Pazopanib attenuated bleomycin-induced pulmonary fibrosis via suppressing TGF-β1 signaling pathway
Highlight box
Key findings
• Pazopanib attenuated bleomycin (BLM)-induced pulmonary fibrosis by suppressing the transforming growth factor-β1 (TGF-β1) signaling pathway.
What is known and what is new?
• Pazopanib is a drug for the treatment of renal cell carcinoma and advanced soft tissue sarcoma.
• In vivo experiments showed that pazopanib can alleviate pulmonary fibrosis caused by BLM, reduce the degree of collagen deposition and improve lung function. In vitro experiments showed that pazopanib suppressed TGF-β1-induced myofibroblast activation and promoted apoptosis and autophagy in myofibroblasts. Further mechanistic studies demonstrated that pazopanib inhibited the TGF-β1/Smad and non-Smad signaling pathways during fibroblast activation.
What is the implication, and what should change now?
• Pazopanib attenuated BLM-induced pulmonary fibrosis by suppressing the TGF-β1 signaling pathway. It has the same target as nintedanib and is a tyrosine kinase inhibitor.
Introduction
Idiopathic fibrosis is a progressive interstitial lung disease, the pathogenesis of which is unknown. The pathogenesis of idiopathic fibrosis is related to age. Idiopathic pulmonary fibrosis (IPF) is characterized by abnormal deposition of extracellular matrix (ECM) in the lung (1-3). Due to the aging of the population and the increasing severity of air pollution, the incidence of IPF is increasing annually (4,5). At present, there are only two drugs available on the market for treating idiopathic fibrosis, but their efficacy is limited (6). The development of new drugs for the treatment of IPF has become a hot topic of research and development.
In recent studies, many researchers have agreed that fibroblast activation, myofibroblast apoptosis and autophagy are vital for fibrotic progression (1,7). Transforming growth factor-β1 (TGF-β1) is a well-known core regulator of the development of pulmonary fibrosis (8) and is extremely upregulated in the lungs of IPF patients (9). High levels of myeloid cell activation, migration and proliferation are likely to occur, and TGF-β1 can induce fibroblasts to transform into non-fibroblasts (10). The phosphorylation of Smad2 and Smad3 is promoted by TGF-β1 and mainly acts on Smad2 and Smad3 pathways downstream of TGF-β1 to regulate fibroblast activation and ECM production (11,12). Autophagy is essential for survival, differentiation, development, and homeostasis because it can promote lysosomal degradation (13). ECM protein overexpression is a catastrophic component in the etiology of pulmonary fibrosis, and autophagy can downregulate the expression of ECM proteins (14,15). In addition, TGF-β1 strongly suppresses interleukin-1β (IL-1β)-induced apoptosis in myofibroblasts through two mechanisms, namely, inhibiting inducible nitric oxide synthase (iNOS) expression and restricting the decrease in Bcl-2 expression (16). Therefore, suppressing TGF-β1-induced fibroblast activation and ECM production and promoting myofibroblast autophagy and intriguing myofibroblast apoptosis are essential for treatment of fibrosis.
Pazopanib is a multityrosine kinase inhibitor with the same targets as nintedanib and was approved by the Food and Drug Administration (FDA) in June 2010 for treating metastatic renal cell carcinoma (RCC) (17,18). Pazopanib also has a positive impact on solitary fibrous tumor (SFT) by decreasing the size of the tumor in clinical trials (19). In a murine xenograft, pazopanib was found to be a possible agent against synovial sarcoma growth mainly via suppression of the PI3K-Akt pathway (18). Pazopanib can induce autophagic flux by increasing the number of LC3-green fluorescent protein (GFP) vesicles in sarcoma cells, the results of which revealed a reduction in tumor volume (20). Pazopanib can decrease the survival of A549 cells, possibly mainly through the induction of apoptotic cell death (21). However, the efficacy and pharmacological mechanism of pazopanib in pulmonary fibrosis have not been reported. In this study, we explored the antifibrotic effect of pazopanib on bleomycin (BLM)-induced pulmonary fibrosis in mice.
Our study clarified that pazopanib plays a key role in alleviating BLM-induced pulmonary fibrosis by suppressing fibroblast activation and promoting myofibroblast autophagy and apoptosis via the inhibition of TGF-β1/Smad and TGF-β1/non-Smad signaling. This study provides a theoretical and experimental basis for the clinical treatment of pulmonary fibrosis with pazopanib. We present this article in accordance with the ARRIVE reporting checklist (available at https://jtd.amegroups.com/article/view/10.21037/jtd-23-1349/rc).
