Intraoperative changes in upper extremity perfusion index after thoracic paravertebral and intertransverse process block in patients undergoing lung resection surgery
Highlight box
Key findings
• Paravertebral block induced a noticeable unilateral increase in upper extremity perfusion index (PI), whereas intertransverse process block tended to result in an inconsistent and lesser degree of increase.
What is known and what is new?
• PI has been used as a surrogate marker of sympathetic blockade.
• By monitoring bilateral upper extremity PI, sympathetic blockade can be observed intraoperatively.
What is the implication, and what should change now?
• Monitoring PI values can serve as an indicator of upper extremity sympathetic blockade, but consideration of potential confounders impacting these observations during surgery is essential.
Introduction
There has been an enthusiasm around paraspinal blocks (1-5). These blocks include erector spinae plane block, intertransverse process block (ITPB), and retrolaminar block, and commonly aims paravertebral spreading of local anesthetic without directly introducing the needle into the paravertebral space (6). This kind of approaches can relieve technical difficulty and decrease risks associated with the paravertebral block (PVB).
Previous cadaveric studies have demonstrated the spread of dye into the paravertebral space following paraspinal blocks (7-9). However, despite these findings, there is a lack of clinical data on the efficacy of these blocks in achieving sympathetic blockade. It is important to consider that cadaveric studies may have limitations and may not accurately reflect results in living patients (10,11). This highlights the need for a clinically feasible tool to evaluate sympathetic blockade.
Perfusion index (PI) is a parameter that derived from a photoplethysmogram signal and reflects the pulsatile versus non-pulsatile component of the signal. This index has been used as a surrogate marker of sympathetic blockade (12-16). This pilot study hypothesized that thoracic PVB and ITPB would increase PI of upper extremity. The aims of this study were two-fold: (I) to evaluate intraoperative changes in PI after these blockades; (II) to set up a framework for the analysis of intraoperative PI changes after these blockades. We present this article in accordance with the TREND reporting checklist (available at https://jtd.amegroups.com/article/view/10.21037/jtd-24-69/rc).
Methods
This was a joined work of two pilot studies, one for (I) PVB; and the other for (II) ITPB, both conducted at a university affiliated hospital (Chungnam National University Hospital, Daejeon, Korea) from (i) November 2022 to January 2023; and (ii) June to August 2023. The study followed the Good Clinical Practice guidelines and the principles of the Declaration of Helsinki (as revised in 2013). The protocol was approved by the Institutional Review Board of Chungnam National University Hospital (1, CNUH 2022-07-078; 2, CNUH 2023-04-042) and was registered prior to patient enrollment at cris.nih.go.kr (1, KCT0007668; 2, KCT0008508). Written informed consent was obtained from all enrolled participants before surgery.
Participants
These studies commonly included patients aged 20 to 80 years, American Society of Anesthesiologist (ASA) physical status classification 1 to 3, body mass index (BMI) >18.5 and <35 kg/m2 scheduled for elective unilateral pulmonary resection under general anesthesia with PVB or ITPB (study groups), or urologic procedure performed with general anesthesia (control group). Only the procedures that are planned to be performed under lateral decubitus position were included. The exclusion criteria included known hypersensitivity to the local anesthetics used in the study; pre-existing wound infection or severe inflammation; pre-existing non-minor pleural adhesion; pregnancy; previous intrathoracic surgery; limitation in the application of pulse oximeter monitoring in both hands (2nd or 3rd finger); known pathology that can manifest abnormal PI in upper extremity (e.g., arteriovenous fistula).
Design
The first pilot study (PVB) was designed as a two-arm non-randomized controlled trial. The study group was set to observe PI change after PVB intraoperatively. As the surgical position, lateral decubitus position, per se could affect the PI values in both hands, a control group was set which also adopts same surgical position intraoperatively. To meet the needs for a control group that matches the surgical position and anesthesia type of the study group, urologic procedures such as nephrectomy, radiofrequency ablation of renal mass were included.
The second pilot study (ITPB) was designed as a single arm observational study which resembles the PVB group of the prior pilot study.
