Effects of no mask, a surgical mask and a fabric buff on peripheral oxygenation saturation during moderate intensity exercise: a randomised cross-over study
Highlight box
Key findings
• Wearing a mask caused a mild but sustained reduction in peripheral oxygen saturation (SpO2) at the commencement of exercise, which did not worsen throughout short (<30 min) moderate intensity exercise.
• In line with reductions in SpO2, perception of breathlessness was higher while wearing a mask, but rating of perceived exertion remained unchanged.
What is known and what is new?
• Currently unclear how masks affect SpO2 and other cardiorespiratory physiological measures.
• It is reasonable for healthy people to wear masks while engaging in 30-min moderate intensity exercise, as well as during other mild activities of daily living, including commuting, household chores and shopping.
What is the implication, and what should change now?
• Fear of participating in moderate intensity exercise with a mask seems unwarranted in healthy populations, but further research is required to confirm our findings.
• Future studies should aim to recruit children/adolescents and clinical populations.
Introduction
Background
Even prior to coronavirus disease 2019 (COVID-19), the ‘severe acute respiratory syndrome’ virus, which occurred in Asia almost 20 years earlier, led to a ‘mask culture’ in many Asian countries, where wearing face masks in public was considered normal (1). Since the emergence of the novel COVID-19, many countries and jurisdictions implemented wearing face masks in public to control the spread of COVID-19. Indeed face masks effectively reduced the spread of COVID-19 (2). Importantly, even after a person received vaccination, face masks were considered a cost-effective non-pharmaceutical strategy to contain the spread of COVID-19 (3). Unsurprisingly, many Asian countries achieved high compliance rates with wearing face masks in public and continued to do so even after the pandemic subsided (4).
Rationale and knowledge gap
At the height of the COVID-19 pandemic, countries and jurisdictions utilised ‘lockdowns’, where people could only leave home for certain reasons, in an attempt to stymie the spread of COVID-19 (5). One of the reasons a person could leave home was physical exercise, an important tool to maintain both metabolic and mental health (6). Yet, due to the respiratory transmission of COVID-19 and the increased ventilation during exercise (7), there were concerns that asymptomatic COVID-19 infected people may risk transmitting COVID-19 during exercise, as increased ventilation would produce a greater aerosol effect. Therefore, even with outdoor physical exercise, it was initially recommended that face masks should be worn (8). Indeed, survey data from the United States indicates that face mask usage during competitive sports reduced COVID-19 transmission.
Yet, there have been lingering concerns surrounding the potential risks of reduced peripheral oxygen saturation (SpO2) and dyspnoea due to prolonged wearing of face masks (9). Wearing a face mask during normal daily activities did not cause hypoxemia (10), but it remains unclear whether face masks cause hypoxemia and/or dyspnoea during exercise. Some studies showed significant reductions in SpO2 during moderate intensity exercise (11,12), while others showed no effect of mask-wearing on SpO2 levels (13,14). Unfortunately, these study limitations included small samples, short exercise duration, or using frontline medical staff masks, such as the N95. Indeed, while ‘medical-grade’ masks, such as the N95, were initially popular, the use of a tubular fabric neck gaiter mask (buff mask; BM), which could be washed, gained in popularity due to a combination of cost-effectiveness, reduction in pollution, and ensuring the supply of medical grade masks for frontline workers (15).
Objective
The primary aim of this study was to determine whether wearing either a medical grade surgical mask (SM) or a tubular fabric neck gaiter (BM) resulted in reductions in SpO2 throughout moderate intensity exercise, also implying activities of daily living. The secondary aims of this study were to examine whether masks changed physiological measures, including end-tidal carbon dioxide (EtCO2), respiratory rate (RR), heart rate (HR), and expired breath temperature (EBT). Subjective measurements included ratings of perceived exertion (RPE), ratings of perceived breathlessness (RPB), and symptomology. We hypothesised that SpO2 will be impaired by wearing a SM and a BM during moderate intensity exercise as opposed to not wearing a mask. We present this article in accordance with the CONSORT reporting checklist for randomised crossover RCTS (available at https://jtd.amegroups.com/article/view/10.21037/jtd-23-1178/rc).
Methods
Participants and demographics
Adults aged 30–45 years who regularly performed exercise (at least three times a week for more than 30 min for at least the previous six weeks) were invited to participate. Participants were recruited through public advertisement (19 March–11 April 2021). Personal, local running clubs and gyms’ social media platforms were used. Exclusion from participation included chronic respiratory conditions (e.g., asthma, chronic obstructive pulmonary disease), known cardiovascular problems (e.g., congestive heart failure, atrial fibrillation), known rheumatological conditions that may be exacerbated by exercise (e.g., osteoarthritis, rheumatoid arthritis), present musculoskeletal injuries, any medications that may affect respiration (e.g., opioids), or any current acute illness, or COVID-19 infection within the last 3 months. The study was conducted in accordance with the Declaration of Helsinki (as revised in 2013). The study was approved by the Health Sciences Ethics Committee at the University of Pretoria (REC577/2020) and informed consent was obtained from all individual participants. The informed consent document included information on possible harms or unintended effects, e.g., fainting during exercise with a mask.