Methods
BLM-induced animal model of pulmonary fibrosis
Six- to 8-week-old male C57BL/6 mice were purchased from Charles River (Beijing, China). All mice were housed and cared for in a pathogen-free facility at Nankai University Experimental Animal Center. The Nankai University Institutional Animal Care and Use Committee (IACUC) authorized all animal care and experimental procedures (project code: SCXK 2019-0001, date of approval: 14 January 2019; Approval No. SYXK 2014-0003), in compliance with institutional guidelines for the care and use of animals. The FDA of the United States and the European Drug Administration has approved the daily dose of pazopanib as 800 mg. The mice were divided into high-dose and low-dose groups according to the equivalent dose ratio of 88 mg/kg in different groups. The mice were randomly divided into five groups (n=7 per group): the control group, BLM group, nintedanib-treated group (100 mg/kg), low-dose pazopanib-treated group (50 mg/kg), and high-dose pazopanib-treated group (100 mg/kg). Nintedanib was purchased from Macklin Biochemical (Shanghai, China), and pazopanib was purchased from Dalian Meilun Biotechnology (Dalian, China). For 7–14 days, the mice were given either pazopanib, nintedanib, or water orally. In order to study the fibrotic response, mice were intratracheally injected with BLM (Medicine Co., Tokyo, Japan) at a dose of 2 U/kg body weight. The sham group received the same volume of saline intravenously. The drugs were administered daily the 7th day of modeling and continued through the 14th day. Mice were sacrificed on day 14, and lung tissues were harvested.
Cell culture
The Mlg and NIH-3T3 cell lines were kindly gifted from Professor Wen Ning of Nankai University. Mlg and NIH-3T3 cells were stimulated with TGF-β1 or pazopanib. Mouse primary pulmonary fibroblasts (PPFs) were isolated from the lung tissues of NaCl- or BLM-treated mice on day 14. All cells were cultured according to the established guidelines. These cells were cultured in Dulbecco’s Modified Eagle Medium (DMEM) (Solarbio, Beijing, China) supplemented with 10% fetal bovine serum (Gibco, New York, USA) in an incubator at constant temperature of 37 ℃ with 5% CO2.
Transfection methods
NIH-3T3 cells were seeded onto glass slides in 24-well plates, after which 1 µg of GFP-LC3B or mCherry-GFP-LC3B was transfected into NIH-3T3 cells with polyethylenimine (PEI) (Thermo Fisher Scientific, New York, USA) for 6 hours.
Western blot
The lysates of cells or tissues were prepared with RIPA buffer (Beyotime, Shanghai, China) supplemented with a cocktail (Sigma, New Jersey, USA) and quantified via a BCA protein assay (Beyotime). Sodium dodecyl sulfate polyacrylamide gel electrophoresis (SDS-PAGE) was used to detect protein expression. After the membranes were incubated with primary and secondary antibodies, immunoreactivity was detected via enhanced chemiluminescence (ECL) (Affinity, New York, USA). The secondary antibodies used were as follows: goat anti-rabbit immunoglobulin G (IgG)-horseradish peroxidase (HRP) (Abcam, London, UK) and goat anti-mouse IgG-HRP (Abcam). The primary antibodies used in this study are listed in Table 1.
Table 1
Antibody | Company, item No. |
---|---|
Anti-GAPDH | Affinity, AF7021 |
α-SMA | Affinity, BF9212 |
Anti-collagen I | Affinity, AF0134 |
Anti-Smad2 | Affinity, AF6449 |
Anti-Smad3 | Affinity, AF6362 |
Anti-Akt1/2/3 | Affinity, AF6259 |
Anti-Erk1/2 | Affinity, AF0155 |
Anti-caspase3 | Affinity, AF6311 |
Anti-caspase7 | Affinity, AF5118 |
Anti-caspase9 | Affinity, AF6348 |
Anti-SQSTM1/P62 | CST, 5144 |
Anti-β-tubulin | Affinity, AF7011 |
Anti-fibronectin | Affinity, AF0738 |
Anti-LC3A/B | CST, 4018 |
Anti-p-Smad2 (Ser467) | Affinity, AF3449 |
Anti-p-Smad3 (Ser423 + Ser425) | Affinity, AF8315 |
Anti-p-Akt1/2/3 (Ser473) | Affinity, AF0016 |
Anti-p-Erk1/2 (Thr202 + Thr204) | Affinity, AF1015 |
Anti-cleaved-caspase3 (Asp175, p17) | Affinity, AF7022 |
Anti-cleaved-caspase7 (Asp198) | Affinity, AF4023 |
Anti-cleaved-caspase9 (Asp353) | Affinity, AF5240 |
Real-time quantitative polymerase chain reaction (PCR)
Total RNA was extracted using TRIzol (Thermo Fisher Scientific). The RNA was transcribed using the reverse SYBR Select Master Mix Kit according to the manufacturer’s instructions (Tiangen, Beijing, China), followed by fluorescence quantitative real-time PCR (Yeasen, Shanghai, China). We used Celemetor 96 real-time fluorescence quantitative PCR analysis system for real-time fluorescence quantitative PCR detection (instrument batch number: YS80520-2304-00001; Yeasen). PCR primer sequences were as follows:
- β-actin: AGGCCAACCGTGAAAAGATG (forward primer); AGAGCATAGCCCTCGTAGATGG (reverse primer).
