Yiqigubiao pill treatment regulates Sirtuin 5 expression and mitochondrial function in chronic obstructive pulmonary disease
Highlight box
Key findings
• Yiqigubiao (YQGB) pill treatment can elevate Sirtuin 5 (SIRT5) expression, restore mitochondrial function in chronic obstructive pulmonary disease (COPD), and exert protective effects.
What is known and what is new?
• SIRT5 is a key mediator in maintaining mitochondrial energy metabolism, and decreased SIRT5 can lead to mitochondrial dysfunction, which is one of the important areas for studying the pathogenesis of COPD.
• YQGB pill can increase the expression of SIRT5 in cells, improve mitochondrial function in the absence of SIRT5, and can improve some lung function indicators in COPD patients.
What is the implication, and what should change now?
• The improvement of lung function in COPD patients by YQGB is worth further research in the future.
Introduction
Chronic obstructive pulmonary disease (COPD) is a heterogeneous group of pathophysiological bases of airway inflammation and its anti-inflammatory response, characterized by persistent respiratory symptoms and airflow limitation (1). COPD is a serious health problem and constitutes the fourth leading cause of death worldwide (2). It is expected that 4.5 million people will die from COPD each year by 2030 (3). These patients often experience symptoms such as dyspnea, fatigue, poor sleep, morning headaches, and energy loss, which reduce their quality of life and increase the medical burden (4). In fact, COPD affects 16 million people, with medical costs exceeding US $32.1 billion (5). A significant number of patients with COPD experience exacerbations that eventually lead to higher morbidity associated with such events (6). Due to the complexity of maintaining chronic inflammation and tissue destruction, more emphasis should be placed on diagnosis and treatment in the early stages of the disease to make it possible to slow progression.
Currently, treatments for COPD include inhaled corticosteroids and bronchodilators (7,8). Although these treatments are effective in relieving symptoms, some side effects inevitably occur (9,10). Therefore, there is an urgent need to find better treatments. Traditional Chinese medicine (TCM) plays a vital role in improving the respiratory symptoms of COPD and reducing exacerbations (11-13). The Yiqigubiao (YQGB) pill is a TCM compound preparation, and studies have confirmed that it can be applied in the treatment of airway COPD (14,15). However, the mechanism of action of YQGB is not well defined.
The pathobiological processes of COPD include persistent inflammation, oxidative and nitrosative stress, impaired cellular repair, and cell death (16). Cigarette smoking is a major risk factor for COPD, and cigarette smoke extract (CSE)-mediated oxidative stress is considered to be one of the causes of glucocorticoid resistance (17,18). The lung is a direct target of reactive oxygen species (ROS) and free radical oxidative damage, while mitochondria produce large amounts of ROS (19). It is becoming increasingly clear that aberrant mitochondrial signaling and mitochondrial dysfunction underlie the pathomechanisms leading to COPD (20). Sirtuin 5 (SIRT5), which is exclusively found in mitochondria, is a nicotinamide adenine dinucleotide (NAD)+-dependent deacetylase involved in the oxidative stress response by regulating mitochondrial metabolism and antioxidant mechanisms (21,22). However, research on the role of SIRT5 in the oxidative stress response is still in its infancy. It is not clear whether SIRT5 plays a role in regulating the oxidative stress response in COPD patients.
To understand the efficacy and mechanism of action of YQGB in treating COPD, clinical trials and cellular experiments were conducted. The results suggest that the alleviation of clinical symptoms of COPD by YQGB is associated with the recovery of mitochondrial dysfunction. YQGB can increase the expression of SIRT5, as well as inhibit the production of ROS in the absence of SIRT5, and improve mitochondrial function, which may be one of its therapeutic mechanisms in the treatment of COPD. We present this article in accordance with the MDAR reporting checklist (available at https://jtd.amegroups.com/article/view/10.21037/jtd-23-1115/rc).
Methods
Materials
These experimental materials and equipment include human bronchial epithelial (HBE) cells (Beinabio, Beijing, China), keratinocyte medium (KM) medium (Hyclone, Logan, UT, USA), fetal bovine serum (FBS; GIBCO, Calb, CA, USA), cyanine chain double antibodies (Zeye Biology, Shanghai, China), MTT (Sigma, St. Louis, MO, USA), dimethyl sulfoxide (DMSO; Invitrogen, USA), ultra-clean table (Suzhou Antai Technology Co., Ltd., Suzhou, China), 96-well culture plate (Costar Company, Washington, USA), enzyme label detector (Thermo MK3, Waltham, MA, USA), shaking table (Qilinbeier TS-1000, Haimen, China), cell incubator (Thermo Scientific 8000, USA), optical microscope (XDS-1A, USA). Trizol (Thermo, Waltham, MA, USA), diethylpyrocarbonate (DEPC) treated water (CTCC, Shanghai, China), chloroform/isopropyl alcohol/anhydrous ethanol (Shanghai National Medicine, Shanghai, China), SYBR Green PCR Kit (Thermo F-415XL, Waltham, MA, USA), reverse transcription kit (Thermo #K1622, Waltham, MA, USA), cryogenic centrifuge (American Sigma 3K15, St. Louis, MO, USA), real-time detector (American ABI-7500, USA) and flow cytometer (BD Accuri C6, USA).
Diagnostic criteria for COPD
Diagnostic criteria for COPD (refer to “GOLD 2019 edition”): forced expiratory volume in one second/forced vital capacity (FEV1/FVC) less than 70% after bronchodilators used in pulmonary function tests; previous history of smoking and/or exposure to environmental occupational pollution; cough, sputum, dyspnea and other clinical symptoms; bronchial asthma, bronchiectasis, congestive heart failure, tuberculosis and other diseases being ruled out.