Measurements
All participants consented and completed a medical and training history document during the first visit and before the PRE-test exercise. During each visit, weight (kg; Tanita BF-350 Body Composition Analyzer, Tokyo, Japan) and height (cm; SECA 213 portable stadiometer, Hamburg, Germany) measurements were collected for each participant. Additionally, cycle saddle height (cm), hip height (cm), saddle position (seat orientation) and handlebar height (cm) were also recorded for each participant.
Three conditions were measured: (I) no mask (NM), which was the control condition; (II) SM, which is a 3-ply blue disposable SM commonly used by healthcare workers; and (III) BM, which is a tubular fabric neck gaiter consisting 95% Polyester 5% Spandex commonly used in the public due to the ability to wash and reuse. Each condition was measured at three separate testing sessions scheduled over a 6-day period with a minimum of 24 h between each test to allow sufficient recovery. During each testing session, physiological measures (SpO2, EtCO2, RR, HR, EBT) were taken at resting baseline (T0), 9 min (T1), 18 min (T2), and 27 min (T3). Participants were fitted with an oral/nasal airflow pressure cannula (ThermiSense®, SalterLabs, IL, USA) placed inside the mask. The cannular was linked to a portable capnograph and pulse oximeter metabolic analyser (LifeSense® II, Nonin Medical, Inc., Plymouth, MN, USA), which measured EtCO2 (in mmHg) and RR (in breaths per min). SpO2 was measured by finger pulse oximetry (LifeSense®). HR (in beats per min) was measured by a Polar® Team Pro sensor and chest strap (Polar Electro Oy, Kempele, Finland) for continuous live time recording.
Ambient temperature and EBT inside the mask were measured using a Fluke® 189 multi-meter with a Type-K integrated temperature probe (Fluke Process Instruments, Berlin, Germany). Subjectively, RPE was measured using the Borg Scale (6–20 rating scale) (16), while dyspnoea was measured using the RPB (a modified Borg 0–10 scale). Directly after the 30-min test, participants were asked to complete a 7-item symptomology questionnaire (0–10 Likert scale) on their subjective experience of wearing the mask during exercise.
Data collection and procedures
The study was a prospective, partially randomised, cross-over design. This type of design yields more efficient comparisons of different conditions, and fewer participants are needed to acquire the same level of statistical precision than a parallel design. While the participants and research staff helping to collect data were aware of the mask wearing conditions, the statistician undertaking the analysis was blinded to conditions. The first author generated the random allocation sequence, and the research coordinator assigned participants to the sequence of mask conditions. Each participant drew the type of mask condition from a concealed container for the first two sessions. For the third session, each participant needed to complete the mask condition not covered in the other two sessions.
Testing occurred within a specialised exercise physiology laboratory (Sports, Exercise Medicine, and Lifestyle Institute, University of Pretoria, South Africa). Appropriate COVID-19 protocols and infection control guidelines were implemented. Only three participants were tested in a time slot to ensure adequate social distancing. All investigators wore masks during the full sessions and sanitiser was routinely available. Cycle ergometers were cleaned and disinfected, and the test laboratory aired in-between tests.
For the PRE-test from 12–15 April 2021 (week 1), all participants were tested to determine maximal min power (MMP) during cycling on a WATTBike PRO (Wattbike Indoor cycle ergometer, Wattbike Ltd, Nottingham, UK). A 10-min cycling warm-up was subdivided into 5-min easy cycling, starting at a low intensity and gradually building towards three maximal short effort sprints [3 × (hard pedal 10 s, 50 s recovery ride)]. This session ended with 2 min of easy cycling to complete the warm-up. The warm-up period was also used to familiarise the participants with the resistance settings on the bike as well as to ensure their bike set-up was optimal for each individual. After the warm-up and a 5-min rest period, a 3-min maximal effort bout was conducted at a resistance the participant felt comfortable with. MMP can be derived from the maximal values achieved in the 3-min high-intensity bout. The peak power output (Watt) was recorded. The test intensity was determined using 60% of the individual’s peak power recorded in the PRE-test. The 60% intensity was chosen as the practitioners felt this intensity could be sustained over the 30-min period for novice participants. Following the 3-min maximal test, participants were allowed to perform a 10-min cool down to further familiarise themselves with maintaining a steady average power and cadence over a prolonged session.
During NM, SM, and BM tests from 3–8 May 2021 (week 2), all participants were scheduled for three separate exercise sessions under the different mask criteria. Participants were randomly assigned to each condition, with the testing order randomised. The exercise intervention for each mask condition was similar.
Resting measures (T0) were established by having participants sit quietly for 5 min during T0. After the baseline measure, there was a 10-min gradual warm-up, building toward the required power output (60%) for the session. A comfortable cadence for the individual participant that corresponded to their 60% power output was identified and recorded during the warm-up phase. Participants were instructed to ride at the identified cadence of ±2 rpm and maintain their 60% power output for 30 min. The data was collected at pre-determined time points [at resting baseline (T0), 9 min (T1), 18 min (T2), and 27 min (T3)]. Each test session ended with a 5-min cool-down period. The mean ambient temperature was kept at 20.8±2.5 ℃ throughout all exercise sessions.