- α-SMA: GCTGGTGATGATGCTCCCA (forward primer); GCCCATTCCAACCATTACTCC (reverse primer).
- Collagen 1a1: CCAAGAAGACATCCCTGAAGTCA (forward primer); TGCACGTCATCGCACACA (reverse primer).
- Fibronectin (FN): GTGTAGCACAACTTCCAATTACGAA (forward primer); GGAATTTCCGCCTCGAGTCT (reverse primer).
Wound-healing assays
For the wound healing assay, Mlg and PPF cells were grown on 6-well plates and scraped to form a 100-µm wound using sterile pipette tips. Because Mlg cells grow quicker, a scratch test was performed when the density of Mlg cells was 50% and the density of Mlg cells was around 100%. The cells were cultured in the presence or absence of pazopanib (2 or 4 µM) in serum-free media for 12, 24, 36, or 48 hours. Images at different time points were captured by a light microscope (Nikon, Tokyo, Japan).
Luciferase assay
A luciferase assay was performed by using a luciferase assay system (Promega, Wisconsin, USA) and a Luminoskan Ascent Reader System (Thermo Fisher Scientific).
Hematoxylin-eosin staining
Hematoxylin-eosin staining was applied after the left lung was sectioned into 5 µm pieces and embedded in paraffin. An upright transmission fluorescence microscope was used to gather the stained images, and Image-Pro Plus Version 6.0 (Media Cybernetics, Inc., Rockville, MD, USA) was used for analysis. By analyzing the entire lung tissue area and automatically calculating the total pixel of the area (Pw), the total pixel of the fibrotic area (Pf) was calculated, and the fibrosis ratio was calculated as Pf/Pw.
Immunohistochemistry
After antigen retrieval, the lung tissue sections were incubated with the primary antibody against the detection marker, the color was developed with Digital Audio Broadcasting (DAB), and the nucleus was stained with hematoxylin. ImageJ software (National Institutes of Health, Maryland, USA) was used to analyze the intensity and percentage of stained cells after microscopic imaging.
Immunofluorescence
Mlg cells were plated on glass slides in 24-well plates and then treated with TGF-β1 (5 ng/mL) or pazopanib (2, 4 µM) for 24 hours. After fixation with 4% paraformaldehyde in phosphate buffer solution (PBS) for 20 minutes, the cells were washed with PBS and incubated with 0.2% Triton X-100 (Sigma) and 5% bovine serum albumin (BSA) (Sigma) for 30 minutes to block nonspecific binding. The primary antibody was incubated on the cells for an entire night at 4 ℃, and the secondary antibody (goat anti-mouse IgG conjugated with DyLight 594) was incubated on the cells for one hour at 37 ℃. Finally, the cells were subjected to nuclear staining with DAPI Staining Solution (Solarbio, Beijing, China). Images were visualized using confocal laser scanning microscopy (Leica SP8, Wetzlar, Germany) and photographed.
Hydroxyproline content
The hydroxyproline concentration in the mouse right lung was determined via the traditional acid hydrolysis method. Briefly, the right lung was immediately dried in an oven and then hydrolyzed at 120 ℃ with 6 N hydrochloric acid for 18 hours. After filtering the residue, 6 N NaOH was used to adjust the pH to 6.5–7.6. The hydroxyproline standard (Sigma) was used to construct a standard curve, and the results were calculated as the µg hydroxyproline/right lung.
Lung function measurement
The lung function of C57BL/6 mice was measured by using an AniRes2005 lung function system (Bestlab, Changsha, China). After anesthesia, the mice were placed in a body drawing box to explore lung function indices, including dynamic compliance (Cydn), inspiratory resistance (Ri), and expiratory resistance (Re), forced vital capacity (FVC), forced expiratory volume in 0.1 second (FEV0.1), and forced expiratory volume in 0.3 second (FEV0.3).
Statistical analysis
The statistical analysis was carried out utilizing GraphPad Prism 9.0. An analysis of significant differences was conducted using a two-tailed Student’s t-test. The concordance was ascertained using linear regression and Pearson correlation. The means ± standard deviations (SDs) were displayed for the data, and the following are the significance levels: P<0.05, P<0.01 and P<0.001.
