Clinical outcomes of radiofrequency catheter ablation guided by intracardiac echocardiography for Chinese atrial fibrillation patients: a single-center, retrospective study
Highlight box
Key findings
• Intracardiac echocardiography (ICE) significantly reduced the fluoroscopic time for radiofrequency catheter ablation in Chinese atrial fibrillation (AF) patients without compromising on safety and effectiveness.
What is known and what is new?
• ICE with real-time imaging and real-time monitoring of intraoperative complications has a number of advantages in electrophysiological applications.
• Given that the research on ICE-guided radiofrequency ablation in China is scanty, we conducted a retrospective study which found that ICE significantly reduced the fluoroscopic time for radiofrequency catheter ablation in Chinese AF patients without compromising on safety and effectiveness.
What is the implication, and what should change now?
• The use of ICE in radiofrequency ablation for AF could reduce or even avoid the radiation hazards posed by fluoroscopy to patients and physicians without compromising the safety and effectiveness of the procedures.
• Larger sample size and longer follow-up study should be conducted in the future.
Introduction
Atrial fibrillation (AF) is one of the most common clinical atrial arrhythmias and a major public health problem affecting human health worldwide. The AF or atrial flutter (AFL) affects patients’ quality of life and increases susceptibility to severe complications, such as myocardial infarction, heart failure (HF), strokes and ultimately death (1). The AF or AFL remains one of the leading causes of death with estimated 287,241 deaths worldwide in 2017. In China, a latest epidemiological survey found that 1.6% of adult population, about 20 million patients suffer from AF (2). Clinical management of AF has been continuously evolving and catheter ablation has become one of the most effective therapies for many patients with symptomatic AF in recent years and was also recommended by the current clinical guidelines in both Europe and China (3,4).
Intracardiac echocardiography (ICE) shows some advantages in treatment of AF and thrombus screening. As a new technology, ICE can not only provide excellent imaging of various anatomical structures in the heart, but also guide the examination of AF thrombus combined with a three-dimensional (3D) electroanatomical mapping system, such as the Carto system, guide the placement of coronary sinus (CS) electrodes, provide direct visualization for the atrial septal puncture, and generate 3D model of the atrium during the operation of the catheter (5). That is, ICE could provide real-time, comprehensive, clear, and precise visualization of intricate anatomical relationships within the heart chambers of interest during procedure to support the assessment of catheter-tissue contact, enhancing the precision and efficacy of procedures (6). ICE also enables real-time monitoring of potential complications during the procedure, such as avoiding the esophagus or aortic penetration, and promptly detecting pericardial effusion or thrombus formation, thus enhancing procedural safety. During the procedure, ICE has advantages in reducing fluoroscopy exposure which could reduce the risk of disease for patients and operators due to exposure to fluoroscopy. In addition, ICE may replace traditional transesophageal echocardiography (TEE) for screening left atrial appendage thrombus in patients who are reluctant to receive or intolerant to TEE (7). Anter et al. reported that ICE demonstrated a higher achievement rate for diagnostic imaging of the left atrial appendage compared to TEE (100% vs. 87.3%, P<0.002) (8).
In recent years, radiofrequency ablation under the guidance of ICE has been introduced in clinical practice in China. A number of studies have reported that ICE is as effective and safe as traditional fluoroscopy and also effectively reduces the time of fluoroscopy exposure. Lin et al. compared the use of ICE and traditional fluoroscopy for atrial septal puncture in radiofrequency ablation in 25 AF patients (9). The results indicated that atrial punctures in both groups had high acute success rates (93.3% vs. 100%, P=0.06). As expected, the use of ICE significantly reduced fluoroscopy time but was associated with longer operation time (10).
While ICE-guided AF ablation has been widely performed in United States and European countries, its application in China is still at infant stage. To date, research on ICE-guided radiofrequency ablation in China has been scanty. To supplement clinical evidence for ICE applications and to provide evidence support for innovative procedural approaches in clinical practice, we conducted this retrospective study at one tertiary hospital in China to evaluate the total fluoroscopy time and dose, safety, and effectiveness of AF ablation guided by ICE in routine clinical practice and compared the findings with those underwent conventional fluoroscopy-guided (non-ICE) AF ablation. We present this article in accordance with the STROBE reporting checklist (available at https://jtd.amegroups.com/article/view/10.21037/jtd-23-1418/rc).