Diagnosis of COPD in stable stage: the symptoms of cough, sputum, and shortness of breath were stable or mild, and the patient was not treated in outpatient or inpatient treatment due to aggravation of the condition in the past 3 months. He had been regularly inhaling drugs for outpatient follow-up.
Inclusion/exclusion criteria
Inclusion criteria:
- Volunteer subjects;
- Age 40–75 years old;
- In line with Western diagnostic criteria and staging criteria for COPD: stable COPD patients;
- COPD patients treated with YQGB;
- Patients who did not take hormones or other immunosuppressants within 1 month and did not receive desensitization therapy.
Exclusion criteria:
- Patients with pneumothorax, pleural effusion, lung cancer, active pulmonary tuberculosis and other serious lung diseases;
- Patients with severe cardiovascular and cerebrovascular diseases, liver and kidney diseases, and blood system diseases;
- Patients with neurological diseases, skeletal muscle dysfunction and psychosis;
- Patients with tumor, hyperlipidemia, type 2 diabetes;
- Patients with congenital or acquired immune deficiency;
- Patients who take large amounts of hormones for a long time.
Sample collection
Thirty-four patients with COPD and 17 healthy control patients were randomly recruited from outpatients of The Fourth Affiliated Hospital of Xinjiang Medical University. The patients were required to meet the diagnostic criteria for stable COPD. All COPD patients were randomly divided into oral YQGB group (n=17) and COPD group (n=17) while receiving conventional treatment for 24 weeks. The dose of YQGB was 10 pills per dose (equivalent to 5 g of native medicinal materials), three times daily. The patient’s prescription adherence was monitored by doctors. The study was conducted in accordance with the Declaration of Helsinki (as revised in 2013). The use of human blood samples described in this study was approved by the ethics committee of The Fourth Affiliated Hospital of Xinjiang Medical University (approval No. 2020XE0125). All subjects gave written informed consent to participate.
The detailed protocol for blood collection: (I) ethylenediaminetetraacetic acid (EDTA) anticoagulant blood vessel collection was used to collect fasting 15–20 mL whole blood of each patient in the morning and it was mixed upside down to ensure the anticoagulant effect. (II) Three mL fresh blood was taken and the supernatant was centrifuged and put into the frozen storage tube in the refrigerator at −80 ℃ for subsequent detection of other indicators. The remaining blood cells were measured by flow cytometry for ROS and membrane potential (done within 6 hours). (III) The remaining whole blood was divided into 3–4 tubes with 5 mL of each tube for mitochondrial extraction.
To evaluate lung function, FEV1/FVC, FEV1, and FVC were measured by Master Screen PET601-1/IP20 (Jader, Free State of Bavaria, Germany).
Cell culture and treatment
The HBE cells were purchased from Beinabio (Beijing, China). The culture conditions were 37 ℃, 5% CO2 in KM containing 10% FBS (GIBCO, Galb, CA, USA) and cyanine chain double antibodies (Zeye Biology). Retail cigarettes with 11 mg of tar per cigarette, 1 mg of smoke nicotine, and 13 mg of smoke carbon monoxide were selected to prepare CSE. The filter tip was syringed after ignition, and 600 mL of smoke was injected into a 25 mL glass bottle, constructing a 100% stock solution. The stock solution was diluted in KM and used to treat HBE cells at 1×105 cells/mL. YQGB was diluted to 0.1 mg/mL in KM and used to treat the CSE-treated cells.
MTT detection
CSE was diluted into different concentrations (1.25%, 2.5%, 5%, 10%, and 20%) with KM. The cell concentration was adjusted to 0.8×104 cells/mL and stimulated with different concentrations of CSE, and the MTT assay was performed after 6, 12, 24, and 48 h of stimulation.
Each well of a 96-well plate was provided with 20 µL MTT (5 mg/mL) and continue cultured for 4 h at 37 ℃ in a 5% CO2 incubator. Furthermore, 150 µL DMSO was added to each well, and the absorbance value of each well was measured at 492 nm after mixing.
Transfection of plasmid
The cell concentration was adjusted to 2×105 cells/mL, siRNA SIRT5 (siSIRT5) was transfected by Lipofectamine RNAiMAX Reagent (Invitrogen), and quantitative real-time polymerase chain reaction (qRT-PCR) was used to verify the transfection efficiency. The SiRNA sequences targeting SIRT5 were 5'-GGAGCAAGGAGCCCAACGCCGGGCA-3' and 5'-CCACAAGAGGTACATCGAGTTTTAA-3'. The cells were grouped as follows: HBE, COPD, COPD + siRNA negative control (NC), COPD + siSIRT5, COPD + YQGB, and COPD + siSIRT5 + YQGB. The siRNA NC is a scrambled siRNA.
qRT-PCR
Mitochondria were extracted from the peripheral blood of patients with COPD and control patients using a mitochondria extraction kit (Beyotime, Shanghai, China). The total RNA of HBE cells or mitochondria was extracted by Trizol (Thermo). The total RNA was reverse transcribed into cDNA using a reverse transcription kit (Thermo). The qRT-PCR was performed using a SYBR Green PCR kit (Thermo). Glyceraldehyde-3-phosphate dehydrogenase (GAPDH) was used as an internal reference gene. The relative expression of genes of interest was analyzed using the 2–ΔΔCT method. The primer sequences were as follows: SIRT5, F: 5'-TTAGAAAGCAGCCGTGGAGACA-3', R: 5'-TCGACTTGGGACAATCTGGAGAGG-3'; GAPDH, F: 5'TGTTGCCATCAATGACCCCTT-3', R: 5'-CTCCACGACGTACTCAGCG-3'.