Results
Pazopanib attenuates BLM-induced pulmonary fibrosis in mice
We first established a BLM-induced pulmonary fibrosis model and evaluated the therapeutic effect of pazopanib. As expected, pazopanib improved BLM-induced alveolar structure distortion and reduced the collagen concentration and percentage of fibrotic areas (Figure 1A,1B). In addition, compared with those in the model group, the hydroxyproline content in the right lung was lower, and the body weight was greater in the pazopanib-treated group (Figure 1C,1D). The effects of pazopanib treatment on pulmonary function parameters are illustrated in Figure 1E-1J, and the results showed that pazopanib significantly reduced the FVC, FEV0.1, FEV0.3, Ri, Re, and Cydn. Nintedanib, a positive control drug, significantly alleviated pulmonary fibrosis in our study, but its effect was worse than that of pazopanib (Figure 1). In conclusion, pazopanib markedly attenuates BLM-induced pulmonary fibrosis mainly by reducing the percentage of fibrosis and improving pulmonary function.
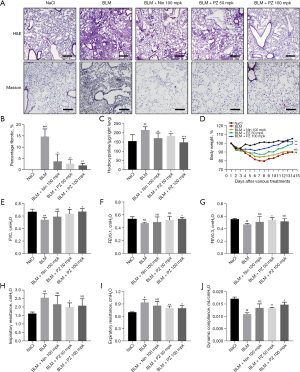
Pazopanib decreased TGF-β1-induced lung fibroblast activation and ECM accumulation in vitro
TGF-β1 is a key profibrotic factor that promotes the progression of pulmonary fibrosis disease and is a major promoter of myofibroblast activation, proliferation and differentiation (22,23). Hence, we evaluated the effect of pazopanib on the TGF-β1-induced activation of lung fibroblasts in vitro. Western blotting analysis revealed that pazopanib treatment downregulated TGF-β1-induced α-SMA, FN and type 1 collagen (Col 1) expression in a dose-dependent manner, which indicated that pazopanib inhibited fibroblast activation and ECM production (Figure 2A,2B). Immunofluorescence staining for α-SMA also confirmed these findings (Figure 2C). In addition, pazopanib reduced the mRNA expression levels of α-SMA, Col 1 and FN in TGF-β1-activated fibroblasts (Figure 2D-2F). In conclusion, pazopanib inhibited TGF-β1-induced lung fibroblast activation and ECM production in vitro.
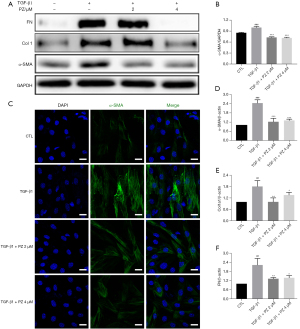
Pazopanib inhibits TGF-β1-induced lung fibroblast migration in vitro
TGF-β1 can induce the migration of human lung fibroblasts, which is strongly related to the progression of pulmonary fibrosis (24). We tested whether pazopanib had an influence on the migration ability of pulmonary fibroblasts by using a wound healing assay. Pulmonary fibroblasts were incubated with pazopanib and/or TGF-β1 for a series of durations. The control group exhibited a narrow cell wound gap, and the pazopanib treatment group exhibited relative delays in wound closure (Figure 3). Hence, pazopanib significantly suppresses the migration of lung fibroblasts.
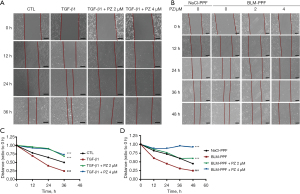
Pazopanib promotes the apoptosis and autophagy of pulmonary myofibroblasts
IPF myofibroblasts exhibit apoptosis resistance both in vivo and in vitro (25). The excessive production of ECM proteins is a major characteristic of IPF, and autophagy controls the turnover of collagen (7). Our previous studies showed that pazopanib treatment can slow the development of pulmonary fibrosis in C57BL/6 mice; therefore, we next explored whether pazopanib had a positive effect on apoptosis and autophagy in lung fibroblasts. Western blotting analysis of pazopanib-treated Mlg cells revealed that the expression levels of cleaved caspase3, cleaved caspase7, and cleaved caspase9 were significantly greater than those in the control group, indicating that pazopanib can promote the apoptosis of lung fibroblasts (Figure 4A). The p62 protein is a cargo essential mediator of autophagy. Bafilomycin a1 (Baf a1) and chloroquine (CQ) are autophagy inhibitors. Our results revealed that pazopanib decreased Baf a1- and CQ-induced p62 expression in a dose-dependent manner (Figure 4B,4C). The GFP-LC3B and mCherry-GFP-LC3B plasmids were transfected into NIH-3T3 cells to measure autophagic flux. As expected, pazopanib increased GFP-LC3B puncta formation and the number of Cherry+ GFP− puncta (autolysosomes) (Figure 4D,4E). Hence, pazopanib attenuates BLM-induced pulmonary fibrosis partly by promoting the apoptosis and autophagy of myofibroblasts.