Methods
Study design and study population
We conducted a single-center retrospective study in The First Affiliated Hospital, Zhejiang University School of Medicine in China, which is one of the earliest institutes adopting ICE technology for cardiac interventions. The patients with AF who were admitted to the hospital from June 1, 2020 to May 31, 2022 and underwent Smart Touch® (ST; Biosense Webster, Diamond Bar, CA, USA) radiofrequency catheter ablation guided by ICE or non-ICE (conventional fluoroscopy-guided) were included. Patients with prior procedural history of AF ablation or cardiovascular events within three months before ablation, including acute myocardial infarction, percutaneous coronary intervention, heart valve surgery, or coronary artery bypass surgery, were excluded. This study was performed in accordance with the principle of the Declaration of Helsinki (as revised in 2013). Approval was granted by the Ethics Committee of The First Affiliated Hospital, Zhejiang University School of Medicine (No. IIT2020688). Individual consent for this retrospective analysis was waived.
Data extraction
Baseline and treatment-related data of patients were obtained from the electronic medical record system and the fluoroscopy time and dose were collected from digital subtraction angiography machine of The First Affiliated Hospital, School of Medicine, Zhejiang University. The collected data included age, sex, type of AF and presence of comorbidities including AF, coronary heart disease (CHD), HF, diabetes, CHA2DS2-VASc score (Congestive heart failure, Hypertension, Age ≥75 years, Diabetes mellitus, previous Stroke/transient ischaemic attack, Vascular disease, Age 65–74 years, Sex category), and HAS-BLED (Hypertension, Abnormal renal/liver function, Stroke, Bleeding history or predisposition, Labile international normalized ratio, Elderly, Drugs/alcohol concomitantly) score. Treatment-related data were collected during hospitalization, including type of surgery, radiofrequency catheter ablation procedure information, ablation strategy used in surgery, and ablation index (AI) software parameters. Follow-up data include clinical visit date and AF recurrence.
Preoperative examination
Patients without preoperative contraindications routinely received the following evaluation: electrocardiogram, dynamic electrocardiogram, echocardiography and coronary computed tomography angiography.
Procedure
ICE-guided atrial transseptal puncture
In the ICE group, ICE catheter (Carto Sound; Biosense Webster) was placed to the right atrium from femoral vein to construct a left atrial model. ICE confirmed that two long guidewires were delivered to the superior vena cava (SVC) via the femoral vein. The Swartz sheath (SL1; Abbott, Abbott Park, IL, USA) was delivered into the SVC along a long guidewire. After ultrasound confirmation, the guidewire was removed and the atrial septal puncture needle was placed. The SL1 was pulled downed to sheath to the fossa ovalis (FO) with the guidance of ICE. The needle punctuated the FO, and saline was injected to confirm the puncture was successful. Then the SL1 sheath and needle were advanced synchronously, afterwards the needle was withdrawn, and a guidewire was delivered through the SL1 sheath to the left superior pulmonary vein (LSPV). The SL1 sheath was placed to the left atrium through the guidewire, and then retracted to the right atrium. The ultrasound was manipulated so that the junction points of the guidewire entering the left atrium and the FO was stably displayed and marked on the 3D model. The steerable sheath (Mobicath or Vizigo; Biosense Webster) was delivered into right atrium through the other guidewire. The ST catheter entered right atrium through the sheath. Under 3D (Carto3; Biosense Webster) visualization, we manipulated the catheter to look for the marked atrial septal puncture point, through the point ST catheter entered the left atrium to the LSPV. Then, the SL1 sheath was placed to the left atrium through the first guidewire, and the steerable sheath was also placed to the left atrium through the ST catheter. The mapping catheter (Lasso Sas or Pentaray; Biosense Webster) entered left atrium through the SL1 sheath.
