Thoracic cavity remodeling and pulmonary function change after chest wall resection
Highlight box
Key findings
• There was a significant long-term decrease in pulmonary function after chest wall resection (CWR) with resection of ≥3 ribs. Thoracic cavity volume (TCV) change partly contributed to this decrease.
What is known and what is new?
• A reduction in pulmonary function has been established when resecting ≥3 ribs or when the diameter of the chest wall resected is ≥5 cm.
• We investigated the long-term changes in TCV and pulmonary function after CWR. The effects of CWR extent and location and chest wall reconstruction were also analyzed.
What is the implication, and what should change now?
• A long-term decrease of pulmonary function after CWR was evident, especially after resection of ≥3 ribs, left-side chest wall, mid-level ribs, and lateral chest wall. The degree of pulmonary function decrease was similar to that observed after lung segmentectomy. These findings should be considered during patient selection, chest wall reconstruction, and long-term patient management.
Introduction
Chest wall resection (CWR) is an essential procedure for the treatment of primary or metastatic malignancies and infectious conditions of the chest wall (1). Even though radiotherapy with or without chemotherapy has been proposed as an alternative for the management of chest wall malignancies, surgical resection is currently the primary curative option (1,2). Extensive CWR is inevitable in some cases because wide resection with margins of up to 4 cm is recommended in malignancies and extensive extirpation of infected tissue is recommended in cases of infections resistant to medical treatment (3,4).
The chest wall plays an important role in respiration along with the lung, diaphragm, and intraabdominal pressure (5,6). Normal respiratory physiology involves the coordinated effort of the chest wall, diaphragm, and lungs to facilitate the exchange of oxygen and carbon dioxide. The mechanics of the chest, including the movement of the rib cage and diaphragm during inhalation and exhalation, play a crucial role in maintaining adequate ventilation and gas exchange. CWR can result in shrinkage of the remaining chest wall, subsequent decrease in thoracic cavity volume (TCV) due to loss of stability, and can also decrease the range of motion during respiration owing to the loss of accessory respiratory muscles and chest wall flexibility (7). Research on the impact of morphological changes following CWR on the reduction of TCV has not been well studied yet. The short-term effects of CWR on pulmonary function and postoperative respiratory complications have been well documented, and chest wall reconstruction is recommended in case of resection of ≥3 ribs, chest wall resected diameter ≥5 cm or with expected chest wall instability even with small defects (8,9). Meanwhile, the long-term effects of CWR have not been getting much attention. Few studies have investigated the long-term effects of CWR in a small number of heterogeneous patients, with controversial results (2,10,11). Thus, we investigated the long-term changes in TCV and pulmonary function after CWR. The effects of CWR extent and location and chest wall reconstruction were also analyzed. We present this article in accordance with the STROBE reporting checklist (available at https://jtd.amegroups.com/article/view/10.21037/jtd-24-25/rc).
Methods
Ethical statement
The study was conducted in accordance with the Declaration of Helsinki (as revised in 2013). The study was approved by the Institutional Review Board of Seoul National University Hospital (approval No. 2204-145-1319) and individual consent for this retrospective analysis was waived.
Patients
This retrospective study included adult patients who underwent CWR, with or without reconstruction, between January 2001 and June 2021 (Figure 1). CWR was defined as resection of the bony thorax with or without soft tissue. Patients who had (I) resection of a single rib, soft tissue only, the sternum, clavicle, vertebra, or abdominal wall; (II) resection beyond a single pulmonary wedge; (III) preoperative radiotherapy, and (IV) low-resolution computed tomography (CT) unable to fulfill the requirements of the reconstruction program; and (V) who underwent repeat surgeries were excluded. Ultimately, 45 patients were included in this study.
Operation
The standard surgical procedure during the study period in our institution was en bloc wide resection with a 2 to 4 cm resection margin in malignancies, or en bloc resection including healthy tissues in infectious conditions. If there was any suspicion of soft tissue or skin invasion, the surrounding soft tissues were resected together, and full-thickness CWR was performed if necessary. Chest wall reconstruction was considered based on traditional recommendations (defect diameter ≥5 cm or resection of ≥3 ribs) case by case. Skeletal reconstruction was performed primarily with expanded polytetrafluoroethylene (ePTFE) patches, and rigid materials were restricted to selected patients. Pedicled muscle or myocutaneous flaps were used to reconstruct large soft-tissue defects (12).
Pulmonary function test (PFT)
The PFT was performed using a spirometer (MedGraphics, St. Paul, Minnesota, USA) according to American Thoracic Society standards (13). PFT measurements consisted of forced expiratory volume in 1 second (FEV1, %), forced vital capacity (FVC, %), FEV1/FVC (%), and diffusing capacity of the lung for carbon monoxide (DLCO, %). PFTs were performed within 1 month preoperatively, and 12±2 months postoperatively; the latter only at the surgeon’s discretion, considering the patients’ respiratory symptoms or underlying pulmonary disease.
CT volumetric analysis
The 3-dimension (3D) image analysis platform (SYNAPSE VINCENT, Fuji Film Co., Ltd., Tokyo, Japan) was used for 3D reconstruction of CT images and TCV calculation (14). After manual designation of minor and major fissures of each lung, right and left TCVs (RCV, LCV) were measured. The TCV was defined as the sum of the RCV and LCV (Figure 2). Perioperative change of TCV (∆) was calculated in percent of change. CT volumetric analysis was conducted by one author (S.Y.B.).