Data and statistical analysis
A power analysis (G*Power 3.1.9.2) utilising the effect sizes of analysis of variance (ANOVA) from previous studies (17,18), at an α of 5%, and power of 80%, indicated 24 participants would be required per condition.
Statistical analysis was performed in R (R Foundation, Vienna, Austria) (19). All data were assessed for normality using the Shapiro Wilk test. The repeated measures ANOVA test was used for normally distributed data, followed by the Bonferroni adjusted post hoc analysis if differences were detected. For non-normally distributed data, differences between conditions were assessed by using the Friedman’s Test, followed by a Nemenyi post-hoc analysis if differences were detected. For normally distributed variables, the independent t-test was used, and for those that were not normally distributed, the non-parametric Mann-Whitney U test. The mask symptomology investigations were performed using the Wilcoxon signed-rank tests. All significance tests were performed at a 5% level of significance (P<0.05).
Results
Participants
All participants (n=30) were included at each stage. Table 1 presents the demographic characteristics of all study participants. Thirty participants [13 female (43.3%); 17 male (56.7%)] consented to participate (mean age 35.83±4.70 years, body mass index (BMI) 24.80±3.41 kg/m2, mean maximal power 242.19±85.53 Watt). All participants engaged in aerobic exercise, including running, cycling, and swimming, at least three times a week (average 324.50±244.14 min of training per week). Table S1 presents the details of the sports that participants listed. Table 2 presents data for all measures of the NM, SM, and BM conditions across all stages of exercise.
Table 1
Characteristics | All participants | Female | Male | P value |
---|---|---|---|---|
Sex | 30 (100.0) | 13 (43.3) | 17 (56.7) | |
Age (years) | 35.83±4.70 | 35.69±4.99 | 35.94±4.62 | 0.8169 |
Height (cm) | 168.99±32.51 | 168.07±4.27 | 180.24±5.36 | <0.0001* |
Weight (kg) | 76.56±15.28 | 63.00±6.24 | 86.93±11.42 | <0.0001* |
BMI (kg/m2) | 24.80±3.41 | 22.31±2.14 | 26.71±2.96 | <0.0001* |
Mean maximal power (Watt) | 242.19±85.53 | 193.85±49.75 | 293.29±62.16 | 0.0003* |
Average training (min/week) | 324.50±244.14 | 308.85±247.02 | 336.47±248.84 | 0.5712 |
Data are presented as n (%) or mean ± standard deviation. *, significant difference between female and male. BMI, body mass index; Watt, peak power output in 3-minute high-intensity session.
Table 2
Mask group | Physiological measure | T0 | T1 | T2 | T3 |
---|---|---|---|---|---|
No mask (n=30) | SpO2 (%) | 93.7±3.7x | 93.1±1.9 | 93.2±1.7 | 93.1±1.9 |
EtCO2 (mmHg) | 28.0±5.5 | 26.0±5.3* | 24.8±5.3* | 24.0±5.5*+ | |
RR (breaths/min) | 26.6±5.9 | 30.3±5.8* | 30.2±6.3* | 29.1±6.8 | |
HR (beats/min) | 76.0±13.5 | 148.4±12.6* | 152.8±13.4*+ | 155.4±12.9*+# | |
EBT (℃) | 17.2±0.8 | 17.5±0.9 | 17.4±1.0 | 17.5 ±1.0* | |
RPE (/20) | – | 10.3±2.0 | 11.5±2.4+ | 12.5±2.4+# | |
RPB (/10) | 0.8±0.9 | 2.0±1.2* | 2.5±1.2*+ | 2.9±1.6*+# | |
Surgical mask (n=30) | SpO2 (%) | 95.4±1.9xx | 93.3±2.1* | 93.0±2.0* | 93.1±1.8* |
EtCO2 (mmHg) | 31.6±5.8‡ | 28.9±6.1*‡ | 26.8±6.2*+‡ | 25.8±5.9*+‡ | |
RR (breaths/min) | 24.9±6.4 | 32.2±7.0* | 32.9±7.4* | 32.9±7.2* | |
HR (beats/min) | 79.1±14.4 | 148.0±12.8* | 151.5±14.4*+ | 154.6±16.2*+# | |
EBT (℃) | 24.0±2.3‡ | 28.1±2.2*‡ | 28.6±1.8*+‡ | 28.7±1.9*+‡ | |
RPE (/20) | – | 10.6±2.6x | 12.0±1.9+ | 13.1±1.9+# | |
RPB (/10) | 0.9±0.8 | 2.5±1.0*‡ | 3.3±1.1*+‡ | 4.2±1.4*+#‡ | |
Buff mask (n=30) | SpO2 (%) | 94.3±3.3x | 92.7±2.2*‡ | 92.6±2.2*‡ | 92.4±2.4* |
EtCO2 (mmHg) | 31.8±5.9‡ | 29.7±6.3*‡ | 27.7±5.9*+‡ | 26.9±5.4*+‡ | |
RR (breaths/min) | 27.0±5.7 | 32.3±5.8* | 33.3±4.8* | 33.0±5.2*+‡ | |
HR (beats/min) | 82.3±14.1 | 147.5±16.2* | 152.3±17.7*+ | 154.1±22.9*+# | |
EBT (℃) | 23.9±3.7‡ | 28.8±2.2*‡ | 28.6±2.3*‡ | 29.3±2.8*‡ | |
RPE (/20) | – | 10.7±2.0 | 12.2±1.9+ | 12.8±3.1+# | |
RPB (/10) | 1.6±1.7‡ | 2.9±1.0*‡ | 3.8±1.2*+‡ | 4.7±1.6 x*+#‡ |
Data are presented as mean ± standard deviation. x, 1 missing value; xx, 2 missing values. Within mask, between exercise timing analysis: *, significantly different from baseline (T0) (P<0.05); +, significantly different from T1 (P<0.05); #, significantly different from T2 (P<0.05). Between mask, within exercise timing analysis: ‡, significantly different from no mask group (P<0.05). Exercise timing analysis: resting baseline (T0), 9 min (T1), 18 min (T2), and 27 min (T3). SpO2, peripheral oxygenation saturation; EtCO2, end-tidal carbon dioxide; RR, respiratory rate; HR, heart rate; EBT, expired breath temperature; RPE, ratings of perceived exertion; RPB, ratings of perceived breathlessness.
