CXCR7 promotes pulmonary vascular remodeling via targeting p38/MMP2 pathway in pulmonary arterial hypertension
Highlight box
Key findings
• We revealed that C-X-C motif chemokine ligand 12 (CXCL12)/chemokine receptor type 7 (CXCR7)/p38 MAPK signaling is critical to hypoxic pulmonary arterial hypertension (PAH).
What is known and what is new?
• PAH is a high-mortality disease with excessive proliferation of pulmonary artery smooth muscle cells. The vascular cell populations that contribute to the molecular and morphological changes of PAH in conjunction with current animal models for studying vascular remodeling in PAH will be promising.
• We identified the critical role of CXCL12/CXCR7/p38 MAPK signaling in hypoxia-induced PAH and the CXCL12/CXCR7 signaling pathway-mediated elevation of MMP2 expression via p38 MAPK is involved in the proliferation of pulmonary artery smooth muscle cells.
What is the implication, and what should change now?
• The findings of this study will assist further research into CXCR7 target in PAH and offer an alternative pharmacological treatment for vascular diseases.
Introduction
In pulmonary arterial hypertension (PAH), the pulmonary arteries are remodeled with medial hypertrophy, intimal proliferation, and adventitial thickening, which eventually leads to right ventricular failure (1). Small peripheral pulmonary arteries (<50 µm) can become blocked due to the proliferation pulmonary artery smooth muscle cells (PASMCs) within the medial layer of the lungs. PASMC proliferation is believed to play a critical role in the pathogenesis of the disease and can trigger pulmonary vascular remodeling (2). Although important advances have been made in recent years, the precise basis of the molecular and cellular mechanism in pulmonary vascular remodeling is still undefined. A genetic and genomic perspective on PAH, allowing insights into pathobiology, and providing opportunities for developing novel therapeutic approaches (3).
The chemokine system consists of around 50 ligands and 20 receptors in humans, and takes part in many aspects of central nervous system development (4), tumorigenesis (5), and angiogenesis (6,7). The C-X-C motif chemokine family has received increased attention due to its role in PAH. Specifically, by binding to its receptor, C-X-C motif chemokine ligand 12 (CXCL12) modulates a variety of cellular activities. A growing body of evidence suggests that comprehending the role that chemokine receptor type 4 (CXCR4) plays in the progression of PAH has clinical relevance (8). Research suggests that chemokine receptor type 7 (CXCR7) is also expressed in various cells as part of the CXCL12 signaling pathway. Importantly, among its functions, CXCR7 regulates proliferation, survival, and metastasis in cancer cells (9,10). Moreover, activation of CXCR7 has been reported to modulate pulmonary vascular endothelial cell proliferation and wound repair (11,12). However, until now, there has been no data available on CXCR7 signaling alteration and its potential molecular mechanism related to vascular PASMCs from PAH. Therefore, acquiring a more comprehensive understanding of the relationship between CXCR7 and PASMCs warrants further investigation.
It is believed that matrix metalloproteinases (MMPs) play an important role in extracellular matrix (ECM) remodeling upon proteolysis of various ECM components and are involved in the pathogenesis of many diseases. As important factors governing structural alterations of the vasculature, MMPs regulate cell growth, migration, angiogenesis, and arteriogenesis (13), and are also implicated in apoptosis (14). In the initial stages of PAH, MMP2, which is produced by PASMCs, affects the ECM, causing it to remodel and disrupt (15). MMP2 has been demonstrated to be accountable for the proliferation of vascular smooth muscle cells and fibroblasts (16,17), specifically in relation to vascular smooth muscle cell proliferation and migration in PAH. Moreover, a tight link between CXCR7 and MMP2 has been suggested in cancer cells (18) and endothelial cells function (19) in triggering cell growth and survival. However, activation of MMP2 mediated by CXCL12/CXCR7 in PAH has not been examined in depth.
We therefore examined the effect of CXCL12/CXCR7 on MMP2 expression in our study. CXCR7 expression was upregulated in PAH with activation of MMP2, and inhibition of CXCR7 ameliorated the development of PAH. Our purpose was to determine the possible role of CXCR7 in hypoxia-induced PAH and to clarify how CXCL12/CXCR7 modulates p38 MAPK signaling and MMP2 level in PAH. We present this article in accordance with the ARRIVE reporting checklist (available at https://jtd.amegroups.com/article/view/10.21037/jtd-24-331/rc).