Anesthesia and monitoring
Anesthesia was conducted according to a standardized institutional protocol, involving 1.5–2 mg/kg of propofol, 0.6–1 mg/kg of rocuronium, and 1–1.5 µg/kg of remifentanil for induction. Maintenance included sevoflurane or desflurane inhalation alongside a continuous infusion of remifentanil at 0.05–0.2 µg/kg/min. Inhalation agents were adjusted based on processed electroencephalogram, while remifentanil dosage was modulated to maintain blood pressure and heart rate within ±25% of baseline values. Intraoperative mechanical ventilation was set at 6–8 mL/kg of tidal volume, 0.4–1.0 of inspired oxygen fraction, with respiratory rate adjustments aimed at maintaining end-tidal carbon dioxide levels within 35–45 mmHg.
In addition to the standard ASA monitoring, disposable pulse oximeter sensors (RD rainbow SETTM-2 Neo sensor, Masimo Corp., Irvine, CA, USA) were applied to both hands (2nd or 3rd finger) and monitored distinctively via corresponding monitors. The PI values were continuously recorded at 1 Hz of frequency via a free data collection program (Vital recorder version 1.10, accessed at https://vitaldb.net, Seoul, Republic of Korea) (17).
Interventions
Following the induction of general anesthesia and the placement of a central venous line as required, blockades were conducted with the patient positioned laterally. A 22-gauge, 80 mm echogenic needle (SonoPlex Stim, Pajunk, Geisingen, Germany) was utilized along with either of two ultrasound machines (Venue Go R2, GE Healthcare, Wauwatosa, WI, USA; SonoSite SII, FUJIFILM, Bothell, WA, USA) and corresponding high-frequency linear probes. These procedures were carried out by anesthesiologists proficient in ultrasound-guided regional techniques.
PVB was performed using an in-plane transverse approach, as previously described (18). Once an optimal image was obtained, consisting of the transverse process, wedge-shaped hypoechoic paravertebral space, and parietal pleura, the needle was advanced from lateral to medial until the tip penetrated the internal intercostal membrane. Proper needle placement was confirmed by observing the downward displacement of the pleura during the injection of local anesthetic; 10 mL aliquots of 0.5% ropivacaine were administered at the T3–4, T5–6, and T7–8 intercostal levels, starting from the upper to lower levels. A protocol update was implemented on December 16, 2022, after enrolling four subjects in the control group. This update shifted from a dual injection protocol (T4–5 and T6–7) to the triple injection protocol previously described, in response to a request from the surgical department to encompass a wider range of dermatomes. This protocol change occurred after enrolling subjects in the control group, ensuring no variations in the protocol within the study groups.
ITPB was conducted using an out-of-plane approach targeting mid-point transverse process above superior costotransverse ligament (5). Proper needle placement was confirmed by observing slight bulging of the intertransverse connective tissue complex during injection; 10 mL aliquots of 0.5% ropivacaine was administered at the same intercostal levels and the sequence as the PVB.
Measurements
The primary measurement was the PI values in both hands. As the procedures were performed with lateral decubitus position, the hand of the operating side (nondependent) was placed on an arm board adjusted for the surgical exposure. To assess the impact of changes in position and blockade on PI, each time point of the events (i.e., immediately after the position change and completion of the blockades) was recorded.
Since the PI values were obtained intraoperatively rather than resting state, confounding due to general anesthesia and positioning was expected. To adjust such factors, the PI value of the operating side (PI-O) was divided by the value of contralateral side (PI-CL). Then the relative change to the baseline (during the initial 3 minutes after position change) was assessed (relative PI-O/PI-CL). A previous study reported a factor of 1.55 times baseline PI value as a sensitive and specific marker for the successful regional block (13). Based on this, a 50% increase in the adjusted value (i.e., 1.5 of relative PI-O/PI-CL) was considered a meaningful increase. A list of the processed measurements is presented in Table 1.
Table 1
Variables | Definitions |
---|---|
PI-O | PI in the upper extremity of the operating side |
PI-CL | PI in the upper extremity contralateral to the operating side |
PI-O/PI-CL | PI in the operating side compared to the contralateral (PI-O divided by PI-CL) |
Relative PI-O/PI-CL | Relative PI-O/PI-CL value compared to the baseline |
(Prefix) Baseline- | The initial 3 minutes after position change; the prefix indicates the mean value of each corresponding variable during that period |
(Prefix) Intraoperative- | The period after the baseline (control group) or after the blockade (study groups); the prefix indicates the mean value of each corresponding variable during that period |
Procedure time | Duration between the position change and the end of the procedure (block) |
Onset time | From the start of the procedure (position change) to the onset of the sympathetic blockade (50% increase of PI-O/PI-CL from the baseline, i.e., 1.5 of relative PI-O/PI-CL) |
PI, perfusion index.