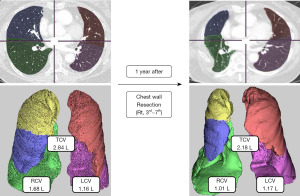
Statistical analysis
All data were analyzed using IBM SPSS ver. 25.0 (IBM Corp., Armonk, NY, USA). Categorical variables are expressed as absolute numbers and relative frequencies. We used the Wilcoxon signed-rank test to compare the significant differences in physiological changes between pre- and postoperative CWR. The Spearman rank correlation coefficient (rho, r) was used to analyze the correlation between the PFT parameters and TCV. A P value of <0.05 was considered statistically significant.
Subgroup analysis was performed according to the laterality (right, left), CWR level (upper, 1st to 4th rib; middle, 4th to 7th rib; lower, 7th to 10th rib), CWR part (anterior, sternal border to anterior axillary line; lateral, anterior to posterior axillary line; posterior, posterior axillary line to rib head), number of resected ribs (2 or ≥3), and reconstruction status (reconstruction/no-reconstruction). For level grouping, nine cases with resected areas evenly distributed across two levels were excluded (e.g., ribs 3rd to 5th or 6th to 8th). Part of the CWR was determined by the location of the center of the resected area. The effect of the reconstruction was analyzed in patients with the longest diameter of the resected chest wall ≥5 cm (n=43, 95.6%).
Results
Patients and operations
The characteristics of the study population are summarized in Table 1. The indications for surgery were primary malignant tumors in 16 (35.6%), metastatic cancer in 17 (37.8%), and infectious conditions in 12 (26.7%) patients. Full-thickness CWR was performed in two (4.4%) patients.
Table 1
Variables | Values (n=45) |
---|---|
Sex | |
Male | 34 (75.6) |
Female | 11 (24.4) |
Age (years) | 55.5±15.1 |
Chronic obstructive pulmonary disease | 4 (8.9) |
Indications for chest wall resection | |
Primary malignancies | 16 (35.6) |
Metastases | 17 (37.8) |
Infections | 12 (26.7) |
Right-side chest wall resection | 27 (60.0) |
Number of resected ribs | |
2 | 16 (35.6) |
3 | 21 (46.7) |
4 | 7 (15.6) |
5 | 1 (2.2) |
Reconstruction after chest wall resection | 30 (66.7) |
Synthetic patch | 24 (80) |
Myocutaneous or muscle flap | 6 (20) |
Wedge resection of lung | 4 (8.9) |
Except for age, which is presented as mean ± standard deviation, all other data are presented as absolute numbers (percentages).
The mean size of the resected lesion was 11.4±4.1 cm transversally and 7.5±3.2 cm vertically. The number of resected ribs was 2 in 16 (35.6%), 3 in 21 (46.7%), 4 in 7 (15.6%), and 5 in 1 (2.2%) patient. Among 43 (95.6%) patients who had ≥5 cm CWR, 30 (66.7%) had chest wall reconstruction, mostly with an ePTFE patch (n=24). Four patients (8.9%) underwent concomitant wedge resection of the lung (Table 1).
Short-term mechanical ventilation was necessary for one patient. Eight patients (17.8%) experienced complications, including wound infection (n=3), atelectasis (n=2), pneumothorax (n=1), pneumonia (n=1), and atrial fibrillation (n=1). Wound infection occurred exclusively in the patients who underwent reconstruction using a synthetic patch. No patients required oxygen at discharge.
DLCO was available for 22 patients (48.9%). TCV was calculated for all patients.
Long-term changes in PFT and TCV
One year after CWR, FVC (∆=−7.9%, P=0.004) and FEV1 (∆=−7.0%, P=0.002) had significantly decreased. However, changes in FEV1/FVC (∆=−3.0%, P=0.06), DLCO (∆=−5.9%, P=0.18) and TCV (∆=−3.1%, P=0.10) were not significant (Figure 3A, Table 2). There was no correlation between the changes in PFT parameters and those in TCV (Figure 3B). The correlation coefficient between change in TCV and change in DLCO was 0.09 (P=0.68).
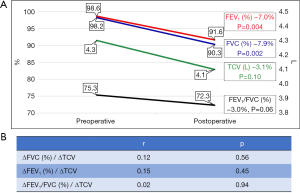
Table 2
Variables | Preoperative | Postoperative | ∆ (%) | P |
---|---|---|---|---|
Pulmonary function (n=35) | ||||
FVC (%) | 98.2 (91.3, 105.8) | 90.3 (84.5, 97.5) | −7.9 (−13.0, 0.0) | 0.004 |
FEV1 (%) | 98.6 (95.3, 107.5) | 91.6 (85.0, 102.5) | −7.0 (−13.0, −2.0) | 0.002 |
FEV1/FVC (%) | 75.3 (71.3, 80.5) | 72.3 (71.0, 78.8) | −3.0 (−5.0, 2.0) | 0.06 |
DLCO (%) | 81.7 (74.5, 88.0) | 75.8 (66.0, 88.5) | −5.9 (−9.0, 0.0) | 0.18 |
TCV (n=45) | ||||
RCV (L) | 2.3 (1.8, 2.7) | 2.2 (1.8, 2.6) | −4.1 (−14.3, 4.6) | 0.04 |
LCV (L) | 1.9 (1.6, 2.3) | 1.9 (1.5, 2.3) | −1.8 (−13.0, 2.1) | 0.28 |
TCV (L) | 4.3 (3.6, 5.0) | 4.1 (3.4, 4.8) | −3.1 (−9.2, 4.4) | 0.10 |
Data are presented as median (interquartile range). TCV, thoracic cavity volume; FVC, forced vital capacity; FEV1, forced expiratory volume in 1 second; DLCO, diffusing capacity of the lung for carbon monoxide; RCV, right TCV; LCV, left TCV.