Western blot
Cells or mitochondria were lysed on ice with a cell lysis buffer [main component is 50 mM Tris (pH 7.40), 150 mM NaCl, 1% TritonX-100, 1% sodium deoxycholate, 0.1% sodium dodecyl sulphate (SDS), 2 mM sodium pyrophosphate, 25 mM B-glycerophosphate, 1 mM EDTA, 1 mM Na3VO4, 0.5 µg/mL leupeptin, etc.]. The extracted total proteins were separated in SDS-polyacrylamide gel electrophoresis (SDS-PAGE) and transferred to polyvinylidene difluoride membranes. Primary antibody was diluted to 1:2,000 and secondary antibody was diluted to 1:5,000. Skim milk blocking was followed by primary antibody (SIRT5, Rabbit pAb, ABclonal, A5784, Beijing, China) incubation, secondary antibody incubation, and the detection of protein bands with enhanced chemiluminescence. After densitometry analysis of ImageJ (Bethesda, USA), the gray value of the target protein is divided by the gray value of the internal reference protein in the excel table for normalization processing. GAPDH (Rabbit pAb, ABclonal, AC001) served as an internal reference protein. All antibodies were purchased from Abcam (Cambridge, UK). GE Amersham Imager600 chemiluminescence instrument (London, UK) was used.
Detection of mitochondrial membrane potential
For recruited patients and healthy people, including 17 patients in the normal control group, 17 patients in the COPD group and 17 patients taking YQGB pills, peripheral blood lymphocytes were extracted using a lymph extraction kit (TBD, Tianjin, China). MitoScreen (JC-1) (BD) was used to detect the level of the mitochondrial membrane potential of peripheral blood lymphocytes in different groups. The extracted lymphocytes were stained using JC-1 and incubated for 15 min in the dark. Flow cytometry was performed after resuspension of the assay buffer.
The mitochondrial membrane potential of HBE cells was detected using a mitochondrial membrane potential detection kit (Solarbio, Beijing, China). The cell concentration was adjusted to 1×106 cells/mL and then added to a 2 µm JC-1 probe, and the cells were incubated for 30 min at 37 ℃ in the dark. After being washed three times with phosphate buffered saline (PBS), the cells were stained with 4',6-diamidino-2-phenylindole (DAPI) to determine their nuclei, and then they were photographed and observed under a fluorescence microscope.
ROS detection
2',7'-Dichlorodihydrofluorescein diacetate (DCFH-DA) (Solarbio) was diluted at a 1:1,000 rate in a serum-free medium to produce a final concentration of 10 µmol/L. The 16 HBE cells were suspended in diluted DCFH-DA at a cell concentration of 1×107 cells/mL and incubated for 20 min at 37 ℃. They were thoroughly mixed by being inverting every 5 min to allow the probe and cells to come into contact. The cells were washed three times with PBS and collected, and fluorescence was detected.
Adenosine triphosphate (ATP) detection
The ATP content of the cells in each group was measured using human ATP enzyme-linked immunosorbent assay (ELISA) kit (Jiyinmei, Shanghai, China). The Microelisa stripplate provided in this kit was pre-coated with an antibody specific to ATP. Standards or samples were added to the appropriate Microelisa stripplate wells and combined with the specific antibody. Horseradish peroxidase-labeled antibody was used for incubation and color development using the 3,3',5,5'-tetramethylbenzidine (TMB) solution. The optical density was measured spectrophotometrically at a wavelength of 450 nm.
Statistical analysis
The SPSS software (v21.0) was used for statistical analysis. The Student’s t-test was used to compare the differences between groups, and the Chi-square test was used for categorical variables. Data that conforms to a normal distribution was represented as “mean ± standard deviation (SD)”; quantitative data that did not conform to a normal distribution was represented as “median (Q25–Q75)” using the quartile method and P<0.05 was considered statistically significant.
Results
General data
Among the baseline characteristics between patients with COPD and healthy control patients, smoking and body mass index (BMI) made a significant difference, but the other characteristics showed no significant differences between the two groups (Table 1). Patients with COPD who underwent YQGB had significant improvements in lung function at FEV1 and FEV1/FVC compared with placebo (Table 2).
Table 1
Item | COPD patients (n=34) | Healthy control (n=17) | χ2/t | P |
---|---|---|---|---|
Age (year, mean ± SD) | 68.7±8.6 | 66.5±7.6 | 1.366 | 0.36 |
BMI (kg/m², mean ± SD) | 23.4±3.7 | 26.7±3.8 | –3.054 | 0.004 |
Sex, n (%) | 0.10 | |||
Male | 24 (70.6) | 8 (47.1) | 2.684 | |
Female | 10 (29.4) | 9 (52.9) | ||
Ethnic group, n (%) | 0.83 | |||
Han | 21 (61.8) | 10 (58.8) | 0.041 | |
Other | 13 (38.2) | 7 (41.2) | ||
Occupation, n (%) | 0.05 | |||
In-service | 3 (8.8) | 5 (29.4) | 3.632 | |
Retired | 31 (91.2) | 12 (70.6) | ||
Smoking history, n (%) | 0.02 | |||
Yes | 21 (61.8) | 5 (29.4) | 4.747 | |
No | 13 (38.2) | 12 (70.6) |
COPD, chronic obstructive pulmonary disease; SD, standard deviation; BMI, body mass index.