Effect of masks on SpO2 throughout exercise
For the NM, SM and BM conditions, no changes in SpO2 were detected between the exercise stages (NM P=0.4, SM P=0.2, BM P=0.5, respectively) (Table S2). Comparing baseline (T0) with the exercise stages the NM showed no significant difference in SpO2 (P=0.096, P=0.149, P=0.156). The pairwise comparison for SM showed that T1, T2, and T3 were significantly lower than T0 (all P<0.001). The pairwise comparison for BM showed significant reductions at T1 (P=0.002), T2 (P=0.004), and T3 (P=0.002) when compared to T0 (Figure 1A, Table S3).
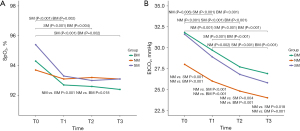
For the between mask conditions, Friedman’s test indicated no significant difference for SpO2 at T0 (P=0.135). For T1, the Friedman’s test shows a significant difference in SpO2 (P=0.026), with the pairwise analysis indicating a significantly lower SpO2 for the BM compared to NM (P<0.001), but no significant differences between SM and NM (P=0.964) and between SM and BM (P=0.072). For T2, the Friedman’s test indicated a significant difference in SpO2 (P=0.025), with the pairwise analysis showing significantly lower SpO2 between the BM condition compared to NM (P=0.018), but no significant differences between SM and NM (P=0.476) and between SM and BM (P=0.268). At T3, no significant differences in SpO2 were detected between the conditions (P=0.107) (Table S4).
Effect of masks on EtCO2 throughout exercise
As is expected for moderate intensity exercise, significant changes in EtCO2 occurred throughout exercise stages for all conditions (all P<0.001) (Table S4). For the NM condition, when compared to T0, significant reductions in EtCO2 were seen at T1 (P=0.006), T2 and T3 (both P<0.001) (Table S3). EtCO2 at T3 was significantly lower than T1 (P=0.002). There were no significant changes between T1 and T2 (P=0.097) and between T2 and T3 (P=0.4) (Table S2).
For the SM condition, significant reductions in EtCO2 were shown at T1, T2, and T3 (all P<0.001). EtCO2 was significantly higher at T1 compared to T2 (P=0.001) and T3 (P<0.001), while no significant difference was shown between exercise timing T2 and T3 (P=0.268). For the BM condition, significant reductions in EtCO2 were shown at T1, T2, and T3 (all P<0.001). EtCO2 was significantly lower at T2 and T3 compared to T1 (both P<0.001), while no significant difference was shown between T2 and T3 (P=0.13) (Figure 1B, Table S2).
Significant differences in EtCO2 were shown between mask conditions at all stages (T1 and T2: P<0.001; T3: P=0.0012). Pairwise comparison at T0 and T1 demonstrated significantly lower EtCO2 for the SM and BM (P<0.001) compared to NM, but no difference between SM and BM (T0: P=0.990, T1: P=0.921). At T2, pairwise comparison demonstrated significantly lower EtCO2 for the SM (P=0.004) and BM (P<0.001) compared to the NM, but no difference between SM and BM conditions (P=0.638). At T3, pairwise comparisons demonstrated significantly lower EtCO2 for the SM (P=0.018) and BM (P=0.001) compared to NM, but no difference between SM and BM conditions (P=0.719) (Table S4).