Methods
Materials
Antibodies against MMP2 (#ab86607; 1 µg/mL dilution; Abcam, Cambridge, UK), CXCR7 (#ab124824; 1:500 dilution; Abcam), phosphor- (p-)ERK (#4376S; 1:1,000 dilution; Cell Signaling Technology, Danvers, MA, USA), ERK (#9102S; 1:1,000 dilution; Cell Signaling Technology), p-JNK (#9251S, 1:1,000 dilution; Cell Signaling Technology), JNK (#9252S; 1:1,000 dilution; Cell Signaling Technology), p38 MAPK (#9212S; 1:1,000 dilution; Cell Signaling Technology), p-p38 MAPK (#9211S; 1:1,000 dilution; Cell Signaling Technology), and α-tubulin (#AC012, 1:10,000 dilution; ABclonal, Woburn, MA, USA) were obtained. Secondary antibodies were purchased from BioWorld Technology, Inc. (Irving, TX, USA) and applied at a 1:10,000 dilution. Enhanced chemiluminescence (ECL) reagents were obtained from Millipore (Burlington, MA, USA), and CCX771 was purchased from ChemoCentryx, Inc. (Mountain View, CA, USA).
Animal models
All procedures involving animals were approved by the Nanjing Medical University’s Institutional Animal Care and Use Committee [No. 2023(149), Nanjing, China], in compliance with national guidelines for the care and use of animals. A protocol was prepared before the study without registration. Male Sprague-Dawley (SD) rats (180–220 g) were purchased from the Animal Center of Nanjing Medical University and randomly divided into three groups: a control group (n=7), a PAH group (n=7), and a treatment group (n=7). The rats in the second and third groups were exposed to chronic hypoxia (10% oxygen) for 28 days. The normoxic control group was injected intraperitoneally with phosphate-buffered saline (PBS) for 3 weeks and kept at 21% O2 for 4 weeks. The hypoxic control group rats were kept at 10% O2 for 4 weeks after intratracheal injection with PBS for 3 weeks. Hypoxic CCX771 group rats were injected with CCX771 (20 mg/kg/day, intraperitoneal injection). Four weeks later, all rats were anesthetized for echocardiography measurements and hemodynamic analysis. Finally, the rats were killed, and their lung tissues and peripheral blood were extracted for further analysis.
Measurement of right ventricular systolic pressure (RVSP)
RVSP was measured via right-heart catheterization according to a previous study (20). Pentobarbital sodium was injected intraperitoneally to anesthetize rats before catheterization.
PAH remodeling and right ventricular hypertrophy assessment
In the previous study, we discussed the use of wall thickness and medial wall thickness percentages when assessing pulmonary arterial remodeling (21). The excised heart was divided into two parts: the pulmonary trunk with the aorta and the right ventricular wall with the ventricular septum. Left ventricle, right ventricle, and ventricular septum weights were calculated, and the right ventricular hypertrophy index (RVHI) was calculated as the weight ratio of right ventricle to (left ventricle + interventricular septum).
Evaluation of cardiac function using echocardiography
The cardiac function of SD rats was assessed using the Vevo2100 system (Fujifilm VisualSonics, Inc., Toronto, ON, Canada) with a high-frequency (30 MHz) MS-400 transducer. The right ventricular internal dimension in diastole (RVID), pulmonary artery acceleration time (PAT), pulmonary ejection time (PET), and left ventricle ejection fraction (LVEF) were recorded.
PASMC isolation and culture
We obtained PASMCs from the peripheral small pulmonary arteries of rats and exposed the luminal surface with PAH sections. The endothelium was removed by gentle scraping with a scalpel blade, and the intima was peeled away from the adventitial layer. At 37 ℃ and 5% carbon dioxide, we segmented the medial explants into 12 mm2 segments and allowed them to adhere for 2 hours. The cells were then cultured in Dulbecco’s modified Eagle’s medium (DMEM; Gibco, Thermo Fisher Scientific, Waltham, MA, USA) containing 4.5 mmol/L of D-glucose supplemented with 10% fetal bovine serum (FBS; Gibco, Thermo Fisher Scientific), 100 U/mL of penicillin, and 100 µg/mL of streptomycin at 37 ℃ with 3% O2, 5% CO2, and 92% N2. α-smooth muscle actin (SMA) was used to identify PASMCs. Three to eight passages of cells were used in the experiments. All PASMCs were starved for 24 hours before treatment to rule out the effects of FBS.