Table 2
Item | COPD + YQGB (n=17) | COPD (n=17) | |||||
---|---|---|---|---|---|---|---|
Pre-treatment | Post-treatment | t/Z/P | Pre-treatment | Post-treatment | t/Z/P | ||
FEV1 (L), median (Q25–Q75) | 1.26 (0.94, 2.12) | 1.28 (1.02, 2.16) | −2.641/0.008 | 1.31 (0.78, 1.93) | 1.32 (0.79, 1.95) | −2.972/0.003 | |
FVC (L), median (Q25–Q75) | 2.11 (2.03, 3.42) | 2.12 (2.06, 3.43) | −2.153/0.03 | 2.37 (1.76, 2.90) | 2.37 (1.77, 2.90) | −3.106/0.002 | |
FEV1/FVC (%predicted), mean ± SD | 55.97±9.16 | 57.61±10.01 | 2.696/0.01 | 55.63±11.05 | 55.83±11.20 | 0.831/0.41 |
YQGB, Yiqigubiao; COPD, chronic obstructive pulmonary disease; FEV1, forced expiratory volume in one second; FVC, forced vital capacity; SD, standard deviation.
YQGB treatment improves mitochondrial function in COPD
To explore the effects of YQGB treatment, we detected the expression changes in SIRT5 in the peripheral blood mitochondria of patients with COPD before and after YQGB treatment. SIRT5 was significantly downregulated in patients with COPD compared with control patients (Figure 1A). When patients were treated with YQGB, SIRT5 mRNA levels were restored. This condition was also confirmed by the Western blot results (Figure 1B). The SIRT5 expression was significantly decreased in patients with COPD and was modulated by YQGB treatment. Flow cytometry revealed that mitochondrial membrane potential (JC-1) was significantly decreased in patients with COPD compared with control patients and was increased after YQGB treatment (Figure 1C). The examination of mitochondrial ROS (mtROS) revealed that it was significantly elevated in patients with COPD compared with the control patients and decreased after YQGB treatment (Figure 1D).
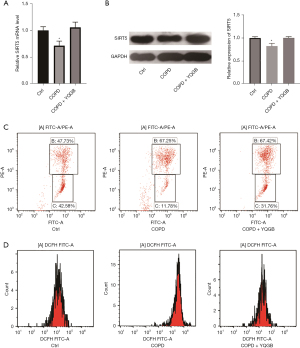
CSE stimulation inhibits the proliferation of HBE cells
HBE cells were treated with CSE, and it was observed that CSE stimulation affects cell proliferation (Figure 2A) and may have toxic effects on cells. Since the result was not obvious to the naked eye, which might be caused by being reflected in the inhibition of cell organelles and other fine points. Therefore, inhibition rate was used for qualification, which refers to the inhibition ability of drugs/reagents on cell proliferation and is mainly manifested in the proliferation ability of cells and the damage to cells. Using the MTT assay, it was found that the proliferation of cells would be inhibited to different degrees with the addition of different concentrations of CSE treatment compared with normal cells (Figure 2B). Its inhibition rate was dose-dependent with the added CSE concentration.
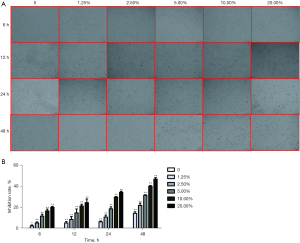
SIRT5 involved in YQGB treatment
To confirm the role of SIRT5 in CSE toxicity, siSIRT5 was transfected in HBE cells. The siRNA sequences with the best knockdown rate were selected using the qRT-PCR (Figure S1). The SIRT5 expression levels was significantly decreased after CSE stimulation compared with normal HBE control patients (Figure 3A). The knockdown of SIRT5 on CSE-stimulated HBE cells significantly decreased the expression of SIRT5. Compared with the CSE-stimulated HBE group, the expression of SIRT5 was considerably elevated after YQGB treatment. Even with the knockdown of SIRT5 on CSE-stimulated HBE cells, there was still an increase in gene expression levels with the addition of YQGB. In addition, cell proliferation in each group was similarly observed by microscopy (Figure 3B). It suggested that YQGB treatment considerably alleviated the decreased cell proliferation ability caused by CSE stimulation.
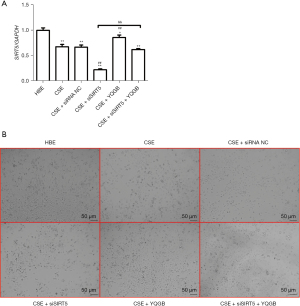
SIRT5 regulates mitochondrial function in YQGB treatment
The results of mitochondrial membrane potential showed that red fluorescence was weakened and green fluorescence was enhanced in the CSE stimulated group compared with HBE cells, indicating a decrease in mitochondrial membrane potential. Compared with the CSE group, the SIRT5 knockout group showed a further significant decrease in mitochondrial membrane potential. After YQGB treatment, the mitochondrial membrane potential not only increased in the CSE group, but also significantly increased in the SIRT5 knockout CSE group (Figure 4A,4B). ELISA kit was used to detect the level of ATP produced by cells, and it was found that the level of ATP produced by cells in the CSE group was significantly lower than that in the HBE group. After SIRT5 knockdown, the ATP content of cells in the CSE group was further decreased. After the CSE group with SIRT5 knock down received YQGB treatment, the ATP level was restored (Figure 4C). Furthermore, ROS detection by flow cytometry showed that CSE stimulation significantly increased ROS levels in HBE cells (Figure 5A,5B). Further knockdown of SIRT5 based on CSE stimulation resulted in a considerable increase in ROS levels. This was supported by a significant reduction in ROS levels following YQGB treatment. These results indicate that CSE stimulation can lead to the decrease in SIRT5 level, the increase in ROS level, and the decrease in mitochondrial function, while YQGB can increase the expression level of SIRT5, decrease ROS level, and alleviate the mitochondrial dysfunction of HBE cells induced by CSE stimulation, which is consistent with the results of clinical trials. At the same time, it was also found that even in the absence of SIRT5, YQGB can still inhibit the production of ROS, improve the mitochondrial membrane potential and ATP levels, and thus improve the mitochondrial dysfunction in COPD.