ICE combined with total 3D (T3D)-guided atrial transseptal puncture
In the study, we evaluated the clinical outcomes of AF ablation with combination of T3D imaging with ICE to perform transseptal puncture, introducing a novel procedural approach. The procedure comprised nine steps, and each step is shown in Figures 1-8. The detailed description of each step is as follows.
- Step 1: modeling of the right atrium (including the CS) with an ST catheter under ultrasound guidance (Figure 1).
- Step 2: under the guidance of ultrasound, use the ST catheter to jump into the FO and mark the FO (Figure 2).
- Step 3: placing the CS catheter into the CS on the constructed 3D geometry (Figure 3).
- Step 4: inserting the SL1 sheath into the SVC under ultrasound guidance and confirmed its position within the vessel by injecting a small amount of saline through the sheath. The figure of step 4 could referenced in Yu et al.’s study (11). Subsequently, the guidewire was replaced with the transseptal puncture needle, featuring a clip attached to its distal end. Before retracting the SL1 sheath from the SVC the puncture needle’s tip was slightly protruded from the sheath (1 mm), visualized on the 3D system to observe its movement from the SVC to the FO on the 3D model.
- Step 5: through ultrasound guidance and 3D imaging, the puncture needle is sent into the FO area (Figure 4).
- Step 6: under the guidance of ultrasound, use a puncture needle to pass through the FO (Figure 5).
- Step 7: under the guidance of ultrasound, insert the guidewire into the LSPV (Figure 6).
- Step 8: recording the trajectory of the guidewire on the 3D geometry (Figure 7).
- Step 9: passing through the FO along the track of the guidewire and send the ST catheter into the left atrium (Figure 8).
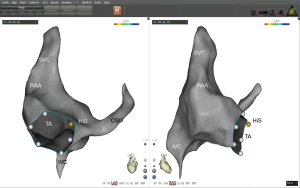
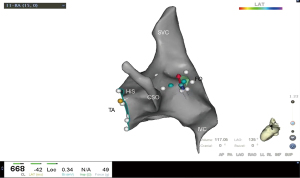
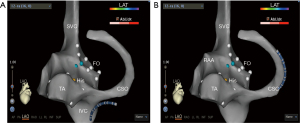
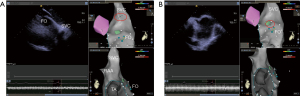
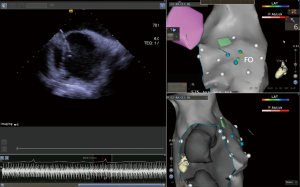
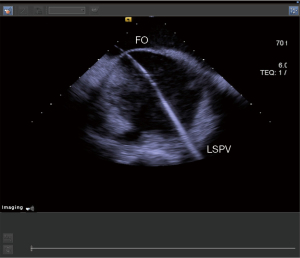
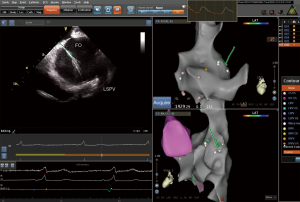
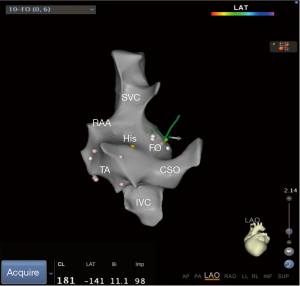
Non-ICE guided atrial transseptal puncture
In the non-ICE group, all transseptal puncture steps were performed under X-ray guidance. In brief, the procedure was performed in following steps: advancing the guidewire and SL1 sheath into the SVC followed by inserting the transseptal puncture needle into the SL1 sheath; withdrawing the sheath and needle to the position of dropping into the FO in anterior-posterior view, confirming anterior-posterior positioning in right anterior oblique view before advancing the needle through the FO, injecting contrast agent in left anterior oblique view to confirm needle entry into the left atrium, retracting the puncture needle, advancing the guidewire into the left SPV and subsequently advancing the SL1 sheath into the left atrium.