In the main analysis, PI values obtained during the observation period up to one hour after the position change were used, taking into consideration the case-specific variations in the observation period. To avoid any errors from the cuff inflation during noninvasive blood pressure measurements, we excluded PI values obtained during these time periods. Specifically, PI values collected during the 20 seconds before and after the blood pressure measurements were discarded.
Sample size
The sample size of the first pilot study was based on our previous study (19). We found that the intraoperative PI after general anesthesia in non-cardiothoracic surgery was 3.1 (mean) with a standard deviation (SD) of 1.6. Assuming that intraoperative PI in thoracic surgery would be similar, with a mean of 3.0 and a SD of 1.5, we calculated that 10 subjects would be required for the study group to detect a 50% of PI difference (i.e., 1.5) (20) between two dependent means (before and after the blockade) with 80% power, two-sided significance level 5%. We planned to recruit the same number of participants for the control group. In light of the possibility of data loss, we decided to increase the sample size by adding two extra subjects per group, resulting in a total of 24 participants.
In the second pilot study, a sample size of 12 was needed to detect 50% increase in PI with 95% power and two-sided significance level 5%, based on the data of the first pilot study which showed baseline PI of 5.5±2.3. To account for possibility of data loss, sample size was increased to 14.
Statistical analysis
Statistical analyses were conducted on a per-protocol basis using R software version 4.0.3 (R Project for Statistical Computing, Vienna, Austria). Continuous variables were reported as mean ± SD or median (1Q, 3Q) based on the results of Shapiro-Wilk tests for assessing normality, and the data were analyzed using one-way analysis of variance (ANOVA) or the Kruskal-Wallis test as appropriate. Post-hoc pairwise comparisons of outcome were conducted using Dunn’s test with P value adjusted using the Bonferroni method. A two-tailed P value <0.05 was considered statistically significant.
Results
A total of 48 subjects were assessed for eligibility, 32 in the first study and 16 in the second. Eight subjects were excluded in the first study based on the exclusion criteria (n=2), lack of informed consent (n=2), and due to an unexpected error in the recording system detected prior to surgery (n=4). Two subjects were excluded in the second study based on the exclusion criteria. Five subjects were withdrawn from the analysis due to data loss. Additionally, two subjects in the ITPB group were withdrawn, one due to unexpected change in the operating schedule (which was incompatible with the research schedule), and the other due to the inability to perform the planned blockade because of poor visualization during ultrasound scanning. Ultimately, 31 subjects (10, 11, and 10 for the control, PVB, and ITPB group, respectively) were included in the final analysis (Figure 1). Clinical characteristics of the included patients are summarized in Table 2.
Table 2
Characteristics | Control (n=10) | PVB (n=11) | ITPB (n=10) | P |
---|---|---|---|---|
Sex (F) | 2 (20.0) | 2 (18.2) | 6 (60.0) | 0.07 |
Age (years) | 67.5 (57.0, 76.0) | 62.0 (56.5, 65.0) | 63.5 (61.0, 69.0) | 0.44 |
Height (cm) | 166.1±7.2 | 168.6±7.0 | 159.0±6.2 | 0.01 |
Weight (kg) | 66.9±11.4 | 64.8±6.7 | 59.4±8.4 | 0.17 |
BMI (kg/m2) | 24.3±4.7 | 22.8±1.8 | 23.5±3.1 | 0.57 |
Comorbidities | ||||
HTN | 7 (70.0) | 3 (27.3) | 4 (40.0) | 0.13 |
DM | 5 (50.0) | 1 (9.1) | 4 (40.0) | 0.11 |
CAD | 0 | 0 | 1 (10.0) | 0.33 |
CVD | 1 (10.0) | 1 (9.1) | 1 (10.0) | 0.99 |
Anesthesia duration (min) | 138.0 (120.0, 184.0) | 124.0 (109.0, 152.0) | 112.5 (99.0, 145.0) | 0.31 |
Fluid intake (mL/h) | 308.4±143.2 | 354.7±144.5 | 267.0±85.5 | 0.30 |
Remifentanil (µg/kg/min) | 0.093±0.025 | 0.069±0.013 | 0.080±0.019 | 0.03 |
Data are reported as number (%), median (1Q, 3Q), or mean ± SD. PVB, paravertebral block; ITPB, intertransverse process block; F, female; BMI, body mass index; HTN, hypertension; DM, diabetes mellitus; CAD, coronary artery disease; CVD, cerebrovascular disease; SD, standard deviation.