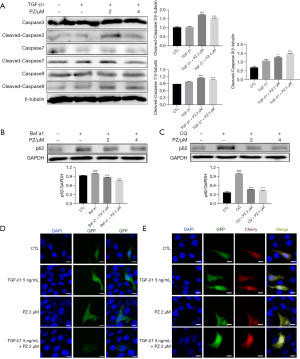
Pazopanib suppresses TGF-β1/Smad and non-Smad signaling
TGF-β1 plays a vital role in the development of pulmonary fibrosis and can upregulate the phosphorylation of Smad2/3. The stable CAGA-NIH-3T3 cell line was constructed in our previous work, and this luciferase reporter system can assess the activation of TGF-β1/Smad3 signaling (26). TGF-β1 promoted Akt and Erk1/2 phosphorylation compared with that in the control group, consistent with previous reports (27,28). Treatment of CAGA-NIH-3T3 cells with pazopanib (0, 0.5, 1, 2, 4, 8, 16, and 32 µM) dose-dependently decreased luciferase activity (Figure 5A). TGF-β1 (5 ng/mL) strongly phosphorylates Smad3 at Ser423/425, so we used this cellular model to determine whether pazopanib had an impact on the TGF-β1/Smad pathway in vitro. As expected, incubating Mlg cells with pazopanib significantly reduced Smad3 and Smad2 phosphorylation (Figure 5B). Mlg cells were incubated with TGF-β1 and/or pazopanib for 12 hours to evaluate whether pazopanib had an impact on TGF-β1/non-Smad (Akt, Erk) signaling. Western blot analysis revealed that pazopanib downregulated the levels of p-Akt (Ser473) and p-Erk1/2 (Thr202/Thr204) (Figure 5C-5E). Next, we verified these results by utilizing PPF cells and found that the results were the same for Mlg cells. In NaCl-PPF and BLM-PPF cells, the levels of Akt and Erk and their phosphorylation were reduced after pazopanib treatment for 24 hours (Figure 5F). These results showed that pazopanib suppresses TGF-β1/Smad and non-Smad signaling in vitro.
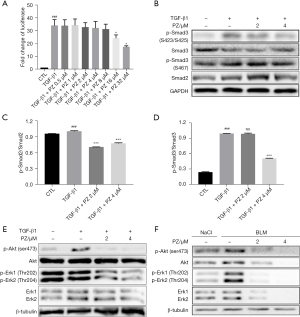
Pazopanib inhibits fibroblast activation and ECM accumulation and promotes autophagy in vivo
We further explored whether pazopanib had similar effects in vivo. Mice were orally treated with pazopanib (50, 100 mg/kg) on days 7–14 after BLM administration, and the control group was treated with saline. To test the effect of pazopanib treatment in vivo, we conducted western blotting to determine the expression and activity levels of important proteins in lung tissues. As shown in Figure 6A, pazopanib significantly decreased the expression of α-SMA, Col1, FN, p-Smad3 and p62 in lung tissues. The results of immunohistochemical staining also confirmed that pazopanib inhibited the activation of lung fibroblasts, the deposition of collagen, the expression of p62 and the signaling of TGF-β1/Smad3 in a dose-dependent manner (Figure 6B). On the basis of the above studies, pazopanib exerted this effect at a much lower concentration than pirfenidone in lung tissues, demonstrating that it has stronger inhibitory effects on fibroblast activation, ECM production, the expression of p62 and the signaling pathway of TGF-β1/Smad3 (Figure 6). Taken together, these findings suggest that pazopanib attenuates BLM-induced pulmonary fibrosis by suppressing the TGF-β1/Smad3 pathway in vivo.