Assessment of PASMC proliferation
In serum-free DMEM, PASMCs were seeded in 96-well plates for 24 hours after seeding (about 1×104 cells per well) and were then exposed to various concentrations (10 and 20 ng/mL) of CXCL12, CCX771 (2 µM), or SB203580 (20 µM), respectively. Following this, the samples were exposed to hypoxia (3% O2). We used the modified 3-(4,5-dimethylthiazol-2-yl)-2,5-diphenyltetrazolium bromide (MTT) assay to quantify the cells. Briefly, 0.25 mg/mL of MTT was added to each well. After incubation at 37 ℃ for 4 hours, the supernatant was removed, and the reaction was terminated via addition of dimethyl sulfoxide (DMSO) to the medium. Measurement of absorption at 490 nm was performed using an enzyme-linked immunosorbent assay (ELISA) reader (Thermo Fisher Scientific). All determinations were confirmed in at least three independent experiments.
ELISA
The blood samples were centrifuged at 3,000 g for 10 min at 4 ℃, and the obtained serum was stored at −80 ℃ until assayed. Through ELISA, we assessed the levels of CXCL12 protein. Briefly, the CXCL12 protein level in neutrophil suspensions was isolated from the blood samples using a rat CXCL12 or SDF-1 ELISA kit (USCN, Wuhan, China) according to the manufacturer’s instructions. In each experiment, seven animals from three separate experiments were evaluated.
Histological analysis
After lung removal, lungs were fixed with 4% paraformaldehyde solution and embedded in paraffin. Left ventricular sections were cut in 5 mm sections for hematoxylin-eosin staining.
Western blot analysis
Samples from animal lungs were cut into pieces and homogenized in cold PBS. We centrifugated the tissue mixture and removed the PBS, isolated pulmonary arterioles or cells were lysed in radioimmunoprecipitation assay (RIPA) buffer for 30 min, and centrifuged at 12,000 g at 4 ℃ for 20 min. In routine fashion, bovine serum albumin was used to determine supernatant protein concentration via spectrophotometry (bicinchoninic acid assay; Pierce, Rockford, IL, USA). Protein samples (50–100 mg) were separated on sodium dodecyl sulfate-polyacrylamide gel electrophoresis (SDS-PAGE) gels and transferred to polyvinylidene fluoride (PVDF) membranes. The membranes were subjected to a 1 hour incubation period with a 5% nonfat dry milk solution in tris-buffered saline with Tween20 (consisting of 20 M Tris-HCl, pH 7.6; 150 mM NaCl; and 0.05% Tween20) in order to prevent nonspecific binding. Under gentle shaking, the membranes were subjected to overnight incubation at 4 ℃ with specific primary antibodies and subsequently incubated with secondary antibodies for a duration of 1 hour. α-tubulin was used as a loading control to normalize the data. The final visualization of the results was conducted using Syngene Bio Imaging Devices and chemiluminescence (Syngene, Cambridge, UK), and the immunoreactive band density was calculated with ImageJ software (National Institutes of Health, Bethesda, MD, USA).
Statistical analysis
All data are presented as the mean ± stand error of the mean (SEM). Comparisons of two groups were conducted with the Student’s t-test, whereas comparisons of three or more groups were determined via analysis of variance (ANOVA) and followed by the Student-Newman-Keuls post-hoc analysis conducted via GraphPad Prism 5.0 (GraphPad Software, La Jolla, CA, USA), as appropriate. P values less than 0.05 were considered statistically significant when the sample size was at least five.
Results
CXCL12/CXCR7 was upregulated in PAH
The progression of PAH was influenced by the expression of the chemokine receptor CXCR7 in various cell lines (8,22); however, we know very little about CXCR7 expression in PASMCs. To study the role of CXCL12/CXCR7 in PAH, we first assessed the levels of CXCL12/CXCR7 and found them to be slightly elevated after 2 and 4 weeks compared with baseline. In Figure 1A, CXCL12 protein levels were significantly upregulated in serum of hypoxic rats. We also observed similar expression patterns in the Western blotting for CXCR7 protein (Figure 1B,1C). Taken together, our results indicate that CXCL12/CXCR7 was upregulated in PAH.
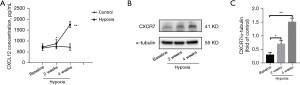
CXCL12/CXCR7 upregulated MMP2 signaling both in vivo and vitro
CXCR7 has been established as a chemokine receptor for CXCL12; however, the nature of the interaction between CXCL12 and CXCR7 in PAH is unclear. We thus analyzed the effect of CXCR7 agonist CXCL12 on MMP2 expression in PASMCs. Since CXCR7 expression was positively correlated with the expression of MMP2 in several cell lines, we assessed the effects of CXCR7 on MMP2 expression in PASMCs. We observed MMP2 expression increased in vivo in similar fashion to CXCR7 (Figure 2A,2B). Exposure to 20 ng/mL of CXCL12 for 12 hours resulted in a notable increase in CXCR7 and MMP2 levels compared to those in the control group (Figure 2C,2D). After pretreatment with CXCR7 inhibitor CCX771, the exogenous CXCL12-induced upregulation of MMP2 protein was inhibited (Figure 2C,2D). It appears that CXCL12 and CXCR7 play a key role in increasing MMP2 expression in PASMCs. Overall, our data confirm that CXCL12 enhances MMP2 expression by binding to the CXCR7 receptor.