PI changes in representative cases in each group are shown in Figure 2 and every individual cases are shown in supplementary material (Appendix 1). The average procedure times were 10.1±3.8 and 7.6±0.9 minutes in PVB and ITPB groups, respectively. PI values of the entire subjects stratified by the group and monitoring side are shown in Figure 3. Noticeable increases of PI-O were observed from the initiation of the procedure, reaching its peak approximately 10–15 minutes later. While the relative PI-O/PI-CL value in the control group generally remained around 1—indicating a consistent ratio of PI between both sides of the upper extremity—occasional fluctuations exceeding 1.5 were observed. Consequently, we considered only the periods from the procedure’s commencement to 5 minutes after the end of blockade (during surgical draping) as valid for assessing PI changes resulting from the blockade. Significant alterations following the blockades were evident in all cases within the PVB and ITPB groups, with onset times recorded at 7.7±3.8 and 6.9±2.8 minutes, respectively.
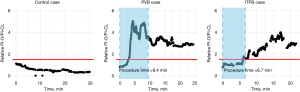
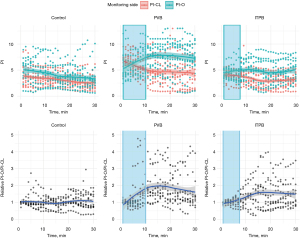
A concise summary of the measured variables is presented in Table 3. The intraoperative relative PI-O/PI-CL was 0.9 (0.8, 1.4), 2.1 (1.4, 2.5), and 1.4 (0.9, 1.9) in the control, PVB, and ITPB group, respectively (P=0.01). Pairwise comparison revealed a significant difference only between the control and PVB groups (adjusted P=0.01).
Table 3
Variables | Control (n=10) | PVB (n=11) | ITPB (n=10) | P |
---|---|---|---|---|
Procedure time (min) | NA | 10.1±3.8 | 7.6±0.9 | 0.04 |
Onset time (min) | NA | 7.7±3.8 | 6.9±2.8 | 0.61 |
Baseline PI-O | 5.1±2.2 | 5.5±2.3 | 4.7±1.1 | 0.65 |
Intraoperative PI-O | 3.5±1.5 | 6.7±2.7 | 4.5±1.7 | 0.004 |
Baseline PI-CL | 3.8±2.0 | 6.6±1.8 | 4.1±1.2 | 0.001 |
Intraoperative PI-CL | 2.8±1.1 | 4.5±2.7 | 3.0±1.1 | 0.09 |
Baseline PI-O/PI-CL | 1.5±0.8 | 0.8±0.3 | 1.3±0.5 | 0.03 |
Intraoperative PI-O/PI-CL | 1.4 (0.7, 2.0) | 1.5 (1.3, 2.4) | 1.7 (1.1, 2.4) | 0.52 |
Intraoperative relative PI-O/PI-CL | 0.9 (0.8, 1.4) | 2.1 (1.4, 2.5) | 1.4 (0.9, 1.9) | 0.01 |
Data are reported as mean ± SD or median (1Q, 3Q). PVB, paravertebral block; ITPB, intertransverse process block; NA, not available; PI, perfusion index; PI-O, PI values of the operating side; PI-CL, PI values of the contralateral side; SD, standard deviation.
Discussion
This study demonstrated increases in PI in the ipsilateral upper extremity following PVB and ITPB. The increase in PI was assessed relative to the contralateral side and baseline (relative PI-O/PI-CL). Dramatic increases in ipsilateral PI were observed in the majority of PVB cases, indicative of unilateral sympathetic blockade. On the contrary, ITPB cases exhibited less pronounced increases in PI, with some cases not showing notable changes, although all cases met the criteria for a significant increase, at least briefly. To our knowledge, this is the first study to illustrate intraoperative changes in PI after these blockades. The study also revealed that these increases in PI were not consistently sustained throughout the surgery and could occur even without any blockade. This implies that changes in PI-O/PI-CL may not always correlate exclusively with the success of the blockade but are also influenced by other factors.