Effect of masks on respiratory rate, HR, expiratory breath temperature
As expected for moderate intensity exercise, RR was significantly higher during exercise compared to T0 (T0 vs. T1: P=0.001, T0 vs. T2: P=0.003) except in the NM condition when comparing T3 to T0 (P=0.088) (Table S3). The Friedman’s test showed no differences in RR between mask conditions at T0 (P=0.158) or T1 (P=0.273). At T2, the Friedman’s test indicated significant differences between the conditions (P=0.045). However, the pairwise comparisons did not identify any significant pairwise differences, but rather a trend toward a higher RR in the SM compared to the NM (P=0.053), but no difference between SM and BM (P=0.921) and BM and NM (P=0.128). This statistical anomaly between the Friedman’s test and the pairwise comparisons is rare and likely due to the reduction in power when undertaking the pairwise comparison. At T3, the Friedman’s test indicated significant differences between the conditions (P=0.016). There was a difference in RR between the conditions with a significantly higher RR in the BM compared to NM (P=0.013), but no differences between both SM and BM (P=0.556) and SM and NM (P=0.167) (Figure 2A, Table S4).
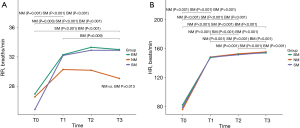
Similarly, as expected for moderate intensity exercise, HR was significantly higher during all exercise stages for all mask conditions (Friedman’s P<0.001) (Table S2). Importantly, no significant differences in HR were detected between the mask conditions at any exercise stage (T0: P=0.102, T1: P=0.230, T2: P=0.226, T3: P=0.182) (Figure 2B, Table S4).
Comparing the mask conditions at different exercise stages showed significant differences (all P<0.001) for EBT. For the pairwise comparisons at T0, T1, T2 and T3, EBT was significantly higher for SM compared to NM and BM compared to NM (all P<0.001) but not for SM compared to BM (T0: P=0.83, T1: P=0.63, T2: P=0.92, T3: P=0.53) (Figure 3, Table S4). Throughout all exercise stages there was no significant difference (NM: P=0.218, SM: P=0.073, BM: P=0.418) in EBT for any of the mask conditions (Table S2). The pairwise comparisons showed NM had a significantly higher EBT at T3 compared to T0 (P=0.029) but not at T2 and T1 compared to T0 (P=0.127 and P=0.105). The SM and BM had significant differences T1, T2 and T3 compared to T0 (all P<0.001) (Table S3). A post-priori analysis examining differences in EBT after adjusting for ambient temperature (EBT – ambient temperature) showed the same results. The NM condition did not experience any significant changes (P=0.08). For the BM condition, the Friedman’s test indicated significant differences in EBT (P<0.001).
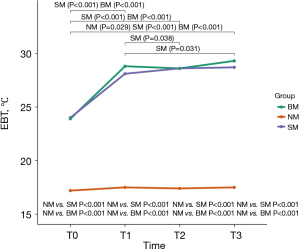
Effects of masks on RPE and RPB
As expected, Friedman’s tests indicated RPE significantly increased throughout exercise for all mask conditions (all P<0.001). The pairwise analysis showed RPE significantly increased for all exercise stages for all mask conditions (NM all P<0.001; SM all P<0.006; BM all P<0.004) (Table S2). The Friedman’s test showed no significant differences in RPE at T1 or T2 (P=0.180, P=0.339) (Table S4). At T3, the Friedman’s test indicated a significant difference (P=0.02) comparing the three masks. However, there was no difference between BM and NM (P=0.097), SM and NM (P=0.072), or between the SM and BM (P=0.991) (Figure 4A).
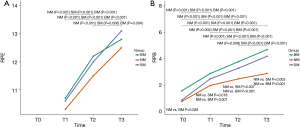
RPB also significantly increased for each condition at each time point, with the Friedman’s test indicating significant differences (P<0.001). The pairwise analysis showed RPB significantly increased from T0 for all stages of exercise for all mask conditions (NM P<0.008; SM P<0.001; BM P<0.001) (Table S2). There were significant differences in RPB between mask conditions. At rest, the Friedman’s test indicated significant differences in RPB (P=0.005), with the pairwise comparison indicating the BM had a significantly higher RPB compared to the NM (P=0.029). There was no difference between SM and NM (P=0.918). At T1, the Friedman’s test indicated significant differences in RPB (P<0.001). The pairwise comparison indicated the NM condition was significantly lower than both SM (P=0.018) and BM (P<0.001), while there was no difference between the SM and BM (P=0.365). At T2, the Friedman’s test indicated significant differences in RPB (P<0.001), with the pairwise comparison indicating the NM was significantly lower than both SM (P=0.007) and BM (P<0.001), while there was no difference between SM and BM (P=0.545). At T3, the Friedman’s test indicated significant differences in RPB (P<0.001). The pairwise comparison indicated the NM condition was significantly lower than both SM (P=0.003) and BM (P<0.001), while there was no difference between SM and BM (P=0.365) (Figure 4B, Table S4).
Mask symptomology
Table 3 presents the mask symptomology. On specific questioning after exercise, the BM condition was perceived to make exercise feel worse compared to the SM (P=0.011). The BM was also perceived to make breathing more difficult compared to SM condition (P=0.048). No other differences in mask symptomology were shown.