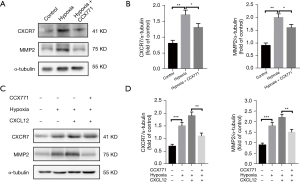
p38 MAPK was associated with CXCL12/CXCR7-mediated MMP2 activation in PASMCs
To clarify the underlying mechanisms of the regulation of MMP2 in PASMCs via CXCL12/CXCR7, we analyzed MAPK signaling. There was a slight increase in p-p38 MAPK, phosphor-ERK, and phosphor-JNK in the control group and dramatic increase in CXCL12-stimulated groups. Via the inhibition of CXCR7, the phosphorylation of p38 MAPK induced by CXCL12 was significantly mitigated, but not in the phosphorylation of ERK or JNK. Thus, it seems that CXCL12/CXCR7 primarily activates the p38 MAPK pathway (Figure 3A,3B).
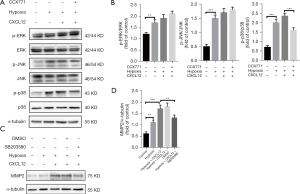
SB203580 was introduced as a specific inhibitor of p38 MAPK to analyze the CXCL12/CXCR7 interaction in PASMCs in further detail. The results of Western blotting confirmed that SB203580 blocked CXCL12/CXCR7-mediated MMP2 activation (Figure 3C,3D), suggesting that MMP2 and p38 MAPK may be linked to PAH downstream of CXCL12/CXCR7.
CXCL12/CXCR7 regulated PASMC proliferation partially via the p38 MAPK pathways
In PAH, chronic hypoxia can lead to abnormal proliferation and migration of the PASMCs as well as remodeling of the pulmonary vessels (21). Our data also revealed that hypoxia increased the number of PASMCs, and inhibition of CXCR7 in PASMCs reduced their proliferation. The upregulation of p38 MAPK protein phosphorylation in PASMCs was observed following the administration of its agonists, CXCL12, and SB203580 was added to PASMCs without disturbing the p38 MAPK protein phosphorylation (Figure 4A,4B). PASMCs were exposed to hypoxia and CXCL12 (20 ng/mL) for 24 hours, resulting in a significantly higher rate of proliferation (Figure 4C). However, the proliferation viability of PASMCs was decreased compared with that of controls. To identify the effects of p38 MAPK pathways on PASMC proliferation in the presence of CXCR7, CCX771 or SB203580 was added to PASMCs stably expressing CXCR7 at concentrations of 0.5 and 25 M, respectively. Cell proliferation decreased in the SB203580-treated group (Figure 4D), indicating that the p38 MAPK pathways is a functional target of CXCR7. Furthermore, activation of CXCR7 constitutively increased the phosphorylation of p38 MAPK, and CXCL12-mediated proliferation was abrogated by p38 MAPK inhibition. To conclude, CXCL12/CXCR7 regulated PASMC proliferation at least partially via the p38 MAPK pathways.
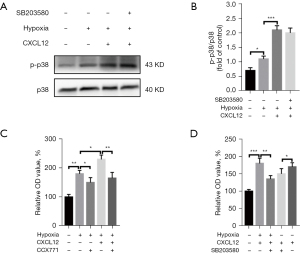
Inhibition of CXCR7 rescued pulmonary vascular remodeling in SD rats
The levels of CXCR7 exhibited an increase in both hypoxia-PASMCs and the pulmonary arteries of rats exposed to hypoxia. We hypothesized that CXCR7 inhibition could alleviate vascular remodeling in experimental PAH. For 4 weeks, rats were divided into three groups and treated in hypoxic or normoxic conditions, and the treated group was intraperitoneally injected a dose of 20 mg per kg body weight CCX771 or vehicle for 4 weeks. The mean RVSP of the hypoxia + CCX771 group was much lower than that of the hypoxia group (Figure 5A). Compared to the hypoxia group, the treated group had a lower RVSP (Figure 5B). We examined whether CCX771 could attenuate pulmonary artery remodeling in hypoxic rats. In hypoxic PAH, precapillary arterioles are often severely muscularized after new growth. In the hypoxia group, CCX771 significantly reduced the thickness of pulmonary vessels (Figure 5C). The findings indicated a decrease in the muscularization of distal pulmonary arteries in the treated group when compared to the hypoxia condition. Since the ratio of right ventricle to left ventricle plus septum was lower in the treated group, and the RVHI in the treated group decreased (Figure 5D). The right ventricular chamber size decreased markedly under CCX771 treatment according to echocardiography. Additionally, PAH rats showed a large RVID despite normal cardiac function and systemic pressure (Figure 5E,5F). In contrast, none of the treatment groups showed elevated systemic arterial blood pressure (carotid artery) (Figure 5G). Finally, we observed treatment with CCX771 in hypoxic rats significantly reduced CXCR7/MMP2 signaling, thus confirming the effects of CXCR7 inhibition in vivo.