AI-guided radiofrequency catheter ablation
Both groups of patients underwent radiofrequency catheter ablation using ST catheter under the guidance of AI with the following parameters: anterior wall of the left atrium 500, posterior wall of the left atrium 400, inferior wall 500, superior wall 500, and ridge 550. Power was set to 35 W under ablation power mode, the temperature of catheter tip was ≤43°. The cold saline perfusion flow rate was 20 mL/h during radiofrequency ablation. The endpoint of ablation was bilateral pulmonary vein isolation. For patients with AFL, activation mapping and ablation were performed. For patients with typical AFL before surgery, additional tricuspid valve ablation was performed with the AI setting of 450 for the cavotricuspid ablation and the end point was isthmic bidirectional block. In the ICE group, ICE is utilized to monitor the procedural complications such as pericardial effusion.
Postoperative follow-up
Follow-up was conducted by assessing relative medical chart data up to 6 months (±14 days) following the index date of the procedure, cases such as refusal or mortality were defined as loss to follow-up. No additional data imputation will be performed for those lost to follow-up. Standard of care during the follow-up period included continued use of anticoagulants (warfarin or novel oral anticoagulants) and anti-arrhythmic drugs for three months after ablation in all patients. Whether anticoagulants were discontinued after 3 months was assessed according to the CHA2DS2-VASc score and the stroke risk for the patients. Electrocardiogram or Holter monitoring record were obtained at the 6-month follow-up for confirming recurrence.
Outcomes measurement
During the operation, the patient was considered having acute surgery failure if the procedure failed to achieve all pulmonary vein afferent block. Major complications were defined as cardiac effusion or tamponade, high-grade atrioventricular block, cardiac decompensation, cardiogenic shock, femoral pseudoaneurysm, arterial-venous fistula, or inguinal hemorrhage requiring intervention. Total procedure time was defined from the time of groin puncture until removal of all venous sheaths. Transseptal puncture time was defined as the time from when the long sheath was inserted into the SVC to its insertion into the left atrium. Ablation time was defined as the duration of radiofrequency energy delivery. Six-month surgery success was defined as freedom of AF recurrence and AFL lasting >30 seconds after a 3-month blanking period within 6 months (±14 days) post the procedure.
Statistical analysis
Data analysis was performed using R statistical software (Version 4.2.1). The continuous variables of normal distribution were expressed as mean ± standard deviation and Student’s t-test was used for comparison between variables. The continuous variables of non-normal distribution were expressed as median (the 5th percentile, the 95th percentile), and the comparison between variables was performed using nonparametric test (Kruskal-Wallis rank-sum test). Discrete variables were expressed as ratios and Chi-squared test or Fisher’s exact test was used as appropriate. P<0.05 was considered statistically significant.
Results
Baseline characteristic
A total of 97 patients were identified in the study, of which 48 patients were categorized into ICE group and 49 patients into non-ICE group. Of them, 60.8% had paroxysmal AF and 39.2% had persistent AF. The baseline demographic characteristics and clinical profiles were comparable between the two groups (Table 1). The mean age of patients in ICE group was 62.3±10.9 years old and 70.8% of them were males. In non-ICE group, the mean age of patients was 60.3±9.3 years old and 69.4% of them were males. There were 52.1% and 53.1% with hypertension, 2.1% and 8.2% patients with HF, 6.2% and 18.4% patients with diabetes in ICE group and non-ICE group, respectively. While 16.7% patients in ICE group with high-risk of stroke (CHA2DS2-VASc scores ≥3), 22.4% patients in non-ICE group with high risk of stroke. Similarly, 6.2% patients in ICE group with a high risk of major bleeding (HAS-BLED score ≥3) and the percentage of patients with high major bleeding risk in non-ICE group was 12.2%.