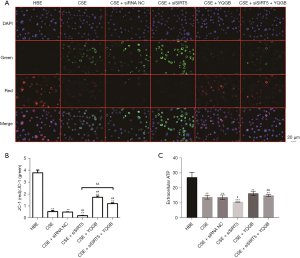
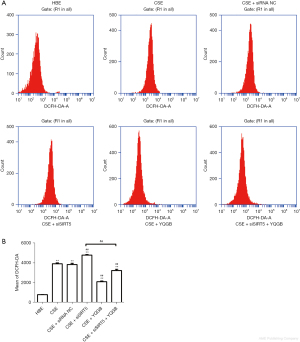
Discussion
The main pathophysiological manifestations of COPD are irreversible airway obstruction and progressive decline in lung function, especially in patients who are continuously exposed to risk factors such as smoking, biomass smoke, and air pollution (23,24). One of the main causes of COPD onset and disease progression is cigarette smoking, which can trigger oxidative stress and cause excessive inflammatory responses in the airways, alveoli and microvasculature. Cigarette extract can cause changes in mitochondrial morphology and function, change mitochondrial membrane potential, increase the production of superoxide dismutase, oxidize proteins, and decrease the expression of mitochondrial division genes (25). Similarly, cigarette smoking particles cause significant changes in mitochondrial structure by altering microtubule network and reducing matrix, causing mitochondrial swelling and thus interfering with mitochondrial energy production (26). In this study, the use of CSE resulted in the production of a large number of ROS in bronchial epithelial cells, decrease in membrane potential, and mitochondrial dysfunction, which was consistent with the relevant experimental reports in the literature reviewed above. ROS produced by CSE resulted in abnormal mitochondrial function and abnormal airway response, which was one of the main causes of intractable inflammation in COPD (27). In this study, CSE-stimulated HBE cells showed a decline in mitochondrial function, and the expression level of SIRT5 significantly decreased, while the mitochondrial function was restored after YQGB intervention. In clinical trials involving COPD patients with smoking history, SIRT5 expression level in peripheral blood of COPD group decreased, and increased after YQGB treatment, with lung function being improved. Therefore, we speculated that YQGB could improve mitochondrial dysfunction caused by CSE and regulate SIRT5 expression. This may be one of the potential mechanisms to improve lung function in patients with COPD.
Sirtuins (Sirts) are found in all organisms and are involved in many other processes that occur within cells, including participation in the regulation of the cell cycle and energy metabolism, apoptosis, and oxidative stress processes (28). In fact, the functions of Sirts have been implicated in the pathogenesis of respiratory diseases, including COPD (29). Wang et al. confirmed the involvement of SIRT5 in the process of COPD by inhibiting CSE-induced apoptosis of lung epithelial cells (30). The experimental results from this study found that SIRT5 expression was downregulated in patients with COPD and the CSE-stimulated HBE cells, which was accompanied by a decline in mitochondrial membrane potential, a decline in ATP content, and an increase in ROS levels. SIRT5 deletion causes a clear decrease in ATP levels in HEK293T cells (31). A deficiency in SIRT5 in mice causes defects in fatty acid metabolism and decreases ATP production (32). In addition, there is a potential link between the downregulation of SIRT5 and increased oxidative damage response in liver cancer tissues (33).
It has been shown that the pathogenesis of COPD is closely related to the disturbance of mitochondrial structure and function in lung epithelial cells (34). Observations in respiratory epithelial cells chronically exposed to CSE reveal that it caused similar aberrations in mitochondrial morphology (20,35). There is a potential link between these morphological alterations and mitochondrial dysfunction, including decreased ATP levels, loss of mitochondrial membrane potential, and increased mtROS levels (36,37). When mitochondrial damage is excessive, ROS can cause cellular damage that further propagates cellular stress responses or programmed cell death pathways (38). Accumulating evidence suggests that COPD may be a cellular aging process with increased ROS from oxidative stress (39), and mtROS inhibitors may be new therapeutic targets for COPD (40). Although the function of SIRT5 is cell- and context-specific, these studies all confirm that SIRT5 is involved in the progression of COPD by regulating mitochondrial damage. The results of this study also showed that after CSE stimulation, ROS in cells increased, membrane potential was significantly decreased, mitochondria were damaged, leading to cell damage and even apoptosis, cell morphology shrunk and became round, and cell density decreased in a poor state. After SIRT5 was knocked out, ROS increased a step compared with the previous studies, and ATP and mitochondrial membrane potential decreased again, indicating that SIRT5 was involved in the regulation of mitochondrial function in COPD cells. The loss of SIRT5 would cause more ROS to be produced in the cells, and the decline of mitochondrial membrane potential would lead to the damage of mitochondrial function, which was consistent with previous studies (41-44), and also proved that SIRT5 regulated mitochondrial function in COPD cells.