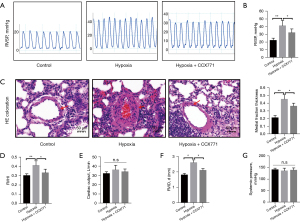
Discussion
PAH is a life-threatening disease with high mortality. A growing body of evidence supports the hypothesis that PAH is caused by abnormal proliferation of PASMCs. In this study, we demonstrated that the CXCL12/CXCR7 signaling pathway-mediated elevation of MMP2 expression via p38 MAPK is involved in the proliferation of PASMCs in vitro. A highly selective inhibitor of CCX771 effectively counteracted pulmonary vessel remodeling, pulmonary arterial pressure, medial hypertrophy of the pulmonary artery, and right ventricular hypertrophy in rats exposed to hypoxia. CXCR7 agonists could promote the proliferation of PASMCs, while CXCR7 antagonists had the opposite effect. CXCL12/CXCR7 regulated the activation of MMP2 and p38 MAPK signaling pathway but not that of ERK or JNK signaling, revealing that CXCL12/CXCR7/p38 MAPK signaling is critical to hypoxic PAH.
CXCL12 is a unique chemokine that is highly conserved in mammals. It can bind to receptors and stimulate many signaling pathways (23). We previously demonstrated that the CXCL12-CXCR4 signaling axis is crucially involved in PAH (6). Recently, a variety of diseases, including cancer, cardiac disorders, autoimmune disorders, and neointima formation, were associated with CXCR7 (24); however, the evidence regarding the relationship between CXCR7 and PAH is limited. We observed an increased CXCR7 level in the pulmonary vessels isolated from hypoxic rats, which was consistent with previous findings (25). It is also worth noting that CXCR7 was upregulated in hypoxic-stimulated normal PASMCs. In patients with idiopathic PAH, the circulating levels of the CXCL12 are increased, and this level is an independent predictor of mortality (26). The increased level of chemokines may induce cell proliferation by stimulating multiple cells and binding to their receptors, resulting in pulmonary vascular remodeling.
The proliferation of PASMCs is stimulated by multiple growth factors and cytokines, which ultimately results in the formation of hypoxic pulmonary vascular reconstruction lesions. A study has shown that CXCL12 attracts bone marrow-derived progenitor cells or mesenchymal stem cells that express CXCR4 and CXCR7 to the subintimal layer (27).
As the link between classical receptor CXCR4 and pulmonary vascular remodeling continues to be investigated, other important receptors expressed in the pathological process of PASMCs will be identified. In our study, serum CXCL12 was elevated and CXCR7 expression was increased in the thickened pulmonary arterioles of hypoxic rats. It can thus be speculated that hypoxia increases the circulation of CXCL12, causing SMCs to secrete more CXCR7, allowing SMC proliferation to contribute to subintima thickening of the vessel wall. It is common for CXCL12 to induce the proliferation of cells, such as colon cancer cells (28), glioma cells (29), and several types of progenitor and stem cells (30). Our in vitro data indicated that CXCL12 stimulated PASMC growth under hypoxic conditions, which is a critical step in the vascular disease process.
A critical component of pulmonary vascular remodeling is ECM remodeling. The ECM primarily consists of collagen, elastin fibers, proteoglycans, and glycoproteins, with collagen and elastin fibers being the predominant structural elements (31). Generally, MMPs secreted by various cells, especially MMP2, play crucial roles in the development of cardiovascular diseases (32), and their pertinence to pulmonary vascular reconstruction in both animal models and clinical patients has been reported (14,33). MMPA-2 not only degrades collagen 2, but also cleaves elastin, disrupting the internal elastic lamina and facilitating SMC migration, with cell proliferation occurring in response to a variety of stimuli, leading to thickening of the intima (34). In our study, when exogenous CXCL12 was added, we also found that MMP2 and CXCR7 were activated, which indicated MMP2 was the downstream molecule of CXCL12/CXCR7, both of which were involved in the adaptive change of PASMCs to hypoxia. Furthermore, the CXCR7 special inhibitor competitively suppressed the activation of MMP2 in response to CXCL12. In PAH, CXCL12/CXCR7 pathway plays a major role in pulmonary vascular remodeling, and CXCR7 acts as an upstream signal to MMP2.