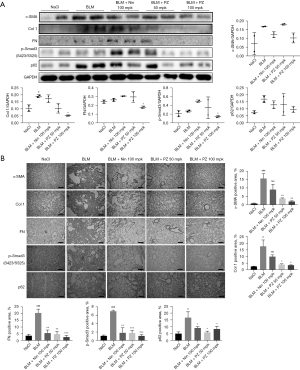
Discussion
ECM accumulation is a fatal characteristic in IPF patients and can lead to irreparable scarring in the lung. It is widely believed that the ECM is secreted mainly by activated fibroblasts and that this accumulation is difficult to reverse entirely in the present study (29). When lung tissues are stimulated continually by chemical materials, fibroblasts can sustain high activation and an uncontrolled state to repair injured lung tissues (1). Obviously, unrepaired scars decrease FVC in IPF patients and eventually lead to death from respiratory failure. Nintedanib is currently the only drug approved for the treatment of IPF. As a tyrosine kinase inhibitor, nintedanib can inhibit a variety of receptor protein tyrosine kinases (PTKs) and non-receptor PTKs (nPTKs), comprising the receptors for fibroblast growth factor, platelet-derived growth factor, and vascular endothelial growth factor (VEGF). Platelet-derived growth factor receptor (PDGFR), fibroblast growth factor receptor (FGFR) and VEGF receptor (VEGFR) are related to the pathogenesis of IPF. Nintedanib can competitively bind to adenosine triphosphate of these receptors and block intracellular signals related to the proliferation, migration and transformation of fibroblasts related to the pathological mechanism of IPF. Pazopanib is also a small molecule inhibitor of multiple protein tyrosine kinases, including vascular endothelial growth factor receptor and platelet-derived growth factor receptor. It is approved for treating patients suffered from renal cell cancer and advanced soft tissue sarcoma (30). It participates in the inhibition of signal pathway, angiogenesis and cell proliferation.
Our findings showed that pazopanib inhibited myofibroblast activation and ECM production in vitro, the same effect was also produced in vivo experiments in mice. Pazopanib also promoted mouse lung function in a BLM-induced pulmonary fibrosis model. This finding suggested that the mechanism through which pazopanib improved lung functions was mainly through the inhibition of ECM production. These in vitro and in vivo results provide an efficient method to support the therapeutic benefits of pazopanib in fibrotic progression.
Many studies have shown that autophagy plays a key role in the pathogenesis of pulmonary fibrosis. The expression level of the autophagy protein p62 in the lung tissue of IPF patients is greater than that in normal individuals (31). When autophagy is inhibited, AICAR (an AMPK activator) is unable to reduce the steady-state levels of collagen induced by TGF-β1 (7). Moreover, the inhibition of autophagic flux induced by profibrotic cytokines can reduce the production of collagen and alleviate pulmonary fibrosis (32). In animal models of BLM-induced pulmonary fibrosis, BLM blocks TFEB-induced autophagic flux by binding to the target ANXA2 (33). In addition, autophagic flux is blocked in alveolar epithelial cells in silica-induced pulmonary fibrosis (34). In summary, autophagy plays an essential role in fibrosis progression because this kind of biological function might promote the degradation of collagen. In our study, pazopanib decreased p62 expression and increased autophagic flux. p62 is a key factor in the regulation of autophagy, mainly through interaction with LC3 (35). However, whether the specific mechanism by which pazopanib reduces p62 expression is by influencing its interaction with LC3 needs to be further investigated.
TGF-β1 is a key regulator of the progression of organ fibrosis. Many studies have shown that the binding of TGF-β to its receptor activates Smad2 and Smad3 to regulate the expression of ECM genes and pulmonary fibrogenesis (36). In addition, TGF-β activates Akt in glomerular mesangial cells by inducing both miR-216a and miR-21 (24). TGF-β also activates Erk MAP kinases mainly by activating the TGF-β type I receptor to recruit and directly phosphorylate ShcA proteins on tyrosine and serine residues (28). Therefore, the inhibition of the TGF-β/Smad and non-Smad (Erk, Akt) signaling pathways efficiently suppresses myofibroblast activation and ECM production to attenuate pulmonary fibrosis. Our results showed that pazopanib inhibited fibroblast activation and ECM deposition mainly by inhibiting the TGF-β/Smad and non-Smad signaling pathways in vitro and in vivo, thus alleviating pulmonary fibrosis.
Conclusions
In conclusion, our data demonstrated that pazopanib attenuated BLM-induced pulmonary fibrosis in mice. We further explored the pharmacological mechanism of pazopanib, and the results indicated that pazopanib can suppress myofibroblast activation, migration, autophagy, apoptosis and ECM accumulation by downregulating TGF-β1/Smad signaling and the TGF-β1/non-Smad pathway. Pazopanib is a small molecule that is highly active in cancer treatment and might have an equal effect on improving fibrosis progression to benefit IPF patients.
Acknowledgments
Funding: This work was supported by
Footnote
Reporting Checklist: The authors have completed the ARRIVE reporting checklist. Available at https://jtd.amegroups.com/article/view/10.21037/jtd-23-1349/rc
Data Sharing Statement: Available at https://jtd.amegroups.com/article/view/10.21037/jtd-23-1349/dss
Peer Review File: Available at https://jtd.amegroups.com/article/view/10.21037/jtd-23-1349/prf
Conflicts of Interest: All authors have completed the ICMJE uniform disclosure form (available at https://jtd.amegroups.com/article/view/10.21037/jtd-23-1349/coif). H.R., C.G., Z.W., L.T., S.G., H.Z., and H.Y. are employees of China Resources Biopharmaceutical Co., Ltd. The other authors have no conflicts of interest to declare.