Effect of laterality
Right-side surgery (n=27; 60.0%) was more common than left-side surgery (n=18; 40.0%). Regardless of CWR laterality, FVC and FEV1 significantly decreased. The degrees of decrease in FVC and FEV1 were larger after left CWR. After right-side CWR, the RCV decreased significantly, whereas LCV showed an increasing trend. Similarly, the LCV decreased significantly, whereas the RCV increased after left-side CWR. The tendency of TCV to decrease was more prominent after right CWR (Table 3).
Table 3
Variables | Preoperative | Postoperative | ∆ (%) | P |
---|---|---|---|---|
Right (n=27) | ||||
FVC (%) | 96.4 (91.5, 103.0) | 90.3 (85.0, 95.5) | −6.1 (−15.0, 2.0) | 0.03 |
FEV1 (%) | 96.0 (92.5, 105.5) | 90.7 (85.0, 102.0) | −5.3 (−11.5, −2.0) | 0.047 |
FEV1/FVC (%) | 74.9 (72.0, 80.0) | 72.2 (71.0, 78.0) | −2.7 (−4.5, 1.5) | 0.12 |
DLCO (%) | 80.3 (74.5, 84.0) | 74.3 (66.0, 83.8) | −6.0 (−8.5, 0.5) | 0.35 |
RCV (L) | 2.3 (1.9, 2.5) | 2.1 (1.7, 2.3) | −7.8 (−13.9, 0.6) | 0.01 |
LCV (L) | 2.1 (1.7, 2.4) | 2.1 (1.7, 2.4) | +2.1 (−5.5, 14.5) | 0.58 |
TCV (L) | 4.4 (3.8, 4.9) | 4.2 (3.5, 4.9) | −6.4 (−10.2, 7.4) | 0.40 |
Left (n=18) | ||||
FVC (%) | 100.9 (91.3, 105.8) | 90.4 (85.3, 103.8) | −10.5 (−13.0, −3.5) | 0.01 |
FEV1 (%) | 102.5 (95.5, 109.8) | 92.9 (81.8, 104.8) | −9.6 (−13.0, −5.0) | 0.01 |
FEV1/FVC (%) | 75.9 (70.5, 82.0) | 73.5 (66.0, 78.8) | −2.4 (−4.8, 1.5) | 0.26 |
DLCO (%) | 84.3 (67.0, 104.0) | 78.7 (66.0, 96.5) | −5.6 (−8.5, −1.0) | 0.18 |
RCV (L) | 2.3 (1.8, 2.9) | 2.3 (1.9, 2.8) | +1.3 (−5.5, 7.0) | 0.85 |
LCV (L) | 1.8 (1.3, 2.3) | 1.6 (1.2, 2.1) | −8.5 (−16.3, −2.2) | 0.004 |
TCV (L) | 4.1 (3.1, 5.0) | 4.0 (3.1, 4.8) | −3.0 (−9.0, 0.3) | 0.13 |
Data are presented as median (interquartile range). TCV, thoracic cavity volume; FVC, forced vital capacity; FEV1, forced expiratory volume in 1 s; DLCO, diffusing capacity of the lung for carbon monoxide; RCV, right TCV; LCV, left TCV.
Effect of the level of CWR
The CWR level was upper in 6 (13.3%), mid in 19 (42.2%), and lower in 11 (24.4%) patients. Although all PFT parameters showed a decreasing trend, FVC (∆=−11.9%, P=0.03) and FEV1 (∆=−13.1%, P=0.02) significantly decreased only in the mid group. The TCV tended to decrease at any level of the operation; however, the difference was only significant in the upper-level group (Figure 4A, Table 4). There was no correlation between the changes in PFT parameters and the changes in TCV in any group (Table 5).
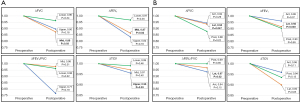
Table 4
Variables | Preoperative | Postoperative | ∆ (%) | P |
---|---|---|---|---|
Level: upper (n=6) | ||||
FVC (%) | 107.5 (105.8, 109.3) | 96.5 (92.8, 100.3) | −11.0 (−13.0, −9.0) | 0.18 |
FEV1 (%) | 100.0 (98.5, 101.5) | 83.5 (81.8, 85.3) | −16.5 (−19.8, −13.3) | 0.18 |
FEV1/FVC (%) | 79.5 (75.8, 83.3) | 61.0 (59.5, 62.5) | −18.5 (−23.8, −13.3) | 0.18 |
TCV (L) | 4.0 (3.5, 4.1) | 3.8 (3.4, 4.1) | −4.4 (−4.8, −1.1) | 0.03 |
Level: mid (n=19) | ||||
FVC (%) | 95.3 (89.3, 104.0) | 83.4 (83.0, 91.5) | −11.9 (−15.5, −7.5) | 0.03 |
FEV1 (%) | 97.8 (93.0, 100.8) | 84.6 (77.5, 96.5) | −13.1 (−18.5, −6.5) | 0.02 |