MAPK is most closely related to the regulation of cell proliferation. Eukaryotic cells contain MAPK as a signal transduction protein kinase (35). In extracellular signals and cell nuclei, MAPK is the pathway for information transfer (36). The signal transduction pathway operates via a conserved three-level kinase cascade reaction. A variety of extracellular and intracellular stimuli can activate the MAPK pathway, such as cytokines, growth factors, and transcription factors; therefore, it can mediate relevant biological processes and regulate the production of MMPs (37,38). Yue et al. (39) previously reported that p38 MAPK regulates the proliferation of PASMCs. In our study, to further clarify how CXCL12/CXCR7 induces proliferation in PASMCs, the p-p38 MAPK pathway was examined. We found that CXCL12/CXCR7 could activate p38 MAPK signaling in PASMCs, as coculturing with CXCR7 antagonist significantly reduced CXCL12-induced phosphorylation of p38 MAPK, but not that of ERK or JNK signaling. CXCL12/CXCR7 increased MMP2 expression by activating the p38 MAPK pathway, whereas preincubation with SB203580 decreased MMP2 expression. This result indicated that MMP2 was one of downstream molecules of CXCL12/CXCR7. Moreover, p38 MAPK inhibitor SB203580 abolished CXCL12-induced cell proliferation and the downregulation of MMP2, indicating that CXCL12/CXCR7 regulates MMP2 expression in part through the activation of the p38 MAPK pathway.
There are some limitations to our study that should be mentioned. First, the study only examined PASMCs, but vascular endothelial cells, poor endothelial coverage, and fibroblasts also contribute to vascular dysfunction, and thus further exploration is warranted. Additionally, the rat model was limited by the rapid development of disease phenotype and maladaptive remodeling processes compared with those in humans. Moreover, inhibiting CXCR7 for 4 weeks might not have been sufficient to produce clinically significant PVR improvement.
Conclusions
In conclusion, our data confirmed the significance of CXCL12/CXCR7 as a mediator of hypoxia-induced pulmonary vascular remodeling. We demonstrated, for the first time, that the p38 MAPK/MMP2 pathway is responsible for CXCL12/CXCR7-mediated proliferation in PASMCs. The findings of this study will assist further research into CXCR7 antagonists in PAH and offer an alternative pharmacological treatment for vascular diseases.
Acknowledgments
Funding: This study was supported by
Footnote
Reporting Checklist: The authors have completed the ARRIVE reporting checklist. Available at https://jtd.amegroups.com/article/view/10.21037/jtd-24-331/rc
Data Sharing Statement: Available at https://jtd.amegroups.com/article/view/10.21037/jtd-24-331/dss
Peer Review File: Available at https://jtd.amegroups.com/article/view/10.21037/jtd-24-331/prf
Conflicts of Interest: All authors have completed the ICMJE uniform disclosure form (available at https://jtd.amegroups.com/article/view/10.21037/jtd-24-331/coif). The authors have no conflicts of interest to declare.
Ethical Statement: The authors are accountable for all aspects of the work in ensuring that questions related to the accuracy or integrity of any part of the work are appropriately investigated and resolved. All procedures involving animals were approved by the Nanjing Medical University’s Institutional Animal Care and Use Committee [No. 2023(149), Nanjing, China], in compliance with national guidelines for the care and use of animals.
Open Access Statement: This is an Open Access article distributed in accordance with the Creative Commons Attribution-NonCommercial-NoDerivs 4.0 International License (CC BY-NC-ND 4.0), which permits the non-commercial replication and distribution of the article with the strict proviso that no changes or edits are made and the original work is properly cited (including links to both the formal publication through the relevant DOI and the license). See: https://creativecommons.org/licenses/by-nc-nd/4.0/.