Table 1
Characteristics | Non-ICE (n=49) | ICE (n=48) | P value |
---|---|---|---|
Age (years) | 60.31±9.28 | 62.31±10.87 | 0.33 |
Male | 34 (69.4) | 34 (70.8) | >0.99 |
Type of AF | |||
Persistent AF | 16 (32.7) | 22 (45.8) | 0.26 |
Paroxysmal AF | 33 (67.3) | 26 (54.2) | |
BMI (kg/m2) | 24.16±3.10 | 24.05±3.34 | 0.87 |
Prior antiarrhythmic drug use | 26 (53.1) | 22 (45.8) | 0.61 |
Hypertension | 26 (53.1) | 25 (52.1) | >0.99 |
Heart failure | 4 (8.2) | 1 (2.1) | 0.37 |
Diabetes | 9 (18.4) | 3 (6.2) | 0.13 |
Previous stroke/TIA | 2 (4.1) | 0 (0.0) | 0.48 |
CHD | 6 (12.2) | 2 (4.2) | 0.28 |
Atrial flutter | 4 (8.2) | 1 (2.1) | 0.37 |
Atrial tachycardia | 4 (8.2) | 6 (12.5) | 0.71 |
Atrial premature beats | 15 (30.6) | 11 (22.9) | 0.53 |
LVEF (%) | 62.84±9.87 | 63.15±6.00 | 0.86 |
CHA2DS2-VASc score | 0.64 | ||
<3 | 38 (77.6) | 40 (83.3) | |
≥3 | 11 (22.4) | 8 (16.7) | |
HAS-BLED score | 0.50 | ||
<3 | 43 (87.8) | 45 (93.8) | |
≥3 | 6 (12.2) | 3 (6.2) |
Data are presented as mean ± standard deviation or n (%). ICE, intracardiac echocardiography; AF, atrial fibrillation; BMI, body mass index; TIA, transient ischemic attack; CHD, coronary heart disease; LVEF, left ventricular ejection fraction; CHA2DS2-VASc, Congestive heart failure, Hypertension, Age ≥75 years, Diabetes mellitus, previous Stroke/transient ischaemic attack, Vascular disease, Age 65–74 years, Sex category; HAS-BLED, Hypertension, Abnormal renal/liver function, Stroke, Bleeding history or predisposition, Labile international normalized ratio, Elderly, Drugs/alcohol concomitantly.
Procedure characteristics
Total fluoroscopy time and dose were significantly lower in ICE group in contrast to non-ICE group (0.0±0.0 vs. 9.7±4.9 min, P<0.001; 0.0±0.0 vs. 77.1±44.3 mGy/cm2, P<0.001, respectively). Transseptal puncture left atrial modelling and AI-guided radiofrequency ablation were achieved in all patients in ICE group. None of patients experienced acute surgery failure with no major procedure-related complications occurred in either group. The average total procedure time in ICE group was about 12 min longer compared to non-ICE groups (132.1±37.6 vs. 120.5±33.6 min) but the difference was not statistically significant (P=0.11). Although the transseptal puncture time (7.5±5.2 vs. 8.9±8.0 min, P=0.30) and ablation time (29.8±9.0 vs. 28.6±9.3 min, P=0.53) were quite similar between the two groups. Procedure characteristics are summarized in Table 2.
Table 2
Characteristics | Non-ICE (n=49) | ICE (n=48) | P value |
---|---|---|---|
First pass isolation | |||
Left | 28 (57.1) | 30 (62.5) | 0.74 |
Right | 36 (73.5) | 38 (79.2) | 0.67 |
Acute failure rate | 0 (0.0) | 0 (0.0) | NA |
Fluoroscopy exposure (min) | 9.7±4.9 | 0.0±0.0 | <0.001 |
Fluoroscopy dose (mGy/cm2) | 77.1±44.3 | 0.0±0.0 | <0.001 |
Total procedure time (min) | 120.5±33.6 | 132.1±37.6 | 0.11 |
Transseptal puncture (min) | 8.9±8.0 | 7.5±5.2 | 0.30 |
Ablation time (min) | 28.6±9.3 | 29.8±9.0 | 0.53 |
Major complication | 0 (0.0) | 0 (0.0) | NA |
Data are presented as mean ± standard deviation or n (%). ICE, intracardiac echocardiography; NA, not applicable.