FEV1/FVC (%) | 74.5 (71.8, 76.8) | 72.8 (71.8, 74.5) | −1.8 (−4.0, 0.3) | 0.31 |
DLCO (%) | 93.6 (74.0, 101.0) | 74.5 (64.3, 84.8) | −19.1 (−24.0, 5.0) | 0.66 |
TCV (L) | 4.1 (2.8, 5.1) | 4.0 (3.1, 4.8) | −2.9 (−12.2, 5.7) | 0.40 |
Level: lower (n=11) | ||||
FVC (%) | 101.5 (98.3, 105.8) | 97.8 (94.3, 105.3) | −3.7 (−7.3, −0.8) | 0.34 |
FEV1 (%) | 106.6 (103.0, 109.8) | 103.2 (100.3, 109.3) | −3.4 (−10.3, −1.3) | 0.35 |
FEV1/FVC (%) | 80.5 (77.3, 83.5) | 77.5 (72.5, 79.0) | −3.0 (−4.0, 2.0) | 0.22 |
DLCO (%) | 91.5 (82.0, 96.5) | 86.0 (81.3, 92.8) | −5.5 (−10.5, 0.5) | 0.66 |
TCV (L) | 4.3 (3.7, 4.8) | 4.3 (3.9, 5.0) | −0.2 (−1.1, 1.1) | 0.46 |
Part: anterior (n=20) | ||||
FVC (%) | 98.4 (91.5, 107.0) | 92.9 (88.5, 103.5) | −5.5 (−12.5, 3.0) | 0.29 |
FEV1 (%) | 97.4 (92.0, 105.5) | 93.5 (87.0, 102.0) | −3.9 (−12.5, 2.0) | 0.37 |
FEV1/FVC (%) | 74.5 (70.5, 79.0) | 69.7 (65.0, 73.0) | −4.8 (−8.0, 0.5) | 0.15 |
DLCO (%) | 75.0 (72.0, 86.0) | 73.3 (66.3, 87.5) | −1.7 (−3.3, 0.5) | 0.41 |
TCV (L) | 4.2 (3.6, 4.8) | 4.0 (3.4, 4.6) | −4.1 (−6.5, 3.5) | 0.11 |
Part: lateral (n=15) | ||||
FVC (%) | 97.9 (90.0, 105.0) | 91.6 (85.0, 96.0) | −6.3 (−11.8, −1.3) | 0.047 |
FEV1 (%) | 103.4 (97.0, 110.5) | 94.7 (85.0, 102.5) | −8.7 (−11.8, −4.0) | 0.003 |
FEV1/FVC (%) | 79.2 (75.0, 82.5) | 76.6 (71.5, 79.0) | −2.8 (−4.0, 0.8) | 0.02 |
DLCO (%) | 86.8 (73.8, 90.5) | 84.3 (71.5, 97.3) | −2.5 (−6.8, 0.0) | 0.72 |
TCV (L) | 4.1 (3.6, 4.7) | 4.1 (3.6, 4.7) | −1.0 (−7.5, 5.5) | 0.65 |
Part: posterior (n=10) | ||||
FVC (%) | 92.8 (84.0, 99.0) | 81.6 (74.0, 95.0) | −11.2 (−25.0, −4.0) | 0.22 |
FEV1 (%) | 87.0 (83.0, 102.0) | 78.6 (53.0, 101.0) | −8.4 (−6.0, −2.0) | 0.04 |
FEV1/FVC (%) | 67.8 (73.0, 77.0) | 68.0 (66.0, 79.0) | +0.2 (−1.0, 2.0) | 0.85 |
DLCO (%) | 83.3 (78.5, 87.0) | 66.7 (63.0, 73.0) | −16.6 (−23.0, −5.5) | 0.11 |
TCV (L) | 4.5 (3.8, 5.7) | 4.2 (2.8, 5.4) | −6.5 (−17.3, 0.3) | 0.13 |
Data are presented as median (interquartile range). TCV, thoracic cavity volume; FVC, forced vital capacity; FEV1, forced expiratory volume in 1 second; DLCO, diffusing capacity of the lung for carbon monoxide.
Table 5
Variables | r | P |
---|---|---|
Level: upper (n=6) | ||
∆FVC (%)/∆TCV | 1.00 | – |
∆FEV1 (%)/∆TCV | 1.00 | – |
∆FEV1/FVC (%)/∆TCV | 1.00 | – |
Level: mid (n=19) | ||
∆FVC (%)/∆TCV | 0.23 | 0.63 |
∆FEV1 (%)/∆TCV | 0.26 | 0.57 |
∆FEV1/FVC (%)/∆TCV | 0.08 | 0.87 |
∆DLCO (%)/∆TCV | 1.00 | – |
Level: lower (n=11) | ||
∆FVC (%)/∆TCV | 0.19 | 0.59 |
∆FEV1 (%)/∆TCV | 0.29 | 0.42 |
∆FEV1/FVC (%)/∆TCV | −0.25 | 0.48 |
∆DLCO (%)/∆TCV | 0.03 | 0.97 |
Part: anterior (n=20) | ||
∆FVC (%)/∆TCV | 0.01 | 0.98 |
∆FEV1 (%)/∆TCV | 0.29 | 0.38 |
∆FEV1/FVC (%)/∆TCV | −0.11 | 0.76 |
∆DLCO (%)/∆TCV | 0.99 | 0.07 |
Part: lateral (n=15) | ||
∆FVC (%)/∆TCV | 0.81 | 0.049 |
∆FEV1 (%)/∆TCV | 0.87 | 0.02 |
∆FEV1/FVC (%)/∆TCV | 0.11 | 0.83 |
∆DLCO (%)/∆TCV | −0.30 | 0.56 |
Part: posterior (n=10) | ||
∆FVC (%)/∆TCV | −0.19 | 0.77 |
∆FEV1 (%)/∆TCV | 0.46 | 0.44 |
∆FEV1/FVC (%)/∆TCV | 0.79 | 0.11 |
∆DLCO (%)/∆TCV | 0.61 | 0.27 |
TCV, thoracic cavity volume; FVC, forced vital capacity; FEV1, forced expiratory volume in 1 second; DLCO, diffusing capacity of the lung for carbon monoxide.