TCM has been widely used in the treatment of chronic lung diseases (45,46). YQGB is composed of Dangshen [DS, Codonopsis pilosula (Franch.)], Baizhu (BZ, Atractylodes macrocephala), Fuling [FL, Poria cocos (Schw.) Wolf], Chenpi (CP, Citrus reticulata Blanco), Banxia [BX, Pinellia ternata (Thunb.) Breit], Yiyiren [YYR, Coixlacryma-jobi L.var.mayuen (Roman.) Stapf], Fuxiaomai (FXM, Triticum aestivum L.), Zisu [ZS, Perilla frutescens (L.) Britt], Kuandonghua (KDH, Tussilago farfara L.), Huangqin (HQ, Scutellaria baicalensis Ceorgi), Yibeimu (YBM, Fritillariapallidiflora Schrenk), Pibaye [Eriobotrya japonica (Thunb.) Lindl] and Fangfeng [FF, Saponshnikovia divaricate (Turcz.) Schischk]. With beneficial effects of qi strengthening, spleen drying and phlegm relieving, it has been put into use in the form of TCM pills since 2004, and approved for clinical trials of investigational new drug in 2018. During the past decade of clinical use, it can reduce the number of acute exacerbations in COPD patients, extend the time interval, and shorten the duration of acute exacerbations, showing promising efficacy (47-50). This study found that YQGB was able to significantly alleviate pulmonary function in patients with COPD. Consistent with previous studies, YQGB improved the quality of life of stable COPD patients and reduced the number of acute exacerbation events per year (14). YQGB can significantly prolong the cough latency of COPD rat models, reduce cough frequency, and lower serum tumor necrosis factor (TNF) content (51). The expression of SIRT5 increased in patients with COPD after YQGB treatment, along with the recovery of mitochondrial function. These results suggest that YQGB may exert therapeutic effects by increasing SIRT5 expression and regulating mitochondrial functional pathways in patients with COPD.
Conclusions
This study demonstrated that YQGB was able to increase SIRT5 expression and reversing mitochondrial dysfunction from the cytostatic effects in patients with COPD and CSE stimulated HBE cells. These findings suggest that YQGB can treat COPD and improve pulmonary function indicators in COPD patients to a certain extent. The mechanism of action may be through increasing the expression level of SIRT5 and reversing mitochondrial dysfunction, thus showing therapeutic effect in the treatment of COPD.
Acknowledgments
Funding: This study was supported by
Footnote
Reporting Checklist: The authors have completed the MDAR reporting checklist. Available at https://jtd.amegroups.com/article/view/10.21037/jtd-23-1115/rc
Data Sharing Statement: Available at https://jtd.amegroups.com/article/view/10.21037/jtd-23-1115/dss
Peer Review File: Available at https://jtd.amegroups.com/article/view/10.21037/jtd-23-1115/prf
Conflicts of Interest: All authors have completed the ICMJE uniform disclosure form (available at https://jtd.amegroups.com/article/view/10.21037/jtd-23-1115/coif). The authors have no conflicts of interest to declare.
Ethical Statement: The authors are accountable for all aspects of the work in ensuring that questions related to the accuracy or integrity of any part of the work are appropriately investigated and resolved. The study was conducted in accordance with the Declaration of Helsinki (as revised in 2013). The use of human blood samples described in this study was approved by the ethics committee of The Fourth Affiliated Hospital of Xinjiang Medical University (approval No. 2020XE0125). All subjects gave written informed consent to participate.
Open Access Statement: This is an Open Access article distributed in accordance with the Creative Commons Attribution-NonCommercial-NoDerivs 4.0 International License (CC BY-NC-ND 4.0), which permits the non-commercial replication and distribution of the article with the strict proviso that no changes or edits are made and the original work is properly cited (including links to both the formal publication through the relevant DOI and the license). See: https://creativecommons.org/licenses/by-nc-nd/4.0/.
References
- Singh D, Agusti A, Anzueto A, et al. Global Strategy for the Diagnosis, Management, and Prevention of Chronic Obstructive Lung Disease: the GOLD science committee report 2019. Eur Respir J 2019;53:1900164. [Crossref] [PubMed]
- Brandsma CA, Van den Berge M, Hackett TL, et al. Recent advances in chronic obstructive pulmonary disease pathogenesis: from disease mechanisms to precision medicine. J Pathol 2020;250:624-35. [Crossref] [PubMed]
- Zhao K, Chen K, Huang Q, et al. Traditional Chinese medicine may reduce the dosage of systemic glucocorticoids in required patients with acute exacerbation of chronic obstructive pulmonary disease: Study protocol for a randomized placebo-controlled trial. Medicine (Baltimore) 2020;99:e20035. [Crossref] [PubMed]
- van Dijk M, Gan CT, Koster TD, et al. Treatment of severe stable COPD: the multidimensional approach of treatable traits. ERJ Open Res 2020;6:00322-2019. [Crossref] [PubMed]