Follow-up
Of out of the 97 patients analyzed, 6-month follow-up data were available for 60 patients. There were 33.3% of patients (26.1% and 40.9% in ICE and non-ICE group, respectively) lost to follow-up. There were two AF recurrences in each group during the 6-month follow-up, which was not statistically significant (P>0.99). One patient in ICE group was readmitted for AF recurrence and two patients were readmitted for non-AF related events in each group.
Subgroup analysis by AF diagnosis
Different types of AF may be associated with the clinical outcomes. Therefore, we conducted subgroup analyses to address potential sources of bias. For patients diagnosed with persistent AF, transseptal puncture time and ablation time were similar between ICE and non-ICE groups. The average total procedure time was 17 min longer in ICE group but the difference was not statistically significant (147.3±45.4 vs. 130.2±27.7 min, P=0.18). The transseptal puncture time, ablation time, and total procedure time were all comparable between ICE and non-ICE groups. There was no significant difference noted with respect to 6-month surgery success between the two groups by AF diagnosis.
Discussion
In this retrospective cohort study, we investigated fluoroscopy time and dose during the procedure as well as clinical outcomes of AF patients treated with radiofrequency ablation guided by either conventional fluoroscopy or ICE. We found that the ICE-guided radiofrequency ablation significantly reduced fluoroscopy time and dose and even achieved zero fluoroscopy. The incidence of complications was low among both groups with no major procedure-related complications observed in either group. This implies that the use of ICE in radiofrequency ablation for AF could reduce or even avoid the radiation hazards posed by fluoroscopy to patients and physicians, as well as ensuring the safety and effectiveness of the procedures. The transseptal puncture has become one of the significant techniques for interventional cardiac procedures such as catheter ablation. Although the success rate of conventional transseptal puncture is high, there are limitations to this approach. For patients with normal cardiac anatomy, the transseptal puncture without using ICE as guidance is safe and effective. However, for patients with anatomical variants, there is an increased risk of puncture failure and procedure-related complications, such as, cardiac tamponade and aortic root puncture (12). The use of ICE as guidance for transseptal puncture can make the puncture process easier, safer and more specifically directed into the FO. Aldhoon et al. reported perioperative cardiac tamponade rate of only 0.25% following AF radiofrequency ablation guided with ICE (13). Žižek et al. investigated patients with left-sided tachycardia treated with zero-radiation septal puncture and showed that only 0.2% of patients failed transept al puncture under ICE guidance with an overall complication rate of 1.9% (14). In our study, all patients in the ICE group who underwent the intervention of two sheaths in one puncture did not experience major intraoperative complications, confirming its feasibility and safety.
Our study findings showed that performing transseptal puncture under ICE guidance can achieve zero-fluoroscopy, which is beneficial for both the operators and the patients. The exposure to ionizing radiation leading to complications such as skin damage, cancer, radiation-induced cataracts, and genetic mutations has remained a challenge in clinical practice. The use of ICE enables fluoroscopy-free procedures, avoiding prolonged exposure of procedural operators to radiation and offering a safer option for patients, especially for pregnant women and children (15). Moreover, it helps prevent interventional medical staff from experiencing occupational musculoskeletal conditions associated with wearing the radiation blocking equipment, thereby reducing problems like neck and back pain, severe disc herniation, ultimately enhancing the physical and mental well-being of healthcare professionals and extending their careers (16).
ICE technology’s real-time imaging, continual ablation catheter tracking, and intraoperative complication monitoring significantly enhance usability, especially for beginners (15). Study by Zei et al. highlights that employing an ICE-based approach in AF catheter ablation substantially reduces the learning curve for beginners (17). T3D technology guided transseptal puncture was a novel transseptal puncture procedure technique without fluoroscopy or ultrasound guidance developed by Yu et al. (11). It is a technique developed through defining the electrophysiological characteristics of the FO to create 3D transseptal puncture technology (11). However, performing zero-fluoroscopy transseptal puncture solely with T3D technology requires determining the FO position through electrograms and 3D modeling, lacking direct imaging information. Conversely, performing transseptal puncture solely with ICE can lead beginners to lose sight of the ultrasound image of the puncture needle, thereby increasing the difficulty of learning transseptal puncture. The combined T3D and ICE method used in the study addresses these challenges by integrating the advantages of both techniques. It supplements critical imaging information missing in the T3D process and, due to the visualization of the needle tip, facilitates locating the transseptal puncture needle’s position by adjusting the ultrasound plane. This ensures the safety of transseptal puncture by determining the appropriate puncture point and helps shorten the operator’s learning curve.