Effect of the part of CWR
The CWR was anterior in 20 (44.4%), lateral in 15 (33.3%), and posterior in 10 (22.2%) patients. In the lateral group, FVC (∆=−6.3%, P=0.047), FEV1 (∆=−8.7%, P=0.003), and FEV1/FVC (∆=−2.8%, P=0.02) decreased significantly, but the decrease in TCV was less prominent. FEV1 (∆=−8.4%, P=0.04) decreased significantly in the posterior group, while there was no significant change in PFT in the anterior group. There was no significant change in TCV across the board (Figure 4B, Table 4). There was a positive correlation between the changes in FVC (r=0.81, P=0.049), FEV1 (r=0.87, P=0.02), and TCV in the lateral group (Table 5).
Effect of the number of resected ribs and reconstruction
The number of resected ribs was 2 in 16 (35.6%), and ≥3 in 29 (64.4%) patients. The changes in PFT parameters and TCV were not significant in the 2-rib resection group. Conversely, FVC (∆=−9.5%, P=0.02), FEV1 (∆=−7.9%, P=0.02) and TCV (∆=−6.4%, P=0.04) significantly decreased in ≥3-rib resection group (Figure 5A, Table 6).
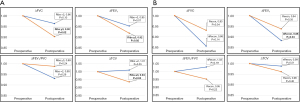
Table 6
Variables | Preoperative | Postoperative | ∆ (%) | P |
---|---|---|---|---|
Resected ribs =2 (n=16) | ||||
FVC (%) | 101.4 (98.5, 105.5) | 97.7 (94.5, 101.0) | −3.7 (−5.5, −1.5) | 0.10 |
FEV1 (%) | 103.9 (99.5, 107.5) | 98.9 (98.0, 102.0) | −5.0 (−9.0, −1.5) | 0.07 |
FEV1/FVC (%) | 79.6 (77.5, 81.5) | 74.1 (73.5, 79.0) | −5.5 (−4.0, 0.0) | 0.29 |
DLCO (%) | 94.8 (78.0, 98.8) | 85.3 (71.7, 94.6) | −9.5 (−12.1, −3.5) | 0.09 |
TCV (L) | 4.3 (3.5, 4.9) | 4.3 (3.7, 5.2) | +1.0 (−4.1, 7.6) | 0.55 |
Resected ribs ≥3 (n=29) | ||||
FVC (%) | 96.9 (89.5, 105.8) | 87.4 (80.3, 94.5) | −9.5 (−16.5, 1.5) | 0.02 |
FEV1 (%) | 96.6 (87.5, 107.3) | 88.7 (81.3, 104.0) | −7.9 (−13.0, −2.5) | 0.02 |
FEV1/FVC (%) | 73.6 (70.0, 79.0) | 72.2 (67.3, 77.8) | −1.4 (−5.0, 2.0) | 0.24 |
DLCO (%) | 81.7 (74.0, 89.0) | 75.8 (64.0, 89.0) | −5.9 (−9.0, 0.0) | 0.18 |
TCV (L) | 4.3 (3.7, 5.1) | 4.1 (3.2, 4.8) | −6.4 (−12.0, 2.6) | 0.04 |
No-reconstruction (n=13) | ||||
FVC (%) | 99.0 (92.0, 105.0) | 84.8 (86.0, 90.0) | −14.2 (−20.0, −3.0) | 0.14 |
FEV1 (%) | 95.6 (89.0, 105.0) | 84.4 (85.0, 87.0) | −11.2 (−13.0, −8.0) | 0.04 |
FEV1/FVC (%) | 71.2 (69.0, 78.0) | 73.2 (71.0, 77.0) | +2.0 (−4.0, 2.0) | 0.79 |
DLCO (%) | 63.0 (54.0, 72.0) | 58.5 (50.8, 66.3) | −4.5 (−5.8, −3.3) | 0.18 |
TCV (L) | 4.3 (3.6, 5.1) | 4.3 (3.3, 5.0) | −1.2 (−6.7, 2.6) | 0.51 |
Reconstruction (n=30) | ||||
FVC (%) | 98.4 (91.5, 105.5) | 91.6 (84.0, 100.5) | −6.8 (−13.0, 0.0) | 0.34 |
FEV1 (%) | 99.3 (95.5, 109.0) | 93.1 (86.0, 105.0) | −6.2 (−12.0, −1.5) | 0.35 |
FEV1/FVC (%) | 76.3 (71.5, 82.5) | 72.6 (68.0, 79.0) | −3.7 (−5.0, 1.5) | 0.22 |
DLCO (%) | 89.2 (78.3, 92.0) | 78.3 (66.0, 88.5) | −10.9 (−12.0, 0.0) | 0.66 |
TCV (L) | 4.3 (3.6, 4.9) | 4.1 (3.4, 4.7) | −3.8 (−9.2, 4.1) | 0.46 |
Data are presented as median (interquartile range). TCV, thoracic cavity volume; FVC, forced vital capacity; FEV1, forced expiratory volume in 1 second; DLCO, diffusing capacity of the lung for carbon monoxide.
