: 5-Year Follow-up of a Phase 2 Clinical Trial. Int J Radiat Oncol Biol Phys 2023;115:347-55. [Crossref] [PubMed]
- Sur R, Pond G, Falkson C, et al. BRACHY: A Randomized Trial to Evaluate Symptom Improvement in Advanced Non-Small Cell Lung Cancer Treated With External Beam Radiation With or Without High-Dose-Rate Intraluminal Brachytherapy. Int J Radiat Oncol Biol Phys 2023;116:601-10. [Crossref] [PubMed]
- Xiang Z, Wang L, Yan H, et al. (125)I seed brachytherapy versus external beam radiation therapy for the palliation of painful bone metastases of lung cancer after one cycle of chemotherapy progression. Onco Targets Ther 2018;11:5183-93. [Crossref] [PubMed]
- Zhang Z, Mao H, Wang X, et al. Comparison of I(125) seed brachytherapy (radioactive seed brachytherapy) joint three-dimensional conformal radiotherapy and stereotactic ablative radiotherapy on early nonsmall cell lung cancer. J Cancer Res Ther 2020;16:1560-8. [Crossref] [PubMed]
- Wang H, Lu J, Zheng XT, et al. Oligorecurrence Non-small Cell Lung Cancer After Failure of First-Line Chemotherapy: Computed Tomography-Guided (125)I Seed Implantation vs. Second-Line Chemotherapy. Front Oncol 2020;10:470. [Crossref] [PubMed]
- Xiang Z, Zhong Z, Mu L, et al. The Clinical Value of Computed Tomography (CT)-Guided (125)I Brachytherapy for Locally Advanced Non-Small Cell Lung Cancer After Progression of Concurrent Radiochemotherapy. Cancer Manag Res 2021;13:5297-307. [Crossref] [PubMed]
- Zhao G, Liu S, Liu Y, et al. CalliSpheres(®) microsphere transarterial chemoembolization combined with (125)I brachytherapy for patients with non-small-cell lung cancer liver metastases. Front Oncol 2022;12:882061. [Crossref] [PubMed]
- Wang X, Wang D. Clinical analysis of 125I seed implantation combined with epidermal growth factor receptor-tyrosine kinase inhibitors in advanced non-small cell lung cancer. J BUON 2021;26:1879-86.
- Zhang T, Mo Z, Duan G, et al. (125)I Seed Promotes Apoptosis in Non-small Lung Cancer Cells via the p38 MAPK-MDM2-p53 Signaling Pathway. Front Oncol 2021;11:582511. [Crossref] [PubMed]
- Xiang GL, Zhu XH, Lin CZ, et al. 125I seed irradiation induces apoptosis and inhibits angiogenesis by decreasing HIF-1alpha and VEGF expression in lung carcinoma xenografts. Oncol Rep 2017;37:3075-83. [Crossref] [PubMed]
- Cheng J, Ma S, Yang G, et al. The Mechanism of Computed Tomography-Guided 125I Particle in Treating Lung Cancer. Med Sci Monit 2017;23:292-9. [Crossref] [PubMed]
- Chargari C, Magne N, Guy JB, et al. Optimize and refine therapeutic index in radiation therapy: Overview of a century. Cancer Treat Rev 2016;45:58-67. [Crossref] [PubMed]
- Chargari C, Deutsch E, Blanchard P, et al. Brachytherapy: An overview for clinicians. CA Cancer J Clin 2019;69:386-401. [Crossref] [PubMed]
- Parashar B, Port J, Arora S, et al. Analysis of stereotactic radiation vs. wedge resection vs. wedge resection plus Cesium-131 brachytherapy in early stage lung cancer. Brachytherapy 2015;14:648-54. [Crossref] [PubMed]
- Zaorsky NG, Horwitz EM. Chapter 44 - Brachytherapy for Prostate Cancer: An Overview. In: Mydlo JH, Godec CJ, editors. Prostate Cancer (Second Edition). San Diego: Academic Press; 2016:399-411.