To account for systemic factors prone to fluctuations induced by general anesthesia and surgical stimuli, such as sympathetic tone, hemodynamics, and temperature—factors that, in turn, affect the PI (19,21)—we implemented bilateral monitoring of PI. By observing changes in the adjusted value, PI-O/PI-CL, we could discern whether the increase in PI values was induced systematically or regionally (i.e., by the blockade). Unfortunately, however, hidden confounders seem to remain, leading to fluctuations in PI-O/PI-CL even in the absence of a blockade, as observed in the control group. Potential confounding factors may include imbalanced changes in perfusion or temperature of an extremity. This issue should be addressed in future studies with more meticulous PI monitoring and carful patient positioning. Additionally, it is advisable to carefully control for hemodynamics and surgical stimuli to accurately determine changes in PI values before and after regional blockade or surgical sympathectomy.
It is important to note that the current study was a combined study of two pilot studies aimed at observing intraoperative changes in PI with (PVB or ITPB) or without (control) regional blockades within each group. The study was conducted in a non-randomized fashion sequentially, with the first pilot study for PVB and control, followed by the second pilot study for ITPB. As these studies were not designed with a prior hypothesis specific to group comparison and power calculation, the result should be conceived as a hypothesis and framework generating measure rather than a confirmatory result. Future studies with proper sample size and randomization are needed to validate the results of the current study.
There has been a growing interest in paravertebral proxies and their underlying mechanisms that convey analgesic effects on the chest wall (1-9). Theoretically, the anterior spread of local anesthetic should occur through channels such as the costotransverse foramen to provide this analgesic effect, as supported by various cadaveric and anatomical studies (7-9). However, there is still limited evidence available from real clinical contexts. Therefore, the present study aimed to develop a clinically useful tool for the noninvasive detection of sympathetic blocks in an operating environment. This tool not only offers a way to better understand newly developed regional techniques but also addresses the scarcity of evidence in clinical settings. Furthermore, it may provide real-time intraoperative feedback for a successful surgical sympathectomy (22). Additional measures can be considered before emergence from anesthesia if expected changes are not observed after surgical sympathectomy or regional blockade.
In a previous study that reported an increase in upper extremity PI after PVB, an additional experimental setting was necessary (20). In that study, the blockade was administered before anesthesia and the surgical stimulus, with a distinct observation period of 30 minutes. Although this setup could offer more controlled data, it deviates from everyday clinical practice. In the current study, our aim was to establish a more practical approach for evaluating sympathetic blockade after paravertebral or its proxies, one that can be readily applied in clinical settings.
The majority of preganglionic fibers to the upper extremity originate from spinal segments T3 to T6, whereas postganglionic fibers to the arm stem from T2, with a lesser contribution from T3 ganglia (23). Consequently, the upper thoracic region holds significant importance in sympathetic blockade for the upper extremity (22,24). In a prior cadaveric study, PVB exhibited a more vertically extensive spread within the paravertebral space (approximately 2–4 vertebral levels), contrasting with ITPB, which displayed a more limited extent (about 1–2 vertebral levels) (25). Consequently, ITPB increases the likelihood of sparing T2 ganglia, potentially leading to an inconsistent elevation of PI in this study. Moreover, while not conclusively established, it is intuitively apparent that as an indirect injection technique (i.e., ITPB), it tends to induce a lesser degree of sympathetic block compared to a direct injection (i.e., PVB).
The rationale for employing the multiple injection technique in this study was drawn from existing literature on PVB, which indicated a variable and unpredictable distribution following a single injection (26,27). Despite some evidence supporting the efficacy of the single injection technique (28,29), we opted for multiple injections due to the current lack of reliability in single injection methods for ITPB in thoracic surgery. By standardizing block levels in both PVB and ITPB, our emphasis was on the ventral spread of local anesthetic rather than its vertical distribution across multiple dermatomal levels. Notably, we set the uppermost target at T3, as areas beyond this level are considered less relevant for achieving analgesia in thoracic surgery.
This study presents several limitations. Firstly, the success of blockade was solely determined based on sonographic endpoints, such as the downward movement of the pleura or bulging of the intertransverse tissue complex during injection. Future studies would benefit from a more comprehensive evaluation, including detailed assessments of dermatomal coverage and postoperative pain scores. Secondly, despite adjustments for the contralateral side and baseline, the newly derived tool (i.e., relative PI-O/PI-CL) may not exclusively reflect the success of the blockade. A more stable and controlled environment, especially in the immediate pre- and post-blockade phases, would yield more reliable results. Thirdly, since three levels of blockades were conducted in this study, the distinct contribution of each level to changes in PI cannot be analyzed. Determining the success of sympathetic blockade for lower thoracic levels based on upper extremity PI may be misleading. Fourthly, we were unable to include a true control group in thoracic procedures. Since incorporating a regional blockade as part of multimodal analgesia is a widely accepted standard, omitting a blockade for a research purpose was clinically unfeasible. Instead, we included a urologic procedure group as a control group, which currently lacks routine regional blockade for analgesia.