The size of the resected lesion was larger in the reconstruction group than in the no-reconstruction group (transverse length: 11.9±3.7 vs. 10.7±3.4 cm, P=0.30; vertical length: 8.4±2.9 vs. 5.9±2.9 cm, P=0.02). There was no difference in distributions of level (P=0.70) and part (P=0.51) between the reconstruction group and no-reconstruction group. There was no significant change in PFT parameters and TCV in both groups, except for a FEV1 (∆=−11.2%, P=0.04) decrease in the no-reconstruction group (Figure 5B, Table 6). No significant differences in the changes in PFT parameters and TCV were noted between the reconstruction and no-reconstruction groups. There was no difference in change of TCV and PFT parameters among the prosthesis reconstruction subgroup and non-prosthesis reconstruction subgroup (Table 7).
Table 7
Variables | Preoperative | Postoperative | ∆ (%) | P |
---|---|---|---|---|
Non-prosthesis reconstruction (n=6) | ||||
FVC (%) | 101.8 (98.0, 106.0) | 101.8 (98.0, 106.0) | 0.0 (−5.0, 0.0) | >0.99 |
FEV1 (%) | 105.6 (97.0, 108.0) | 106.2 (104.0, 113.0) | +0.6 (−4.0, 3.0) | 0.93 |
FEV1/FVC (%) | 85.4 (82.0, 93.0) | 75.4 (71.0, 79.0) | −10.0 (−22.0, 2.0) | 0.24 |
DLCO (%) | 102.0 (93.5, 110.5) | 95.5 (91.3, 99.8) | −6.5 (−10.8, −2.3) | 0.76 |
TCV (L) | 4.3 (3.9, 4.8) | 4.4 (3.9, 5.0) | +0.2 (−4.7, 3.1) | 0.98 |
The prosthesis (n=24) | ||||
FVC (%) | 98.3 (92.0, 104.0) | 90.7 (84.0, 95.0) | −7.6 (−13.0, −4.0) | 0.14 |
FEV1 (%) | 98.2 (95.0, 110.0) | 91.2 (85.0, 101.0) | −7.0 (−12.0, −3.0) | 0.30 |
FEV1/FVC (%) | 73.1 (71.0, 79.0) | 71.6 (65.0, 79.0) | −1.5 (−5.0, 1.0) | 0.75 |
DLCO (%) | 79.3 (74.5, 82.5) | 75.0 (68.0, 80.5) | −4.3 (−6.5, −2.0) | 0.65 |
TCV (L) | 4.3 (3.7, 5.2) | 4.1 (3.5, 4.7) | −6.0 (−12.0, 3.7) | 0.57 |
Data are presented as median (interquartile range). TCV, thoracic cavity volume; FVC, forced vital capacity; FEV1, forced expiratory volume in 1 second; DLCO, diffusing capacity of the lung for carbon monoxide.
Discussion
This study demonstrated the long-term effects of CWR on pulmonary function and TCV. FEV1 and FVC decreased after CWR, whereas TCV was preserved. The pulmonary function decline was greater in patients with an extended CWR. The long-term protective effects of chest wall reconstruction on PFT and TCV are unclear.
CWR can result in any degree of change in pulmonary function because the chest wall plays an important role in the mechanics of respiration. However, the long-term effects of CWR on pulmonary function remain unclear because few studies have investigated this subject. Heuker et al. reported that 13.1% of FEV1 and 12.2% of FVC decreased after combined CWR and wedge resection. Pulmonary function was measured more than 1 year postoperatively; however, the extent of CWR was not clearly defined in this study (15). Meanwhile, Leuzzi et al. reported that about 4.8% of FEV1 and 12.1% of FVC decreased after CWR, albeit not significantly (16). The limitations of these studies included population heterogeneity and the adopted surgical methods. Therefore, in the present study, we limited the study population to patients who underwent rib cage and wedge resections to determine the exclusive effects of CWR. In this study, which included only four patients with single-wedge resection, FEV1 and FVC decreased by 7.9% and 7.0%, respectively. The effect of CWR on pulmonary function did not exceed the reported effect of pulmonary segmentectomy on pulmonary function (15,17). These findings may serve as a reference for risk assessment of CWR in patients with impaired pulmonary function.
An important finding of this study was that the decreases in morphological parameters and TCV were not statistically significant, whereas the decreases in functional parameters (FEV1 and FVC) were, and there was no correlation between the changes in TCV and FEV1 or FVC. This suggests that the effect of the CWR on pulmonary function is not limited to morphological changes but includes the dynamics of respiratory movement and chest wall compliance. However, increased rigidity at the surgical site, the compensatory mechanisms in respiratory movement, and patient rehabilitation could be factors contributing to the discrepancy between changes in TCV and pulmonary function. As expected, DLCO did not show any significant changes after CWR in this study. This might be because this study only included patients with minimal pulmonary resection, and gas exchange was influenced by the lung itself rather than the respiratory mechanism. Leuzzi et al. also reported no differences in DLCO and blood oxygen levels after CWR (16).
Separate quantitative analyses of each TCV revealed that different remodeling processes occurred in the ipsilateral and contralateral thoracic cavities after CWR; this is another important finding of this study. The ipsilateral TCV decreased, whereas the contralateral TCV showed an increasing trend, regardless of the operation side. The decrease in ipsilateral TCV was more severe in the right-side operation group but decreased FVC and FEV1 were more severe in the left-side operation group. These results may be related to the morphological asymmetry of the thoracic cavity. Jeong et al.’s study with adolescents treated with the Nuss procedure reported that the reduction of peak expiratory flow and inspiratory cavity was more prominent in patients with the asymmetric type (18). According to the Blickman et al.’s study, addressing asymmetry in patients with pectus excavatum can improve the pulmonary ventilation-perfusion ratio (19). Considering the natural asymmetry of the human thoracic cavity due to heart, the findings of that study may explain the findings of the present study. As asymmetry increases following left-side CWR, the decrease in pulmonary function is magnified. Conversely, right-side CWR tended to reduce asymmetry, which could have a protective effect on the decrease in pulmonary function.