References
- Hoeper MM, Humbert M. The new haemodynamic definition of pulmonary hypertension: evidence prevails, finally! Eur Respir J 2019;53:1900038. [Crossref] [PubMed]
- Li B, Teng C, Yu H, et al. Alleviating experimental pulmonary hypertension via co-delivering FoxO1 stimulus and apoptosis activator to hyperproliferating pulmonary arteries. Acta Pharm Sin B 2023;13:2369-82. [Crossref] [PubMed]
- Morrell NW, Aldred MA, Chung WK, et al. Genetics and genomics of pulmonary arterial hypertension. Eur Respir J 2019;53:1801899. [Crossref] [PubMed]
- Yan Y, Su J, Zhang Z. The CXCL12/CXCR4/ACKR3 Response Axis in Chronic Neurodegenerative Disorders of the Central Nervous System: Therapeutic Target and Biomarker. Cell Mol Neurobiol 2022;42:2147-56. [Crossref] [PubMed]
- Yang Y, Li J, Lei W, et al. CXCL12-CXCR4/CXCR7 Axis in Cancer: from Mechanisms to Clinical Applications. Int J Biol Sci 2023;19:3341-59. [Crossref] [PubMed]
- Li X, Li Z, Li C, et al. Contrast-enhanced ultrasound for the evaluation of CXCR7-mediated angiogenesis in colon cancer. J Cancer 2023;14:665-75. [Crossref] [PubMed]
- Wang S, Lou N, Luo R, et al. Role of chemokine-mediated angiogenesis in resistance towards crizotinib and its reversal by anlotinib in EML4-ALK positive NSCLC. J Transl Med 2022;20:248. [Crossref] [PubMed]
- Xu J, Li X, Zhou S, et al. Inhibition of CXCR4 ameliorates hypoxia-induced pulmonary arterial hypertension in rats. Am J Transl Res 2021;13:1458-70.
- Kim S, Kim JE, Kim N, et al. Decursin inhibits tumor growth, migration, and invasion in gastric cancer by down-regulating CXCR7 expression. Am J Cancer Res 2019;9:2007-18.
- Gao JH, Yu XH, Tang CK. CXC chemokine ligand 12 (CXCL12) in atherosclerosis: An underlying therapeutic target. Clin Chim Acta 2019;495:538-44. [Crossref] [PubMed]
- Sartina E, Suguihara C, Ramchandran S, et al. Antagonism of CXCR7 attenuates chronic hypoxia-induced pulmonary hypertension. Pediatr Res 2012;71:682-8. [Crossref] [PubMed]
- Li Q, Guo Y, Chen F, et al. Stromal cell-derived factor-1 promotes human adipose tissue-derived stem cell survival and chronic wound healing. Exp Ther Med 2016;12:45-50. [Crossref] [PubMed]
- Mustafa S, Koran S, AlOmair L. Insights Into the Role of Matrix Metalloproteinases in Cancer and its Various Therapeutic Aspects: A Review. Front Mol Biosci 2022;9:896099. [Crossref] [PubMed]
- Bai P, Lyu L, Yu T, et al. Macrophage-Derived Legumain Promotes Pulmonary Hypertension by Activating the MMP (Matrix Metalloproteinase)-2/TGF (Transforming Growth Factor)-β1 Signaling. Arterioscler Thromb Vasc Biol 2019;39:e130-45. [Crossref] [PubMed]
- Belo VA, Guimarães DA, Castro MM. Matrix Metalloproteinase 2 as a Potential Mediator of Vascular Smooth Muscle Cell Migration and Chronic Vascular Remodeling in Hypertension. J Vasc Res 2015;52:221-31. [Crossref] [PubMed]
- Cabral-Pacheco GA, Garza-Veloz I, Castruita-De la Rosa C, et al. The Roles of Matrix Metalloproteinases and Their Inhibitors in Human Diseases. Int J Mol Sci 2020;21:9739. [Crossref] [PubMed]
- Cao Z, Lis R, Ginsberg M, et al. Targeting of the pulmonary capillary vascular niche promotes lung alveolar repair and ameliorates fibrosis. Nat Med 2016;22:154-62. [Crossref] [PubMed]
- Zhao ZW, Fan XX, Song JJ, et al. ShRNA knock-down of CXCR7 inhibits tumour invasion and metastasis in hepatocellular carcinoma after transcatheter arterial chemoembolization. J Cell Mol Med 2017;21:1989-99. [Crossref] [PubMed]
- Zhao K, Yao Y, Luo X, et al. LYG-202 inhibits activation of endothelial cells and angiogenesis through CXCL12/CXCR7 pathway in breast cancer. Carcinogenesis 2018;39:588-600. [Crossref] [PubMed]
- Wang JJ, Zuo XR, Xu J, et al. Evaluation and Treatment of Endoplasmic Reticulum (ER) Stress in Right Ventricular Dysfunction during Monocrotaline-Induced Rat Pulmonary Arterial Hypertension. Cardiovasc Drugs Ther 2016;30:587-98. [Crossref] [PubMed]