- Mayadev J, Qi L, Lentz S, et al. Implant time and process efficiency for CT-guided high-dose-rate brachytherapy for cervical cancer. Brachytherapy 2014;13:233-9. [Crossref] [PubMed]
- Wu B, Ricchetti F, Sanguineti G, et al. Patient geometry-driven information retrieval for IMRT treatment plan quality control. Med Phys 2009;36:5497-505. [Crossref] [PubMed]
- Georg D, Hopfgartner J, Gòra J, et al. Dosimetric considerations to determine the optimal technique for localized prostate cancer among external photon, proton, or carbon-ion therapy and high-dose-rate or low-dose-rate brachytherapy. Int J Radiat Oncol Biol Phys 2014;88:715-22.
- LeCun Y, Bengio Y, Hinton G. Deep learning. Nature 2015;521:436-44. [Crossref] [PubMed]
- Rivard MJ, Coursey BM, DeWerd LA, et al. Update of AAPM Task Group No. 43 Report: A revised AAPM protocol for brachytherapy dose calculations. Med Phys 2004;31:633-74. [Crossref] [PubMed]
- Beaulieu L, Carlsson Tedgren A, Carrier JF, et al. Report of the Task Group 186 on model-based dose calculation methods in brachytherapy beyond the TG-43 formalism: current status and recommendations for clinical implementation. Med Phys 2012;39:6208-36. [Crossref] [PubMed]
- Ji Z, Sun H, Jiang Y, et al. Comparative study for CT-guided (125)I seed implantation assisted by 3D printing coplanar and non-coplanar template in peripheral lung cancer. J Contemp Brachytherapy 2019;11:169-73. [Crossref] [PubMed]
- Han X, Fang S, Sheng R, et al. Dosimetry verification of three-dimensional printed polylactic acid template-guided precision (125) I seed implantation for lung cancer using a desktop three-dimensional printer. J Appl Clin Med Phys 2021;22:202-9. [Crossref] [PubMed]
- Zheng Y, Jiang S, Yang Z, et al. Automatic needle detection using improved random sample consensus in CT image-guided lung interstitial brachytherapy. J Appl Clin Med Phys 2021;22:121-31. [Crossref] [PubMed]
- Chen ZK, Fan J, Li FQ, et al. I-125 seeds with chemotherapy for progressive non-small-cell lung cancer after first-line treatment: a meta-analysis. J Cardiothorac Surg 2022;17:75. [Crossref] [PubMed]
- Patel MA, Fazli Y, Sivakumar S, et al. Brachytherapy vs external beam therapy among NSCLC patients undergoing limited surgical resection. J Cancer Res Clin Oncol 2021;147:853-61. [Crossref] [PubMed]
- Zhang W, Li J, Li R, et al. Efficacy and safety of iodine-125 radioactive seeds brachytherapy for advanced non-small cell lung cancer-A meta-analysis. Brachytherapy 2018;17:439-48. [Crossref] [PubMed]
- Doggett SW, Chino S, Lempert T. A novel approach for salvage treatment of non-small-cell lung cancer: percutaneous CT fluoroscopy-guided permanent seed brachytherapy for salvage treatment of lung cancer: long-term results of a case series. J Contemp Brachytherapy 2019;11:174-9. [Crossref] [PubMed]
- Wang Y, Zhu L, Lin X, et al. Therapeutic Effect of CT-guided 125I Seed Implantation on Advanced Lung Cancer and Pulmonary Metastatic Carcinoma. Zhongguo Fei Ai Za Zhi 2020;23:424-8. [Crossref] [PubMed]
- Wang Z, Chen S, Su M, et al. Analysis of the clinical efficacy and safety of computerized tomography-guided 125 I seed implantation in the treatment of non-small cell lung cancer that relapsed after chemoradiotherapy. J Cancer Res Ther 2022;18:426-31. [Crossref] [PubMed]
- Chatzikonstantinou G, Zamboglou N, Baltas D, et al. Image-guided interstitial high-dose-rate brachytherapy for dose escalation in the radiotherapy treatment of locally advanced lung cancer: A single-institute experience. Brachytherapy 2019;18:829-34. [Crossref] [PubMed]