This study has several limitations. First and foremost, our research was a retrospective, non-randomized study. Even though we included all eligible patients over the study period, the performance of AF ablation with or without ICE was at discretion of operators, which may lead to selection bias. As well, the effectiveness and safety outcomes of ICE-guided radiofrequency ablation procedures may be associated with the experience of the operators, as different levels of operator skills could impact the outcomes. All surgeries included in the study were performed by the same operator, thereby minimizing bias in the study results due to operator’s experience. What is more, the research center in this study is a large tertiary hospital in China, and the skill level of operators involved in this study is similar to those who can perform ICE in China. Hence, the research findings hold a certain level of generalizability. Secondly, our study had a relatively small sample size, which limited its statistical power in testing the differences between ICE and non-ICE groups, increasing the chance of false negative findings. It is suggested that future real-world studies include more simple size and research centers. Thirdly, we had a relatively short follow-up period of only 6 months and also the coronavirus disease 2019 (COVID-19) was epidemic over the study period and a few patients were without full follow-up data largely due to the COVID-19 quarantine or isolation. Finally, the price of ICE catheters is considerably higher compared to traditional non-ICE-guided radiofrequency ablation. In our study, the cost aspect was not included in our research assessment. It is suggested that future real-world studies should include cost data to reflect the expenses incurred by patients using ICE catheters and evaluate the cost-effectiveness of ICE catheters for AF ablation.
Conclusions
In this retrospective study that included 97 Chinese patients, compared to fluoroscopy-guided radiofrequency ablation for treatment of AF, ICE-guided radiofrequency ablation significantly reduces fluoroscopy time and dose and achieves zero radiation exposure for all individuals involved in the procedure without compromising on safety and effectiveness in routine clinical practice.
Acknowledgments
Funding: This work was supported by
Footnote
Reporting Checklist: The authors have completed the STROBE reporting checklist. Available at https://jtd.amegroups.com/article/view/10.21037/jtd-23-1418/rc
Data Sharing Statement: Available at https://jtd.amegroups.com/article/view/10.21037/jtd-23-1418/dss
Peer Review File: Available at https://jtd.amegroups.com/article/view/10.21037/jtd-23-1418/prf
Conflicts of Interest: All authors have completed the ICMJE uniform disclosure form (available at https://jtd.amegroups.com/article/view/10.21037/jtd-23-1418/coif). All authors report that this work was supported by Johnson & Johnson Medical (China) Co., Ltd. C.Z. and X.L. are employees of Shanghai Centennial Scientific Co., Ltd., Shanghai, China. The authors have no other conflicts of interest to declare.
Ethical Statement: The authors are accountable for all aspects of the work in ensuring that questions related to the accuracy or integrity of any part of the work are appropriately investigated and resolved. The study was conducted in accordance with the Declaration of Helsinki (as revised in 2013). The study was approved by the Clinical Ethics Committee of The First Affiliated Hospital, Zhejiang University School of Medicine (No. IIT2020688) and individual consent for this retrospective analysis was waived.
Open Access Statement: This is an Open Access article distributed in accordance with the Creative Commons Attribution-NonCommercial-NoDerivs 4.0 International License (CC BY-NC-ND 4.0), which permits the non-commercial replication and distribution of the article with the strict proviso that no changes or edits are made and the original work is properly cited (including links to both the formal publication through the relevant DOI and the license). See: https://creativecommons.org/licenses/by-nc-nd/4.0/.