Conclusions
In conclusion, the study suggests that PVB results in a noticeable unilateral increase in upper extremity PI, whereas ITPB tends to induce a more inconsistent and lesser degree of increase. While monitoring PI values can serve as an indicator of upper extremity sympathetic blockade, it is essential to acknowledge potential confounders during surgery that may impact these observations. It is important to emphasize that the present study serves as a preliminary exploration, and further research, particularly through randomized trials, is needed to validate these findings conclusively.
Acknowledgments
Funding: This research was supported by research funds from
Footnote
Reporting Checklist: The authors have completed the TREND reporting checklist. Available at https://jtd.amegroups.com/article/view/10.21037/jtd-24-69/rc
Data Sharing Statement: Available at https://jtd.amegroups.com/article/view/10.21037/jtd-24-69/dss
Peer Review File: Available at https://jtd.amegroups.com/article/view/10.21037/jtd-24-69/prf
Conflicts of Interest: All authors have completed the ICMJE uniform disclosure form (available at https://jtd.amegroups.com/article/view/10.21037/jtd-24-69/coif). The authors have no conflicts of interest to declare.
Ethical Statement: The authors are accountable for all aspects of the work in ensuring that questions related to the accuracy or integrity of any part of the work are appropriately investigated and resolved.
Open Access Statement: This is an Open Access article distributed in accordance with the Creative Commons Attribution-NonCommercial-NoDerivs 4.0 International License (CC BY-NC-ND 4.0), which permits the non-commercial replication and distribution of the article with the strict proviso that no changes or edits are made and the original work is properly cited (including links to both the formal publication through the relevant DOI and the license). See: https://creativecommons.org/licenses/by-nc-nd/4.0/.
References
- Ohgoshi Y, Usui Y, Ando A, et al. Injection at the costotransverse notch facilitates paravertebral spread of the erector spinae plane block: A cadaveric study. J Clin Anesth 2020;61:109630. [Crossref] [PubMed]
- Nielsen MV, Moriggl B, Hoermann R, et al. Are single-injection erector spinae plane block and multiple-injection costotransverse block equivalent to thoracic paravertebral block? Acta Anaesthesiol Scand 2019;63:1231-8. [Crossref] [PubMed]
- Murouchi T, Yamakage M. Retrolaminar block: analgesic efficacy and safety evaluation. J Anesth 2016;30:1003-7. [Crossref] [PubMed]
- Kilicaslan A, Sarkilar G, Altınok T, et al. A novel ultrasound-guided technique in peri-paravertebral area: Subtransverse process interligamentary (STIL) plane block: The game has not ended yet. J Clin Anesth 2020;60:76-7. [Crossref] [PubMed]
- Costache I, de Neumann L, Ramnanan CJ, et al. The mid-point transverse process to pleura (MTP) block: a new end-point for thoracic paravertebral block. Anaesthesia 2017;72:1230-6. [Crossref] [PubMed]
- Costache I, Pawa A, Abdallah FW. Paravertebral by proxy - time to redefine the paravertebral block. Anaesthesia 2018;73:1185-8. [Crossref] [PubMed]
- Bonvicini D, Boscolo-Berto R, De Cassai A, et al. Anatomical basis of erector spinae plane block: a dissection and histotopographic pilot study. J Anesth 2021;35:102-11. [Crossref] [PubMed]
- Cho TH, Kim SH. Anatomy of the thoracic paravertebral space: 3D micro-CT findings and their clinical implications for nerve blockade. Reg Anesth Pain Med 2021;46:699-703. [Crossref] [PubMed]
- Cho TH, Kwon HJ. The pathway of injectate spread during thoracic intertransverse process (ITP) block: Micro-computed tomography findings and anatomical evaluations. J Clin Anesth 2022;77:110646. [Crossref] [PubMed]