- Rashid A, Pinkawa M, Haddad H, et al. Interstitial single fraction brachytherapy for malignant pulmonary tumours. Strahlenther Onkol 2021;197:416-22. [Crossref] [PubMed]
- Ji Z, Jiang Y, Guo F, et al. Radiation-related Adverse Effects of CT-guided Implantation of (125)I Seeds for Thoracic Recurrent and/or Metastatic Malignancy. Sci Rep 2019;9:14803. [Crossref] [PubMed]
- Ji Z, Jiang Y, Guo F, et al. Safety and efficacy of CT-guided radioactive iodine-125 seed implantation assisted by a 3D printing template for the treatment of thoracic malignancies. J Cancer Res Clin Oncol 2020;146:229-36. [Crossref] [PubMed]
- Zhao Z, Yao W, Zhang T, et al. Computed tomography-guided implantation of (125)I radioactive seeds in patients with malignant airway compression induced by advanced lung cancer: effectiveness and safety in 40 patients. J Contemp Brachytherapy 2020;12:343-50. [Crossref] [PubMed]
- Meng QK, Yu XY, Li W, et al. Radioactive and normal stent insertion for the treatment of malignant airway stenosis: A meta-analysis. Brachytherapy 2021;20:883-91. [Crossref] [PubMed]
- Wang Y, Lu J, Guo JH, et al. A Novel Tracheobronchial Stent Loaded with (125)I Seeds in Patients with Malignant Airway Obstruction Compared to a Conventional Stent: A Prospective Randomized Controlled Study. EBioMedicine 2018;33:269-75. [Crossref] [PubMed]
- Yuan FK, Fu YF, Shi YB, et al. Radioactive Seed Strand Efficacy in Superior Vena Cava Stenting Due to Non-small-cell Lung Cancer Obstruction. Vasc Endovascular Surg 2022;56:173-9. [Crossref] [PubMed]
- Zhu HD, Guo JH, Mao AW, et al. Conventional stents versus stents loaded with (125)iodine seeds for the treatment of unresectable oesophageal cancer: a multicentre, randomised phase 3 trial. Lancet Oncol 2014;15:612-9. [Crossref] [PubMed]
- Cron GO, Beghein N, Crokart N, et al. Changes in the tumor microenvironment during low-dose-rate permanent seed implantation iodine-125 brachytherapy. Int J Radiat Oncol Biol Phys 2005;63:1245-51. [Crossref] [PubMed]
- Li F, Wang L, Zhang Y, et al. A Retrospective Study on Using a Novel Single Needle Cone Puncture Approach for the Iodine-125 Seed Brachytherapy in Treating Patients With Thoracic Malignancy. Front Oncol 2021;11:640131. [Crossref] [PubMed]
- Chen Y, Jiang Y, Ji Z, et al. Efficacy and safety of CT-guided (125)I seed implantation as a salvage treatment for locally recurrent head and neck soft tissue sarcoma after surgery and external beam radiotherapy: A 12-year study at a single institution. Brachytherapy 2020;19:81-9. [Crossref] [PubMed]
- Rong JH, Li D, Li YL. Lobaplatin Enhances Radioactive (125)I Seed-Induced Apoptosis and Anti-Proliferative Effect in Non-Small Cell Lung Cancer by Suppressing the AKT/mTOR Pathway. Onco Targets Ther 2021;14:289-300. [Crossref] [PubMed]
- Chen E, Wang J, Zhang H, et al. Analysis of the efficacy and safety of iodine-125 seeds implantation in the treatment of patients with inoperable early-stage non-small cell lung cancer. J Contemp Brachytherapy 2021;13:347-57. [Crossref] [PubMed]
- Yue TH, Xing W. (125)I Seed Brachytherapy Combined with Single-Agent Chemotherapy in the Treatment of Non-Small-Cell Lung Cancer in the Elderly: A Valuable Solution. Onco Targets Ther 2020;13:10581-91. [Crossref] [PubMed]
- Xie P, He L, Zhang Y. Analysis on the Efficacy of Bronchial Artery Chemoembolization Combined with 125I Seed Implantation in the Therapy of Advanced Non-Small-Cell Lung Cancer Based on the Medical Database. Biomed Res Int 2022;2022:7376844. [Crossref] [PubMed]