- Nie X, Tan J, Dai Y, et al. CCL5 deficiency rescues pulmonary vascular dysfunction, and reverses pulmonary hypertension via caveolin-1-dependent BMPR2 activation. J Mol Cell Cardiol 2018;116:41-56. [Crossref] [PubMed]
- Shi A, Wang T, Jia M, et al. Effects of SDF-1/CXCR7 on the Migration, Invasion and Epithelial-Mesenchymal Transition of Gastric Cancer Cells. Front Genet 2021;12:760048. [Crossref] [PubMed]
- Wu X, Qian L, Zhao H, et al. CXCL12/CXCR4: An amazing challenge and opportunity in the fight against fibrosis. Ageing Res Rev 2023;83:101809. [Crossref] [PubMed]
- Sarma P, Carino CMC, Seetharama D, et al. Molecular insights into intrinsic transducer-coupling bias in the CXCR4-CXCR7 system. Nat Commun 2023;14:4808. [Crossref] [PubMed]
- Daniel SK, Seo YD, Pillarisetty VG. The CXCL12-CXCR4/CXCR7 axis as a mechanism of immune resistance in gastrointestinal malignancies. Semin Cancer Biol 2020;65:176-88. [Crossref] [PubMed]
- McCullagh BN, Costello CM, Li L, et al. Elevated plasma CXCL12α is associated with a poorer prognosis in pulmonary arterial hypertension. PLoS One 2015;10:e0123709. [Crossref] [PubMed]
- Shao Y, Zhou F, He D, et al. Overexpression of CXCR7 promotes mesenchymal stem cells to repair phosgene-induced acute lung injury in rats. Biomed Pharmacother 2019;109:1233-9. [Crossref] [PubMed]
- Ma J, Su H, Yu B, et al. CXCL12 gene silencing down-regulates metastatic potential via blockage of MAPK/PI3K/AP-1 signaling pathway in colon cancer. Clin Transl Oncol 2018;20:1035-45. [Crossref] [PubMed]
- Yi L, Zhou X, Li T, et al. Notch1 signaling pathway promotes invasion, self-renewal and growth of glioma initiating cells via modulating chemokine system CXCL12/CXCR4. J Exp Clin Cancer Res 2019;38:339. [Crossref] [PubMed]
- Zhao Z, Ma X, Ma J, et al. Naringin enhances endothelial progenitor cell (EPC) proliferation and tube formation capacity through the CXCL12/CXCR4/PI3K/Akt signaling pathway. Chem Biol Interact 2018;286:45-51. [Crossref] [PubMed]
- Liang D, Liu L, Zhao Y, et al. Targeting extracellular matrix through phytochemicals: a promising approach of multi-step actions on the treatment and prevention of cancer. Front Pharmacol 2023;14:1186712. [Crossref] [PubMed]
- Jiao X, Yu H, Du Z, et al. Vascular smooth muscle cells specific deletion of angiopoietin-like protein 8 prevents angiotensin II-promoted hypertension and cardiovascular hypertrophy. Cardiovasc Res 2023;119:1856-68. [Crossref] [PubMed]
- Ahmed A, Ahmed S, Arvidsson M, et al. Prolargin and matrix metalloproteinase-2 in heart failure after heart transplantation and their association with haemodynamics. ESC Heart Fail 2020;7:223-34. [Crossref] [PubMed]
- Samah N, Ugusman A, Hamid AA, et al. Role of Matrix Metalloproteinase-2 in the Development of Atherosclerosis among Patients with Coronary Artery Disease. Mediators Inflamm 2023;2023:9715114. [Crossref] [PubMed]
- Ma H, Qi G, Han F, et al. HMGB3 promotes the malignant phenotypes and stemness of epithelial ovarian cancer through the MAPK/ERK signaling pathway. Cell Commun Signal 2023;21:144. [Crossref] [PubMed]
- Zhang L, Zhou T, Ji Q, et al. Myricetin improves apoptosis after ischemic stroke via inhibiting MAPK-ERK pathway. Mol Biol Rep 2023;50:2545-57. [Crossref] [PubMed]
- Mummidi S, Das NA, Carpenter AJ, et al. RECK suppresses interleukin-17/TRAF3IP2-mediated MMP-13 activation and human aortic smooth muscle cell migration and proliferation. J Cell Physiol 2019;234:22242-59. [Crossref] [PubMed]
- Zhu G, Shen Q, Jiang H, et al. Curcumin inhibited the growth and invasion of human monocytic leukaemia SHI-1 cells in vivo by altering MAPK and MMP signalling. Pharm Biol 2020;58:25-34. [Crossref] [PubMed]
- Yue Y, Li YQ, Fu S, et al. Osthole inhibits cell proliferation by regulating the TGF-β1/Smad/p38 signaling pathways in pulmonary arterial smooth muscle cells. Biomed Pharmacother 2020;121:109640. [Crossref] [PubMed]