- Rozen WM, Chubb D, Stella DL, et al. Evaluating anatomical research in surgery: a prospective comparison of cadaveric and living anatomical studies of the abdominal wall. ANZ J Surg 2009;79:913-7. [Crossref] [PubMed]
- Chin KJ, El-Boghdadly K. Mechanisms of action of the erector spinae plane (ESP) block: a narrative review. Can J Anaesth 2021;68:387-408. [Crossref] [PubMed]
- Chung K, Kim KH, Kim ED. Perfusion index as a reliable parameter of vasomotor disturbance in complex regional pain syndrome. Br J Anaesth 2018;121:1133-7. [Crossref] [PubMed]
- Galvin EM, Niehof S, Verbrugge SJ, et al. Peripheral flow index is a reliable and early indicator of regional block success. Anesth Analg 2006;103:239-43. table of contents. [Crossref] [PubMed]
- Ginosar Y, Weiniger CF, Meroz Y, et al. Pulse oximeter perfusion index as an early indicator of sympathectomy after epidural anesthesia. Acta Anaesthesiol Scand 2009;53:1018-26. [Crossref] [PubMed]
- Huang B, Sun K, Zhu Z, et al. Oximetry-derived perfusion index as an early indicator of CT-guided thoracic sympathetic blockade in palmar hyperhidrosis. Clin Radiol 2013;68:1227-32. [Crossref] [PubMed]
- Yamazaki H, Nishiyama J, Suzuki T. Use of perfusion index from pulse oximetry to determine efficacy of stellate ganglion block. Local Reg Anesth 2012;5:9-14. [Crossref] [PubMed]
- Lee HC, Jung CW. Vital Recorder-a free research tool for automatic recording of high-resolution time-synchronised physiological data from multiple anaesthesia devices. Sci Rep 2018;8:1527. [Crossref] [PubMed]
- Shibata Y, Nishiwaki K. Ultrasound-guided intercostal approach to thoracic paravertebral block. Anesth Analg 2009;109:996-7. [Crossref] [PubMed]
- Oh C, Lee S, Song BS, et al. Comparative effects of desflurane and sevoflurane on intraoperative peripheral perfusion index: a retrospective, propensity score matched, cohort study. Sci Rep 2023;13:2991. [Crossref] [PubMed]
- Tugcugil E, Besir A. Perfusion index as an objective, simple and quick parameter for determining the success of paravertebral block. Niger J Clin Pract 2021;24:115-20. [Crossref] [PubMed]
- Coutrot M, Dudoignon E, Joachim J, et al. Perfusion index: Physical principles, physiological meanings and clinical implications in anaesthesia and critical care. Anaesth Crit Care Pain Med 2021;40:100964. [Crossref] [PubMed]
- Jeng EI, Gravenstein N, Klodell CT. Perfusion Index: An Indicator of Success During Endoscopic Thoracic Sympathectomy for Hyperhidrosis. Ann Thorac Surg 2017;104:426-30. [Crossref] [PubMed]
- Schiller Y. The anatomy and physiology of the sympathetic innervation to the upper limbs. Clin Auton Res 2003;13:I2-5. [Crossref] [PubMed]
- Reisfeld R. Sympathectomy for hyperhidrosis: should we place the clamps at T2-T3 or T3-T4? Clin Auton Res 2006;16:384-9. [Crossref] [PubMed]
- Varela V, Ruíz C, Montecinos S, et al. Spread of local anesthetic injected in the paravertebral space, intertransverse processes space, and erector spinae plane: a cadaveric model. Reg Anesth Pain Med 2024;49:228-32. [Crossref] [PubMed]
- Cheema S, Richardson J, McGurgan P. Factors affecting the spread of bupivacaine in the adult thoracic paravertebral space. Anaesthesia 2003;58:684-7. [Crossref] [PubMed]
- Naja ZM, El-Rajab M, Al-Tannir MA, et al. Thoracic paravertebral block: influence of the number of injections. Reg Anesth Pain Med 2006;31:196-201. [Crossref] [PubMed]
- Kaya FN, Turker G, Mogol EB, et al. Thoracic paravertebral block for video-assisted thoracoscopic surgery: single injection versus multiple injections. J Cardiothorac Vasc Anesth 2012;26:90-4. [Crossref] [PubMed]
- Saito T, Den S, Cheema SP, et al. A single-injection, multi-segmental paravertebral block-extension of somatosensory and sympathetic block in volunteers. Acta Anaesthesiol Scand 2001;45:30-3. [Crossref] [PubMed]