- Xie P, Zhang Y, He L. Prognostic Evaluation of CT Imaging Big Data-Assisted Arterial Chemoembolization Combined with (125)I Seed Implantation for Non-Small-Cell Lung Cancer. Comput Math Methods Med 2022;2022:3472982. [Crossref] [PubMed]
- Chen C, Wang W, Yu Z, et al. Combination of computed tomography-guided iodine-125 brachytherapy and bronchial arterial chemoembolization for locally advanced stage III non-small cell lung cancer after failure of concurrent chemoradiotherapy. Lung Cancer 2020;146:290-6. [Crossref] [PubMed]
- Zhao Z, Tu J, Fan X, et al. Drug-eluting beads-transcatheter arterial chemoembolization with or without iodine-125 treatment is effective and tolerable in treating advanced non-small cell lung cancer patients: a pilot study. Transl Cancer Res 2020;9:3191-202. [Crossref] [PubMed]
- Jiang AG, Lu HY, Ding ZQ. Implantation of (125)I radioactive seeds via c-TBNA combined with chemotherapy in an advanced non-small-cell lung carcinoma patient. BMC Pulm Med 2019;19:205. [Crossref] [PubMed]
- Feng Y, Yang B, Li X. Clinical analysis of 125I seed implantation combined with Apatinib in the treatment of locally advanced lung cancer: A case series. J Pak Med Assoc 2021;71:1025-7. [Crossref] [PubMed]
- Zhang Y, Yin S, Jia Y, et al. Safety and efficacy of apatinib combined with iodine-125 in chemotherapy-refractory advanced lung cancer: A case report. Medicine (Baltimore) 2020;99:e21600. [Crossref] [PubMed]
- He F, Bao Q, Bai J, et al. Effects of I-125 seeds combined with anlotinib on tumor growth and bone metabolism in A549 tumor-bearing mice. Int J Radiat Biol 2021;97:1578-88. [Crossref] [PubMed]
- Xiang L, Zhang JW, Lin S, et al. Computed Tomography-Guided Interstitial High-Dose-Rate Brachytherapy in Combination With Regional Positive Lymph Node Intensity-Modulated Radiation Therapy in Locally Advanced Peripheral Non-Small Cell Lung Cancer: A Phase 1 Clinical Trial. Int J Radiat Oncol Biol Phys 2015;92:1027-34. [Crossref] [PubMed]
- Leppelmann KS, Mooradian MJ, Ganguli S, et al. Thermal Ablation, Embolization, and Selective Internal Radiation Therapy Combined with Checkpoint Inhibitor Cancer Immunotherapy: Safety Analysis. J Vasc Interv Radiol 2021;32:187-95. [Crossref] [PubMed]
- Wang S, Zhang J, Meng FJ, et al. Combination of pembrolizumab and (125)I attenuates the aggressiveness of non-small cell lung cancer. Oncol Lett 2020;19:4142-50. [Crossref] [PubMed]
- Kelly G, Milligan JJ, Mastria EM, et al. Intratumoral delivery of brachytherapy and immunotherapy by a thermally triggered polypeptide depot. J Control Release 2022;343:267-76. [Crossref] [PubMed]
- Zhang F, Wang J, Guo J, et al. Chinese expert consensus workshop report: Guideline for permanent iodine-125 seeds implantation of primary and metastatic lung tumors (2020 edition). J Cancer Res Ther 2020;16:1549-54. [Crossref] [PubMed]
- Salembier C, De Hertogh O, Daisne JF, et al. Brachytherapy in Belgium in 2018. A national survey of the brachytherapy study group of the Belgian SocieTy for Radiotherapy and Oncology (BeSTRO). Radiother Oncol 2020;150:245-52. [Crossref] [PubMed]
- Terheyden MM, Melchert C, Kovács G. External beam boost versus interstitial high-dose-rate brachytherapy boost in the adjuvant radiotherapy following breast-conserving therapy in early-stage breast cancer: a dosimetric comparison. J Contemp Brachytherapy 2016;8:294-300. [Crossref] [PubMed]
- Otake S, Goto T. Stereotactic Radiotherapy for Oligometastasis. Cancers (Basel) 2019;11:133. [Crossref] [PubMed]