References
- Dong XJ, Wang BB, Hou FF, et al. Global burden of atrial fibrillation/atrial flutter and its attributable risk factors from 1990 to 2019. Europace 2023;25:793-803. [Crossref] [PubMed]
- Shi S, Tang Y, Zhao Q, et al. Prevalence and risk of atrial fibrillation in China: A national cross-sectional epidemiological study. Lancet Reg Health West Pac 2022;23:100439. [Crossref] [PubMed]
- Hindricks G, Potpara T, Dagres N, et al. 2020 ESC Guidelines for the diagnosis and management of atrial fibrillation developed in collaboration with the European Association for Cardio-Thoracic Surgery (EACTS): The Task Force for the diagnosis and management of atrial fibrillation of the European Society of Cardiology (ESC) Developed with the special contribution of the European Heart Rhythm Association (EHRA) of the ESC. Eur Heart J 2021;42:373-498. [Crossref] [PubMed]
- Chinese Society of Pacing and Electrophysiology, Chinese Society of Arrhythmias, Atrial Fibrillation Center Union of China. Current knowledge and management of atrial fibrillation: consensus of Chinese experts 2021. Chinese Journal of Cardiac Arrhythmias 2022;26:15-88.
- Enriquez A, Saenz LC, Rosso R, et al. Use of Intracardiac Echocardiography in Interventional Cardiology: Working With the Anatomy Rather Than Fighting It. Circulation 2018;137:2278-94. [Crossref] [PubMed]
- Vitulano N, Pazzano V, Pelargonio G, et al. Technology update: intracardiac echocardiography – a review of the literature. Med Devices (Auckl) 2015;8:231-9. [PubMed]
- Jingquan Z, Deyong L, Huimin C, et al. Intracardiac echocardiography Chinese expert consensus. Front Cardiovasc Med 2022;9:1012731. [Crossref] [PubMed]
- Anter E, Silverstein J, Tschabrunn CM, et al. Comparison of intracardiac echocardiography and transesophageal echocardiography for imaging of the right and left atrial appendages. Heart Rhythm 2014;11:1890-7. [Crossref] [PubMed]
- Lin J, Li H, Liu M, et al. Safety and Feasibility of Fluoroscopy-Free Atrial Transseptal Puncture Guided by Intracardiac Echocardiography. J Adv Cardiovasc Dis 2022;43:93-6.
- Bai XY, Bai ZL, Liu GQ, et al. Clinical application of zero-X ray radiofrequency ablation guided by a simple method of intracardiac echocardiography for atrial fibrillation. Chinese Journal of Evidence-Bases Cardiovascular Medicine 2021;13:541-4.
- Yu R, Liu N, Lu J, et al. 3-Dimensional Transseptal Puncture Based on Electrographic Characteristics of Fossa Ovalis: A Fluoroscopy-Free and Echocardiography-Free Method. JACC Cardiovasc Interv 2020;13:1223-32. [Crossref] [PubMed]
- Zhang R, Rao L, Peng Y. Clinical Application of Intracardiac Echocardiography. Advances in Cardiovascular Diseases 2020;41:926-9.
- Aldhoon B, Wichterle D, Peichl P, et al. Complications of catheter ablation for atrial fibrillation in a high-volume centre with the use of intracardiac echocardiography. Europace 2013;15:24-32. [Crossref] [PubMed]
- Žižek D, Antolič B, Prolič Kalinšek T, et al. Intracardiac echocardiography-guided transseptal puncture for fluoroless catheter ablation of left-sided tachycardias. J Interv Card Electrophysiol 2021;61:595-602. [Crossref] [PubMed]
- Jiang C, Ma C, Chen S, et al. Chinese expert consensus on the construction of the fluoroless cardiac electrophysiology laboratory and related techniques. Pacing Clin Electrophysiol 2023;46:1035-48. [Crossref] [PubMed]
- JiangMD R, ChenMD M, LiuMS Q, et al. Body pain – An unheeded personal health hazard in interventional cardiologists: A national online cross-sectional survey study in China. Int J Cardiol 2022;350:27-32. [Crossref] [PubMed]
- Zei PC, Hunter TD, Gache LM, et al. Low-fluoroscopy atrial fibrillation ablation with contact force and ultrasound technologies: a learning curve. Pragmat Obs Res 2019;10:1-7. [Crossref] [PubMed]