A more rigid reconstruction was recommended after anterior CWR, whereas reconstruction was not recommended after upper and posterior CWR (8,20-22). Based on this, we analyzed the effect of the level and part of the resected chest wall. Changes in TCV were not significantly different according to the CWR level or part. FEV1 and FVC decreased significantly in mid-and lateral CWR. Insignificant changes in the PFT parameters in the upper and lower levels and in the anterior and posterior parts might be due to the compensatory effects of the sternum, scapula, diaphragm, and abdominal wall (8,20-22). More prominent decrease in FEV1 and FVC after the lateral/mid-level CWR could be due to the absence of overlaying rigid structures such as sternum and scapular. Leuzzi et al. reported that in univariable analysis, a statistically significant decline in FEV1 was observed on the anterolateral side (16). An animal experiment showed significant decreases in FEV1 and FVC after mid-and lateral CWR (23). Cappello et al. suggested that the combined effects of the absence of a bony thorax and loss of accessory muscles—including intercostal muscles—might be responsible (23).
In general, chest wall reconstruction has been recommended for resection of ≥3 ribs or defects size ≥5 cm to prevent acute respiratory distress. Several studies have shown that reconstruction can provide chest wall stability and prevent paradoxical movement during respiration in the acute phase (16,24). However, the long-term effect of chest wall reconstruction after CWR is not fully understood (8,9,25). In the present study, consisting of 24 (80%) ePTFE reconstructions among 30 reconstructions, the FEV1 of the no-reconstruction subgroup decreased significantly, whereas that of the reconstruction subgroup showed an insignificant decrease. However, no differences between the reconstruction and no-reconstruction subgroups were noted in the changes in FVC, FEV1/FVC ratio, and TCV. Considering that the size of the resected lesion was larger in the reconstruction than in the no-reconstruction subgroup, reconstruction is presumed to exert a protective effect not only on short- but also long-term pulmonary function. However, previous studies have reported contradictory results. Leuzzi et al. reported that the mean reduction in FEV1 was 4.1% in a prosthesis reconstruction subgroup and 17.5% in a no-prosthesis subgroup after extended CWR (16). On the contrary, Spicer et al. reported that reconstruction did not significantly affect postoperative pulmonary morbidity and FEV1 after CWR (25). The variation in outcomes across studies is believed to be due to differences in the patient cohorts themselves, whether the sternum was resected, and the extent of pulmonary resection. The variation in outcomes across studies is believed to be due to differences in the patient cohorts themselves, whether the sternum was resected, and the extent of pulmonary resection. In future studies, the effect of chest wall reconstruction should be verified considering defect size and location, and the material used for reconstruction.
The present study has several limitations. First, it is a retrospective design conducted at a single center and not all patients have PFT parameters. Second, the sample size was too small to perform an effective subgroup analysis. Third, preoperative PFT might have been underestimated because of preoperative pain and mass effects. Fourth, selection bias could not be avoided because the decision for reconstruction was made based on subjective judgement there was a tendency to choose reconstruction when the defect was larger. Additionally, in this study, a comparison based on the length of the resected rib was not conducted, because, in some cases, both the length of the rib itself or involve length of the resected muscle were measured in the pathological records. To overcome these limitations, prospective organized data collection is indispensable. The first step would be the development of a classification system for CWR regarding the extent and location of defects.
Conclusions
The long-term decline in pulmonary function after CWR was evident, especially in patients with resection of ≥3 ribs and left-side CWR. Reconstruction should be considered in these patients to exert a protective influence on both short-term and long-term pulmonary function. Alterations in the respiratory mechanism may be more influential than the remodeling of the thoracic cavity on long-term changes in pulmonary function.
Acknowledgments
This paper is based on an abstract presented at the 103rd annual meeting of AATS in 2023.
Funding: None.
Footnote
Reporting Checklist: The authors have completed the STROBE reporting checklist. Available at https://jtd.amegroups.com/article/view/10.21037/jtd-24-25/rc
Data Sharing Statement: Available at https://jtd.amegroups.com/article/view/10.21037/jtd-24-25/dss
Peer Review File: Available at https://jtd.amegroups.com/article/view/10.21037/jtd-24-25/prf
Conflicts of Interest: All authors have completed the ICMJE uniform disclosure form (available at https://jtd.amegroups.com/article/view/10.21037/jtd-24-25/coif). C.H.K. serves as an unpaid editorial board member of Journal of Thoracic Disease from October 2022 to September 2024. The other authors have no conflicts of interest to declare.
Ethical Statement: The authors are accountable for all aspects of the work in ensuring that questions related to the accuracy or integrity of any part of the work are appropriately investigated and resolved. The study was conducted in accordance with the Declaration of Helsinki (as revised in 2013). The study was approved by the Institutional Review Board of Seoul National University Hospital (approval No. 2204-145-1319) and individual consent for this retrospective analysis was waived.
Open Access Statement: This is an Open Access article distributed in accordance with the Creative Commons Attribution-NonCommercial-NoDerivs 4.0 International License (CC BY-NC-ND 4.0), which permits the non-commercial replication and distribution of the article with the strict proviso that no changes or edits are made and the original work is properly cited (including links to both the formal publication through the relevant DOI and the license). See: https://creativecommons.org/licenses/by-nc-nd/4.0/.