- Celli BR, Navaie M, Xu Z, et al. Medication management patterns among Medicare beneficiaries with chronic obstructive pulmonary disease who initiate nebulized arformoterol treatment. Int J Chron Obstruct Pulmon Dis 2019;14:1019-31. [Crossref] [PubMed]
- Ritchie AI, Wedzicha JA. Definition, Causes, Pathogenesis, and Consequences of Chronic Obstructive Pulmonary Disease Exacerbations. Clin Chest Med 2020;41:421-38. [Crossref] [PubMed]
- Fan Y, Wen X, Zhang Q, et al. Effect of Traditional Chinese Medicine Bufei Granule on Stable Chronic Obstructive Pulmonary Disease: A Systematic Review and Meta-Analysis Based on Existing Evidence. Evid Based Complement Alternat Med 2020;2020:3439457. [Crossref] [PubMed]
- Oba Y, Keeney E, Ghatehorde N, et al. Dual combination therapy versus long-acting bronchodilators alone for chronic obstructive pulmonary disease (COPD): a systematic review and network meta-analysis. Cochrane Database Syst Rev 2018;12:CD012620. [Crossref] [PubMed]
- Calverley PM, Anderson JA, Celli B, et al. Salmeterol and fluticasone propionate and survival in chronic obstructive pulmonary disease. N Engl J Med 2007;356:775-89. [Crossref] [PubMed]
- Li J, Zhang H, Ruan H, et al. Effects of Chinese Herbal Medicine on Acute Exacerbations of COPD: A Randomized, Placebo-Controlled Study. Int J Chron Obstruct Pulmon Dis 2020;15:2901-12. [Crossref] [PubMed]
- Zu Y, Li D, Lei X, et al. Effects of the Chinese herbal formula San-Huang Gu-Ben Zhi-Ke treatment on stable chronic obstructive pulmonary disease: study protocol of a randomized, double-blind, placebo-controlled trial. Trials 2019;20:647. [Crossref] [PubMed]
- Gao Z, Jing J, Liu Y. Xiaoqinglong decoction (a traditional Chinese medicine) combined conventional treatment for acute exacerbation of chronic obstructive pulmonary disease: A systematic review and meta-analysis. Medicine (Baltimore) 2020;99:e19571. [Crossref] [PubMed]
- Zhang XW, Liu W, Jiang HL, et al. Dissection of Pharmacological Mechanism of Chinese Herbal Medicine Yihuo Huatan Formula on Chronic Obstructive Pulmonary Disease: A Systems Pharmacology-Based Study. Sci Rep 2019;9:13431. [Crossref] [PubMed]
- Li FS, Zhang YL, Li Z, et al. Randomized, double-blind, placebo-controlled superiority trial of the Yiqigubiao pill for the treatment of patients with chronic obstructive pulmonary disease at a stable stage. Exp Ther Med 2016;12:2477-88. [Crossref] [PubMed]
- Zhen G, Jing J, Dan X, et al. A Randomized Controlled Study of the Yi Qi Gu Biao Pill in the Treatment of Frequent Exacerbator Phenotype in Chronic Obstructive Pulmonary Disease (Lung and Spleen Qi Deficiency Syndrome). Evid Based Complement Alternat Med 2017;2017:9130804. [Crossref] [PubMed]
- Acquaah-Mensah GK, Malhotra D, Vulimiri M, et al. Suppressed expression of T-box transcription factors is involved in senescence in chronic obstructive pulmonary disease. PLoS Comput Biol 2012;8:e1002597. [Crossref] [PubMed]
- Oishi K, Matsunaga K, Shirai T, et al. Role of Type2 Inflammatory Biomarkers in Chronic Obstructive Pulmonary Disease. J Clin Med 2020;9:2670. [Crossref] [PubMed]
- Sundar IK, Yao H, Rahman I. Oxidative stress and chromatin remodeling in chronic obstructive pulmonary disease and smoking-related diseases. Antioxid Redox Signal 2013;18:1956-71. [Crossref] [PubMed]
- Tafani M, Sansone L, Limana F, et al. The Interplay of Reactive Oxygen Species, Hypoxia, Inflammation, and Sirtuins in Cancer Initiation and Progression. Oxid Med Cell Longev 2016;2016:3907147. [Crossref] [PubMed]
- Cloonan SM, Choi AM. Mitochondria in lung disease. J Clin Invest 2016;126:809-20. [Crossref] [PubMed]
- Seto E, Yoshida M. Erasers of histone acetylation: the histone deacetylase enzymes. Cold Spring Harb Perspect Biol 2014;6:a018713. [Crossref] [PubMed]
- Lin ZF, Xu HB, Wang JY, et al. SIRT5 desuccinylates and activates SOD1 to eliminate ROS. Biochem Biophys Res Commun 2013;441:191-5. [Crossref] [PubMed]
- Li LSK, Williams MT, Johnston KN, et al. Parental and life-course influences on symptomatic airflow obstruction. ERJ Open Res 2020;6:00343-2019. [Crossref] [PubMed]
- Petersen H, Sood A, Polverino F, et al. The Course of Lung Function in Middle-aged Heavy Smokers: Incidence and Time to Early Onset of Chronic Obstructive Pulmonary Disease. Am J Respir Crit Care Med 2018;198:1449-51. [Crossref] [PubMed]
- Fetterman JL, Pompilius M, Westbrook DG, et al. Developmental exposure to second-hand smoke increases adult atherogenesis and alters mitochondrial DNA copy number and deletions in apoE(-/-) mice. PLoS One 2013;8:e66835. [Crossref] [PubMed]
- Kennedy JR, Elliott AM. Cigarette smoke: the effect of residue on mitochondrial structure. Science 1970;168:1097-8. [Crossref] [PubMed]
- Jiang Y, Wang X, Hu D. Mitochondrial alterations during oxidative stress in chronic obstructive pulmonary disease. Int J Chron Obstruct Pulmon Dis 2017;12:1153-62. [Crossref] [PubMed]