Table 3
Questions | Surgical mask (n=30) | Buff mask (n=30) | P value |
---|---|---|---|
1. How did you find exercise wearing a mask? | 6.0 (4.0–7.0) | 7.0 (5.0–9.0)* | 0.0108* |
2. How was your breathing? | 6.0 (4.0–7.0) | 7.0 (5.0–8.0)* | 0.0480* |
3. How did you perceive your breathing? | 6.5 (5.0–7.0) | 7.0 (5.0–8.0) | 0.6454 |
4. How does the inside of your mask feel? | 7.5 (6.0–9.0) | 8.5 (7.0–10.0) | 0.1306 |
5. Did you experience any adverse events? | 0.2882 | ||
No | 21 (70.0) | 16 (53.3) | |
Yes | 9 (30.0) | 14 (46.7) | |
6. Did you feel thirsty? | 4.5 (2.3–6.8) | 5.5 (1.3–7.0) | 0.5457 |
7. How was your performance affected? | 4.0 (2.3–6.0) | 4.5 (3.0–6.0) | 0.5002 |
Data are presented as median (interquartile range) or n (%). *, significant difference between masks. The rating of self-reported mask symptomology was examined in seven items, each ranking from 0–10 on a Likert scale.
Discussion
Key findings
The primary aim of this study partially confirm the hypothesis that masks would reduce SpO2 while undertaking moderate intensity exercise. Specifically, both the BM and the SM resulted in significant reductions in SpO2 after the commencement of exercise. However, during exercise, SpO2 was only significantly reduced in the BM condition compared to the NM condition at T1 and T2.
The second aim of this study was to examine other physiological and subjective outcomes of wearing masks during exercise. Physiologically, both mask conditions resulted in significantly higher RR, and thus a significantly lower EtCO2, compared to NM condition. Subjectively, both mask conditions resulted in significant RPB, with both increasing continually throughout exercise stages. Considering exercise intensity was the same for all conditions, the physiological and RPB data add further evidence to previous studies showing masks act as a physical barrier to airflow (20,21). We acknowledge, however, that the lack of blinding to conditions may have biased the subjective responses for the RPB, but given the nature of the study design, this was unavoidable. Importantly, however, while this study showed masks resulted in a significant, sustained reduction in SpO2 after the commencement of exercise, these SpO2 reductions were only 1–2%. The results suggest participants compensated for the increased metabolic load when considered alongside the absence of significant differences for either HR or RPE.
A further physiological measure that changed between conditions was EBT. During exercise, EBT increases with increasing metabolic demand (22). The a-priori analysis demonstrated significant increases in EBT from rest to exercise, with both mask conditions recording larger increases. This suggests masks increase metabolic requirements, as previous studies showed (23). Yet, the post-priori analysis, where the ambient temperature was adjusted for, showed no increase in EBT for any condition throughout exercise. Therefore, we concede the temperature sensors may not have recorded the expired air’s actual temperature, especially considering previous studies have shown differences in EBT between masks (24). Nevertheless, the significantly increased EBT in both mask conditions may indicate expired air accumulation, as shown in previous studies (25), providing further evidence of a barrier effect of masks. Furthermore, the accumulation of exhaled air could explain the small yet sustained decrease in oxygen, namely, hotter environmental temperatures reduce the amount of oxygen molecules, as well as a small accumulation of CO2 inside the mask. It is uncertain how EBT can be accurately measured while wearing a facemask. Previous studies have utilised respiratory analysing tools, such as a MetCart, while wearing face masks (26), but issues around mask sealing and leakage would remain.
Previous studies examining SpO2 changes in healthy, physically active people showed conflicting findings. Driver et al. showed significant reductions in SpO2 during the later stages of a maximal cardiopulmonary test while wearing a ‘cloth’ (similar to the BM condition) (27). They also showed worsening RPB (dyspnoea in their study). In contrast to our study, they showed significantly higher HR in the masked condition but a significantly lower RR. These differences are likely due to the disparities in exercise methodologies.
The closest in design to our study was Lässing et al., who showed no changes in SpO2 while cycling at 50% maximal workload for 30 min while wearing a SM (26). Workload variations could possibly explain the difference. However, this would require further investigation. Nevertheless, Lässing et al. demonstrated reductions in oxygen uptake and increased upper airway resistance and cardiac output, indicating similar findings to ours that mask coverings can result in increased metabolic load.
Another similar study was Roberge et al., who also showed no differences in SpO2 when wearing a SM while walking on a treadmill (25). The difference in exercise modality likely explains the differences in SpO2 results between their study and Lässing et al (26). Roberge et al. also showed the SM increased the RR, further indicating a barrier effect of masks.
Jones et al. showed no differences in SpO2 for a ‘cloth’, SM, and a ‘filtering facepiece-3’ mask compared to not wearing a mask (14). This study allowed participants to choose either cycling, running, or rowing at moderate-to-high intensity exercise, and it did not appear to have a set, common intensity. Furthermore, participants undertook four bouts of 15-min exercise with a 5-min break. The differences in methodology likely explain the differences in results. Shein et al. also showed no differences in SpO2 or EtCO2, when wearing a ‘cloth’ mask (28). Similar to Jones et al., this study utilised six bouts of 10 min of “walking briskly”, defined as an increase in HR of 10 beats per min. Again, the differences in methodology likely explain the differences in results.