References
- Petrella F, Spaggiari L. Surgery of the chest wall: indications, timing and technical aspects. J Thorac Dis 2020;12:1-2. [Crossref] [PubMed]
- Scarnecchia E, Liparulo V, Capozzi R, et al. Chest wall resection and reconstruction for tumors: analysis of oncological and functional outcome. J Thorac Dis 2018;10:S1855-63. [Crossref] [PubMed]
- Wang L, Yan X, Zhao J, et al. Expert consensus on resection of chest wall tumors and chest wall reconstruction. Transl Lung Cancer Res 2021;10:4057-83. [Crossref] [PubMed]
- Bergeron EJ, Meguid RA, Mitchell JD. Chronic Infections of the Chest Wall. Thorac Surg Clin 2017;27:87-97. [Crossref] [PubMed]
- Weyant MJ, Bains MS, Venkatraman E, et al. Results of chest wall resection and reconstruction with and without rigid prosthesis. Ann Thorac Surg 2006;81:279-85. [Crossref] [PubMed]
- Dorado JH, Accoce M, Plotnikow G. Chest wall effect on the monitoring of respiratory mechanics in acute respiratory distress syndrome. Rev Bras Ter Intensiva 2018;30:208-18. [Crossref] [PubMed]
- Goldsmith I. Chest Wall Reconstruction With 3D Printing: Anatomical and Functional Considerations. Innovations (Phila) 2022;17:191-200. [Crossref] [PubMed]
- Althubaiti G, Butler CE. Abdominal wall and chest wall reconstruction. Plast Reconstr Surg 2014;133:688e-701e. [Crossref] [PubMed]
- Mansour KA, Thourani VH, Losken A, et al. Chest wall resections and reconstruction: a 25-year experience. Ann Thorac Surg 2002;73:1720-5; discussion 1725-6. [Crossref] [PubMed]
- Clermidy H, Fadel G, De Lemos A, et al. Long-term outcomes after chest wall resection and repair with titanium bars and sternal plates. Front Surg 2022;9:950177. [Crossref] [PubMed]
- Aghajanzadeh M, Alavy A, Taskindost M, et al. Results of chest wall resection and reconstruction in 162 patients with benign and malignant chest wall disease. J Thorac Dis 2010;2:81-5. [PubMed]
- Yoon SH, Jung JC, Park IK, et al. Clinical Outcomes of Surgical Treatment for Primary Chest Wall Soft Tissue Sarcoma. Korean J Thorac Cardiovasc Surg 2019;52:148-54. [Crossref] [PubMed]
- Graham BL, Steenbruggen I, Miller MR, et al. Standardization of Spirometry 2019 Update. An Official American Thoracic Society and European Respiratory Society Technical Statement. Am J Respir Crit Care Med 2019;200:e70-88. [Crossref] [PubMed]
- Tokuno J, Chen-Yoshikawa TF, Nakao M, et al. Resection Process Map: A novel dynamic simulation system for pulmonary resection. J Thorac Cardiovasc Surg 2020;159:1130-8. [Crossref] [PubMed]
- Heuker D, Lengele B, Delecluse V, et al. Subjective and objective assessment of quality of life after chest wall resection. Eur J Cardiothorac Surg 2011;39:102-8. [Crossref] [PubMed]
- Leuzzi G, Nachira D, Cesario A, et al. Chest wall tumors and prosthetic reconstruction: A comparative analysis on functional outcome. Thorac Cancer 2015;6:247-54. [Crossref] [PubMed]
- Suzuki K, Watanabe S, Wakabayashi M, et al. A nonrandomized confirmatory phase III study of sublobar surgical resection for peripheral ground glass opacity dominant lung cancer defined with thoracic thin-section computed tomography (JCOG0804/WJOG4507L). J Clin Oncol 2017;35:8561. [Crossref]
- Jeong JY, Ahn JH, Kim SY, et al. Pulmonary function before and after the Nuss procedure in adolescents with pectus excavatum: correlation with morphological subtypes. J Cardiothorac Surg 2015;10:37. [Crossref] [PubMed]
- Blickman JG, Rosen PR, Welch KJ, et al. Pectus excavatum in children: pulmonary scintigraphy before and after corrective surgery. Radiology 1985;156:781-2. [Crossref] [PubMed]
- Netscher DT, Baumholtz MA. Chest reconstruction: I. Anterior and anterolateral chest wall and wounds affecting respiratory function. Plast Reconstr Surg 2009;124:240e-52e. [Crossref] [PubMed]
- Netscher DT, Baumholtz MA, Bullocks J. Chest reconstruction: II. Regional reconstruction of chest wall wounds that do not affect respiratory function (axilla, posterolateral chest, and posterior trunk). Plast Reconstr Surg 2009;124:427e-35e. [Crossref] [PubMed]
- Rocco G. Overview on current and future materials for chest wall reconstruction. Thorac Surg Clin 2010;20:559-62. [Crossref] [PubMed]
- Cappello M, De Troyer A. On the respiratory function of the ribs. J Appl Physiol (1985) 2002;92:1642-6. [Crossref] [PubMed]
- Akiba T, Marushima H, Nogi H, et al. Chest wall reconstruction using Gore-Tex® dual mesh. Ann Thorac Cardiovasc Surg 2012;18:166-9. [Crossref] [PubMed]
- Spicer JD, Shewale JB, Antonoff MB, et al. The Influence of Reconstructive Technique on Perioperative Pulmonary and Infectious Outcomes Following Chest Wall Resection. Ann Thorac Surg 2016;102:1653-9. [Crossref] [PubMed]