Ethical Statement: The authors are accountable for all aspects of the work in ensuring that questions related to the accuracy or integrity of any part of the work are appropriately investigated and resolved. All animal care and experimental procedures were approved by the IACUC of Nankai University (project code: SCXK 2019-0001, date of approval: 14 January 2019; Approval No. SYXK 2014-0003), in compliance with institutional guidelines for the care and use of animals.
Open Access Statement: This is an Open Access article distributed in accordance with the Creative Commons Attribution-NonCommercial-NoDerivs 4.0 International License (CC BY-NC-ND 4.0), which permits the non-commercial replication and distribution of the article with the strict proviso that no changes or edits are made and the original work is properly cited (including links to both the formal publication through the relevant DOI and the license). See: https://creativecommons.org/licenses/by-nc-nd/4.0/.
References
- Wolters PJ, Collard HR, Jones KD. Pathogenesis of idiopathic pulmonary fibrosis. Annu Rev Pathol 2014;9:157-79. [Crossref] [PubMed]
- Mora AL, Rojas M, Pardo A, et al. Emerging therapies for idiopathic pulmonary fibrosis, a progressive age-related disease. Nat Rev Drug Discov 2017;16:755-72. [Crossref] [PubMed]
- Chanda D, Otoupalova E, Smith SR, et al. Developmental pathways in the pathogenesis of lung fibrosis. Mol Aspects Med 2019;65:56-69. [Crossref] [PubMed]
- Huang H. Update in interstitial lung disease 2023. Zhonghua Jie He He Hu Xi Za Zhi 2024;47:44-9. [PubMed]
- Johannson KA, Vittinghoff E, Lee K, et al. Acute exacerbation of idiopathic pulmonary fibrosis associated with air pollution exposure. Eur Respir J 2014;43:1124-31. [Crossref] [PubMed]
- Karimi-Shah BA, Chowdhury BA. Forced vital capacity in idiopathic pulmonary fibrosis--FDA review of pirfenidone and nintedanib. N Engl J Med 2015;372:1189-91. [Crossref] [PubMed]
- Rangarajan S, Bone NB, Zmijewska AA, et al. Metformin reverses established lung fibrosis in a bleomycin model. Nat Med 2018;24:1121-7. [Crossref] [PubMed]
- Ask K, Bonniaud P, Maass K, et al. Progressive pulmonary fibrosis is mediated by TGF-beta isoform 1 but not TGF-beta3. Int J Biochem Cell Biol 2008;40:484-95. [Crossref] [PubMed]
- Leppäranta O, Sens C, Salmenkivi K, et al. Regulation of TGF-β storage and activation in the human idiopathic pulmonary fibrosis lung. Cell Tissue Res 2012;348:491-503. [Crossref] [PubMed]
- Wipff PJ, Rifkin DB, Meister JJ, et al. Myofibroblast contraction activates latent TGF-beta1 from the extracellular matrix. J Cell Biol 2007;179:1311-23. [Crossref] [PubMed]
- Liu H, Sun M, Wu N, et al. TGF-β/Smads signaling pathway, Hippo-YAP/TAZ signaling pathway, and VEGF: Their mechanisms and roles in vascular remodeling related diseases. Immun Inflamm Dis 2023;11:e1060. [Crossref] [PubMed]
- Schiller M, Javelaud D, Mauviel A. TGF-beta-induced SMAD signaling and gene regulation: consequences for extracellular matrix remodeling and wound healing. J Dermatol Sci 2004;35:83-92. [Crossref] [PubMed]
- Levine B, Kroemer G. Autophagy in the pathogenesis of disease. Cell 2008;132:27-42. [Crossref] [PubMed]
- Lock R, Debnath J. Extracellular matrix regulation of autophagy. Curr Opin Cell Biol 2008;20:583-8. [Crossref] [PubMed]
- Avivar-Valderas A, Bobrovnikova-Marjon E, Alan Diehl J, et al. Regulation of autophagy during ECM detachment is linked to a selective inhibition of mTORC1 by PERK. Oncogene 2013;32:4932-40. [Crossref] [PubMed]
- Zhang HY, Phan SH. Inhibition of myofibroblast apoptosis by transforming growth factor beta(1). Am J Respir Cell Mol Biol 1999;21:658-65. [Crossref] [PubMed]