Implications and actions needed
Overall, the results of this study add further evidence that commonly available public masks produce small reductions in SpO2 compared to not wearing a mask at the commencement of exercise, but these reductions do not worsen throughout moderate intensity exercise in healthy people. As there appear to be no changes in RPE or HR, it is feasible to advise healthy people to wear a face mask while participating in short duration, moderate intensity exercise from a public health perspective. It further implies that it is safe to wear a face mask engaging in <30-min moderate outdoor exercise and during less intense activities of daily living, for example, when commuting, doing household chores and shopping. Given the effectiveness of even basic single layer cloth masks at reducing COVID-19 transmission (29), this is especially pertinent for indoor gym settings. A further potential implication of this study is the use of masks during any outbreak of respiratory illness. It was noted that in 2020, there was a drastic reduction in influenza cases, although this reduction occurred when both ‘lockdowns’ alongside facemask wearing co-occurred (30). Nevertheless, this still suggests that facemask wearing should be commonplace during periods of common respiratory viruses, but that it is safe to do moderate intensity exercise while wearing a facemask and undertaking activities of daily living.
Strengths and limitations
The study followed after researcher’s experience during the COVID-19 pandemic. However, the findings can be applied to any healthy person wearing a mask for any reason, including future pandemic situations. The strengths of this study include an adequately powered sample size, an exercise that mimicked real-world physical activity, continuous physiological measures throughout exercise, and comprehensive subjective assessment. A further strength of this study is examining the impact of a ‘reusable’ cloth/fabric BM, which has become increasingly popular throughout the COVID-19 pandemic. This study utilised a population sample, including only healthy, physically active people. Therefore, we cannot infer our findings to children/adolescents, or clinical populations, such as those with cardio-respiratory conditions. Therefore, future studies should aim to recruit a large sample size, including children/adolescents and clinical populations.
Conclusions
In conclusion, masks readily available to the public reduce SpO2, which does not worsen during moderate intensity exercise of short duration in healthy people. There appears to be a slight increase in metabolic load, as well as increased RPB (dyspnoea). However, these were not reflected in HR or RPE. Therefore, it is reasonable for healthy people to wear masks while engaging in 30-min moderate exercise, as well as during other mild activities of daily living, including commuting, household chores and shopping.
Acknowledgments
Our appreciation goes to the following people without whom this project would not have been possible: Andre van Tonder, CEO of Channels Medical Solutions (Pty) Ltd, South Africa, for lending us the portable capnograph and pulse oximeter metabolic analysers (LifeSense® II, Nonin Medical, Inc.); Karl Dahling (Steve Biko Hospital) for lending us the portable Fluke® 189 multi-meter with Type-K integrated temperature probe (Fluke Process Instruments, Berlin, Germany).
Funding: None.
Footnote
Reporting Checklist: The authors have completed the CONSORT reporting checklist for randomised crossover RCTS. Available at https://jtd.amegroups.com/article/view/10.21037/jtd-23-1178/rc
Trial Protocol: Available at https://jtd.amegroups.com/article/view/10.21037/jtd-23-1178/tp
Data Sharing Statement: Available at https://jtd.amegroups.com/article/view/10.21037/jtd-23-1178/dss
Peer Review File: Available at https://jtd.amegroups.com/article/view/10.21037/jtd-23-1178/prf
Conflicts of Interest: All authors have completed the ICMJE uniform disclosure form (available at https://jtd.amegroups.com/article/view/10.21037/jtd-23-1178/coif). The authors have no conflicts of interest to declare.
Ethical Statement:
Open Access Statement: This is an Open Access article distributed in accordance with the Creative Commons Attribution-NonCommercial-NoDerivs 4.0 International License (CC BY-NC-ND 4.0), which permits the non-commercial replication and distribution of the article with the strict proviso that no changes or edits are made and the original work is properly cited (including links to both the formal publication through the relevant DOI and the license). See: https://creativecommons.org/licenses/by-nc-nd/4.0/.
References
- Fearnley L, Wu X. Beyond Asian ‘mask culture’: understanding the ethics of face masks during the Covid-19 pandemic in Singapore. Crit Public Health 2023;33:343-54.