- Kratz EM, Sołkiewicz K, Kubis-Kubiak A, et al. Sirtuins as Important Factors in Pathological States and the Role of Their Molecular Activity Modulators. Int J Mol Sci 2021;22:630. [Crossref] [PubMed]
- Mongelli A, Gaetano C. Controversial Impact of Sirtuins in Chronic Non-Transmissible Diseases and Rehabilitation Medicine. Int J Mol Sci 2018;19:3080. [Crossref] [PubMed]
- Wang Y, Zhu Y, Xing S, et al. SIRT5 prevents cigarette smoke extract-induced apoptosis in lung epithelial cells via deacetylation of FOXO3. Cell Stress Chaperones 2015;20:805-10. [Crossref] [PubMed]
- Zhang M, Wu J, Sun R, et al. SIRT5 deficiency suppresses mitochondrial ATP production and promotes AMPK activation in response to energy stress. PLoS One 2019;14:e0211796. [Crossref] [PubMed]
- Sadhukhan S, Liu X, Ryu D, et al. Metabolomics-assisted proteomics identifies succinylation and SIRT5 as important regulators of cardiac function. Proc Natl Acad Sci U S A 2016;113:4320-5. [Crossref] [PubMed]
- Chen XF, Tian MX, Sun RQ, et al. SIRT5 inhibits peroxisomal ACOX1 to prevent oxidative damage and is downregulated in liver cancer. EMBO Rep 2018;19:e45124. [Crossref] [PubMed]
- Mizumura K, Cloonan SM, Nakahira K, et al. Mitophagy-dependent necroptosis contributes to the pathogenesis of COPD. J Clin Invest 2014;124:3987-4003. [Crossref] [PubMed]
- Hoffmann RF, Zarrintan S, Brandenburg SM, et al. Prolonged cigarette smoke exposure alters mitochondrial structure and function in airway epithelial cells. Respir Res 2013;14:97. [Crossref] [PubMed]
- Agarwal AR, Yin F, Cadenas E. Short-term cigarette smoke exposure leads to metabolic alterations in lung alveolar cells. Am J Respir Cell Mol Biol 2014;51:284-93. [Crossref] [PubMed]
- Aghapour M, Remels AHV, Pouwels SD, et al. Mitochondria: at the crossroads of regulating lung epithelial cell function in chronic obstructive pulmonary disease. Am J Physiol Lung Cell Mol Physiol 2020;318:L149-64. [Crossref] [PubMed]
- Bolisetty S, Jaimes EA. Mitochondria and reactive oxygen species: physiology and pathophysiology. Int J Mol Sci 2013;14:6306-44. [Crossref] [PubMed]
- Zuo L, Prather ER, Stetskiv M, et al. Inflammaging and Oxidative Stress in Human Diseases: From Molecular Mechanisms to Novel Treatments. Int J Mol Sci 2019;20:4472. [Crossref] [PubMed]
- Wiegman CH, Li F, Ryffel B, et al. Oxidative Stress in Ozone-Induced Chronic Lung Inflammation and Emphysema: A Facet of Chronic Obstructive Pulmonary Disease. Front Immunol 2020;11:1957. [Crossref] [PubMed]
- Ou T, Yang W, Li W, et al. SIRT5 deficiency enhances the proliferative and therapeutic capacities of adipose-derived mesenchymal stem cells via metabolic switching. Clin Transl Med 2020;10:e172. [Crossref] [PubMed]
- Guedouari H, Daigle T, Scorrano L, et al. Sirtuin 5 protects mitochondria from fragmentation and degradation during starvation. Biochim Biophys Acta Mol Cell Res 2017;1864:169-76. [Crossref] [PubMed]
- Liu L, Peritore C, Ginsberg J, et al. Protective role of SIRT5 against motor deficit and dopaminergic degeneration in MPTP-induced mice model of Parkinson's disease. Behav Brain Res 2015;281:215-21. [Crossref] [PubMed]
- Li W, Yang Y, Li Y, et al. Sirt5 Attenuates Cisplatin-Induced Acute Kidney Injury through Regulation of Nrf2/HO-1 and Bcl-2. Biomed Res Int 2019;2019:4745132. [Crossref] [PubMed]
- Wang CY, Ding HZ, Tang X, et al. Effect of Liuweibuqi capsules on CD4(+)CD25(+)Foxp3(+) regulatory T cells, helper T cells and lung function in patients with stable chronic obstructive pulmonary disease complicated with lung Qi deficiency. J Thorac Dis 2018;10:2700-11. [Crossref] [PubMed]
- Wu J, Li X, Qin Y, et al. Jinwei Tang modulates HDAC2 expression in a rat model of COPD. Exp Ther Med 2018;15:2604-10. [Crossref] [PubMed]
- Jin J, Li F, Li Z, et al. Effects of Yiqi Gubiao Pills on Expressions of miRNA of Low FFMI COPD Patients. Chinese Journal of Information on Traditional Chinese Medicine 2019;26:16-22.
- Jin J, Wang J, Yao Z, et al. Effect of Yiqigubiao Pill Regulate Inflammatory Cytokines Through JAK/STAT Pathway in COPD Rats Model. Modernization of Traditional Chinese Medicine and Materia Medica-World Science and Technology 2018;20:1828-33.
- Jin J, Wang J, Yao Z, et al. Effect of Yiqi Gubiao Pill to Regulate the Balance of Th17/Treg in Patients with Chronic Obstructive Pulmonary Disease. Journal of Xinjiang Medical University 2018;41:1182-1185,1189.
- Li W. Clinical study on Yiqi Gubiao Pill for treating idiopathic pulmonary interstitial fiber of lung Qi deficiency type. Xin Med Univ 2018. Available online: https://kns.cnki.net/kcms2/article/abstract?v=C06iYwc_NfQBxUkPzT7yvX2MkstBRqAhY7mTCW9h7odSy8WEWb8knn62mI9J2Fk_oqSdSFZ0yphSzdfDALHsiNefkzeXa3ZD68IzipZfsdfFeWnifP2XYJb3umMDBktJ_3HcMQzgUMUps6vp_dsseg==&uniplatform=NZKPT&language=CHS
- Jiang L, Li F, Sun J, et al. Efficacy of Yiqigubiao pill on chronic obstructive pulmonary disease in rats with the disease induced by lipopolysaccharide and cigarette-smoke fumigation. J Tradit Chin Med 2020;40:983-91. [PubMed]