- Pick AM, Nystrom KK. Pazopanib for the treatment of metastatic renal cell carcinoma. Clin Ther 2012;34:511-20. [Crossref] [PubMed]
- Climent MA, Muñoz-Langa J, Basterretxea-Badiola L, et al. Systematic review and survival meta-analysis of real world evidence on first-line pazopanib for metastatic renal cell carcinoma. Crit Rev Oncol Hematol 2018;121:45-50. [Crossref] [PubMed]
- Martin-Broto J, Stacchiotti S, Lopez-Pousa A, et al. Pazopanib for treatment of advanced malignant and dedifferentiated solitary fibrous tumour: a multicentre, single-arm, phase 2 trial. Lancet Oncol 2019;20:134-44. [Crossref] [PubMed]
- Tavallai S, Hamed HA, Grant S, et al. Pazopanib and HDAC inhibitors interact to kill sarcoma cells. Cancer Biol Ther 2014;15:578-85. [Crossref] [PubMed]
- Olaussen KA, Commo F, Tailler M, et al. Synergistic proapoptotic effects of the two tyrosine kinase inhibitors pazopanib and lapatinib on multiple carcinoma cell lines. Oncogene 2009;28:4249-60. [Crossref] [PubMed]
- Kennedy WJ, Trejdosiewicz LK, Southgate J. The role of the extracellular matrix, TGF beta and beta 1-integrin in the regulation of normal human urothelial cytodifferentiation. British Journal of Cancer 1998;78:45.
- Wells RG, Discher DE. Matrix elasticity, cytoskeletal tension, and TGF-beta: the insoluble and soluble meet. Sci Signal 2008;1:pe13. [Crossref] [PubMed]
- Fang LP, Lin Q, Tang CS, et al. Hydrogen sulfide suppresses migration, proliferation and myofibroblast transdifferentiation of human lung fibroblasts. Pulm Pharmacol Ther 2009;22:554-61. [Crossref] [PubMed]
- Thannickal VJ, Horowitz JC. Evolving concepts of apoptosis in idiopathic pulmonary fibrosis. Proc Am Thorac Soc 2006;3:350-6. [Crossref] [PubMed]
- Wang Q, Xiong F, Wu G, et al. SMAD Proteins in TGF-β Signalling Pathway in Cancer: Regulatory Mechanisms and Clinical Applications. Diagnostics (Basel) 2023;13:2769. [Crossref] [PubMed]
- Kato M, Putta S, Wang M, et al. TGF-beta activates Akt kinase through a microRNA-dependent amplifying circuit targeting PTEN. Nat Cell Biol 2009;11:881-9. [Crossref] [PubMed]
- Lee MK, Pardoux C, Hall MC, et al. TGF-beta activates Erk MAP kinase signalling through direct phosphorylation of ShcA. EMBO J 2007;26:3957-67. [Crossref] [PubMed]
- Sun W, Tang H, Gao L, et al. Mechanisms of pulmonary fibrosis induced by core fucosylation in pericytes. Int J Biochem Cell Biol 2017;88:44-54. [Crossref] [PubMed]
- Miyamoto S, Kakutani S, Sato Y, et al. Drug review: Pazopanib. Jpn J Clin Oncol 2018;48:503-13. [Crossref] [PubMed]
- Yue YL, Zhang MY, Liu JY, et al. The role of autophagy in idiopathic pulmonary fibrosis: from mechanisms to therapies. Ther Adv Respir Dis 2022;16:17534666221140972. [Crossref] [PubMed]
- Mi S, Li Z, Yang HZ, et al. Blocking IL-17A promotes the resolution of pulmonary inflammation and fibrosis via TGF-beta1-dependent and -independent mechanisms. J Immunol 2011;187:3003-14. [Crossref] [PubMed]
- Wang K, Zhang T, Lei Y, et al. Identification of ANXA2 (annexin A2) as a specific bleomycin target to induce pulmonary fibrosis by impeding TFEB-mediated autophagic flux. Autophagy 2018;14:269-82. [Crossref] [PubMed]
- Zhao X, Wei S, Li Z, et al. Autophagic flux blockage in alveolar epithelial cells is essential in silica nanoparticle-induced pulmonary fibrosis. Cell Death Dis 2019;10:127. [Crossref] [PubMed]
- Katsuragi Y, Ichimura Y, Komatsu M. p62/SQSTM1 functions as a signaling hub and an autophagy adaptor. FEBS J 2015;282:4672-8. [Crossref] [PubMed]
- Hu HH, Chen DQ, Wang YN, et al. New insights into TGF-β/Smad signaling in tissue fibrosis. Chem Biol Interact 2018;292:76-83. [Crossref] [PubMed]