- Howard J, Huang A, Li Z, et al. An evidence review of face masks against COVID-19. Proc Natl Acad Sci U S A 2021;118:e2014564118. [Crossref] [PubMed]
- Bartsch SM, O'Shea KJ, Chin KL, et al. Maintaining face mask use before and after achieving different COVID-19 vaccination coverage levels: a modelling study. Lancet Public Health 2022;7:e356-65. [Crossref] [PubMed]
- Clark E. Habitual mask-wearing is likely helping Japan, Singapore and South Korea bring daily Omicron deaths down, epidemiologists say. Australian Broadcasting Corporation; 2022. Available online: https://www.abc.net.au/news/2022-08-01/japan-singapore-south-korea-omicron-wave-and-mask-wearing-impact/101266844
- Hale T, Angrist N, Kira B,et al. Variation in government responses to COVID-19. 2020. Available online: https://www.bsg.ox.ac.uk/covidtracker
- Ai X, Yang J, Lin Z, et al. Mental Health and the Role of Physical Activity During the COVID-19 Pandemic. Front Psychol 2021;12:759987. [Crossref] [PubMed]
- Almstrand AC, Bake B, Ljungström E, et al. Effect of airway opening on production of exhaled particles. J Appl Physiol (1985) 2010;108:584-8. [Crossref] [PubMed]
- Blanco JH, Janse van Rensburg DC. Should people wear a face mask during exercise: What should clinicians advise? Br J Sports Med 2021. Available online: https://blogs.bmj.com/bjsm/2020/06/12/should-people-wear-a-face-mask-during-exercise-what-should-clinicians-advise/
- Hopkins SR, Dominelli PB, Davis CK, et al. Face Masks and the Cardiorespiratory Response to Physical Activity in Health and Disease. Ann Am Thorac Soc 2021;18:399-407. [Crossref] [PubMed]
- Chan NC, Li K, Hirsh J. Peripheral Oxygen Saturation in Older Persons Wearing Nonmedical Face Masks in Community Settings. JAMA 2020;324:2323-4. [Crossref] [PubMed]
- Pifarré F, Zabala DD, Grazioli G, et al. COVID-19 and mask in sports. Apunts Sports Medicine 2020;55:143-5.
- Rosa BV, Rossi FE, Moura HPDSN, et al. Effects of FFP2/N95 face mask on low- and high-load resistance exercise performance in recreational weight lifters. Eur J Sport Sci 2022;22:1326-34. [Crossref] [PubMed]
- Reychler G, Straeten CV, Schalkwijk A, et al. Effects of surgical and cloth facemasks during a submaximal exercise test in healthy adults. Respir Med 2021;186:106530. [Crossref] [PubMed]
- Jones N, Oke J, Marsh S, et al. Face masks while exercising trial (MERIT): a cross-over randomised controlled study. BMJ Open 2023;13:e063014. [Crossref] [PubMed]
- Salter S. Reinventing Cloth Masks in the Face of Pandemics. Risk Anal 2021;41:731-44. [Crossref] [PubMed]
- Borg G. Perceived exertion as an indicator of somatic stress. Scand J Rehabil Med 1970;2:92-8.
- Epstein D, Korytny A, Isenberg Y, et al. Return to training in the COVID-19 era: The physiological effects of face masks during exercise. Scand J Med Sci Sports 2021;31:70-5. [Crossref] [PubMed]
- Fikenzer S, Uhe T, Lavall D, et al. Effects of surgical and FFP2/N95 face masks on cardiopulmonary exercise capacity. Clin Res Cardiol 2020;109:1522-30. [Crossref] [PubMed]
- R: A language and environment for statistical computing. R Foundation for Statistical Computing, Vienna, Austria: 2021. Available online: https://www.R-project.org/
- Fukushi I, Nakamura M, Kuwana SI. Effects of wearing facemasks on the sensation of exertional dyspnea and exercise capacity in healthy subjects. PLoS One 2021;16:e0258104. [Crossref] [PubMed]
- Poon ET, Zheng C, Wong SH. Effect of Wearing Surgical Face Masks During Exercise: Does Intensity Matter? Front Physiol 2021;12:775750. [Crossref] [PubMed]
- Popov TA, Kralimarkova TZ, Labor M, et al. The added value of exhaled breath temperature in respiratory medicine. J Breath Res 2017;11:034001. [Crossref] [PubMed]
- Your lungs and exercise. Breathe (Sheff) 2016;12:97-100. [Crossref] [PubMed]
- Li Y, Tokura H, Guo YP, et al. Effects of wearing N95 and surgical facemasks on heart rate, thermal stress and subjective sensations. Int Arch Occup Environ Health 2005;78:501-9. [Crossref] [PubMed]
- Roberge RJ, Kim JH, Benson SM. Absence of consequential changes in physiological, thermal and subjective responses from wearing a surgical mask. Respir Physiol Neurobiol 2012;181:29-35. [Crossref] [PubMed]
- Lässing J, Falz R, Pökel C, et al. Effects of surgical face masks on cardiopulmonary parameters during steady state exercise. Sci Rep 2020;10:22363. [Crossref] [PubMed]
- Driver S, Reynolds M, Brown K, et al. Effects of wearing a cloth face mask on performance, physiological and perceptual responses during a graded treadmill running exercise test. Br J Sports Med 2022;56:107-13. [Crossref] [PubMed]
- Shein SL, Whitticar S, Mascho KK, et al. The effects of wearing facemasks on oxygenation and ventilation at rest and during physical activity. PLoS One 2021;16:e0247414. [Crossref] [PubMed]
- Dbouk T, Drikakis D. On respiratory droplets and face masks. Phys Fluids (1994) 2020;32:063303.
- Jones N. How COVID-19 is changing the cold and flu season. Nature 2020;588:388-90. [Crossref] [PubMed]