Comparison of high-flow nasal cannula with conventional oxygen therapy for preventing postoperative hypoxemia in patients with lung resection surgery: a systematic review and meta-analysis
Highlight box
Key findings
• Following extubation after lung resection, the use of prophylactic high-flow nasal cannula oxygen therapy may improve the oxygenation index compared to conventional oxygen therapy.
What is known and what is new?
• High-flow nasal cannula compared to conventional oxygen therapy may significantly decrease the incidence of post-extubation respiratory failure, improve PaO2 levels, and can be safely administered to patients following planned extubation.
• Following extubation after lung resection, the use of prophylactic high-flow nasal cannula oxygen therapy did not yield a statistically significant difference in preventing hypoxemia when compared with conventional oxygen therapy.
What is the implication, and what should change now?
• In clinical practice, using high-flow nasal cannula oxygen therapy prophylactically for patients following lung resection does not decrease the incidence of post-extubation hypoxemia compared to conventional oxygen therapy. Nevertheless, high-flow nasal cannula oxygen therapy presents benefits in enhancing the oxygenation index.
Introduction
Patients undergoing lung resection are exposed to potential postoperative morbidity and mortality (1). Following thoracic surgery, acute respiratory failure (ARF) is common, caused by decreased lung capacity, diminished airway clearance, diaphragmatic dysfunction, atelectasis due to postoperative alveolar collapse or fluid accumulation, and pain-induced breathing restriction (2-4). Supplemental oxygen is frequently necessary to enhance arterial oxygenation in the postoperative period. Although it is successful in treating the majority of cases of hypoxemia, patients with a low ventilation-perfusion ratio may only be partially responsive to an augmented oxygen concentration (5).
Utilizing noninvasive ventilation (NIV) to prevent/treat respiratory failure after lung resection has been proposed (6-9), yet its regular practice in clinical settings requires personnel expertise and technological resources that may not be available in surgical wards. In the initial postoperative period, the introduction of positive airway pressure and the chance of patient-ventilator asynchronies during assisted ventilation may endanger the integrity of bronchial anastomosis as a result of sudden fluctuations in airway transmural pressure.
Conventional oxygen therapy (COT) is the primary form of supportive care given to patients extubated after lung resection procedure, and is typically administered through nasal prongs, cannulae, or masks. Nevertheless, the maximal oxygen flow rate that these devices can provide is restricted. Besides, the oxygen supplied is diluted by the air surrounding us, thus resulting in a considerable drop in the fraction of inspired oxygen (FiO2) in the alveoli (10).
High-flow nasal cannula (HFNC) offers airway pressure that is determined by the flow rate, and compared to NIV, it can be more comfortable and efficient in terms of oxygen delivery. HFNC is a device that provides oxygen to the patient through a nasal cannula with a maximum flow rate of 60 L/min, allowing for a FiO2 of up to 100% (11). Research has revealed that HFNC is more effective than COT (e.g., traditional nasal cannula, simple face mask, Venturi mask) in terms of sustaining end-expiratory lung volume, providing comfort and tolerance, decreasing respiratory work, and decreasing the rate of intubation in cases of acute hypoxic respiratory failure (12-14).
However, the data examining routine HFNC use in patients with lung resection surgery is less clear. Therefore, we aimed to perform a rigorous, comprehensive and repeatability systematic meta-analysis to robustly quantify the benefits of HFNC for postoperative weaning patients with lung resection surgery by investigating the ratio of postoperative hypoxemia and other patient-centered outcomes. We hypothesized that routine HFNC use would reduce the risk of postoperative hypoxemia in comparison to COT, and may increase the oxygen index. We present this article in accordance with the PRISMA reporting checklist (available at https://jtd.amegroups.com/article/view/10.21037/jtd-23-1758/rc) (15).
Methods
In advance, the protocol was registered with PROSPERO (https://www.crd.york.ac.uk/PROSPERO; CRD42023437259). No ethics approval was necessary for this systematic review as it did not involve patient-level data.
Data sources and search strategy
We conducted a systematic search of PubMed, Embase, the Cochrane Library, Web of Science and Scopus from inception to July 2023 to identify randomized controlled trial (RCT) that compared the effectiveness of HFNC with COT in patients extubated after lung resection surgery. No language restrictions were applied. The search terms used were: “Humidification oxygen” OR “humidified oxygen” OR “HFO” OR “high-flow” OR “high flow” OR “hfnc” OR “hfnp” OR “hfno” OR “Nasal Cannula” OR “optiflow” combined with “lung resection” OR “pneumonectomy” OR “lobectomy” OR “wedge resection” OR “video assisted thoracoscopic surgery” OR “vats”. We conducted an exhaustive review of the references of pertinent articles to ensure that no studies were omitted. For a thorough search tactics, consult the attachment section (Appendix 1: Tables S1-S5).
Inclusion and exclusion criteria
Our meta-analysis included studies that met the following criteria: (I) population: adults and adolescents (≥10 years of age) patients extubated after lung resection surgery; (II) intervention: receiving HFNC therapy after postoperative extubation; (III) control: receiving COT therapy after postoperative extubation; and (IV) outcomes: the primary outcome of our study was the incidence of postoperative hypoxemia [i.e., the ratio of the partial pressure of arterial oxygen to FiO2 (PaO2/FiO2) is below 300 mmHg], while the secondary outcomes included change in PaO2/FiO2 after extubation, reintubation rate, escalation of respiratory support, change in partial pressure of carbon dioxide (PaCO2) after extubation, hospital and intensive care unit (ICU) length of stay.
The research was limited to RCT, excluding case series, case reports, editorials, observational studies, and full texts not available.
Definitions
Postoperative hypoxemia was identified by PaO2/FiO2 that was lower than 40 kPa (300 mmHg, 1 mmHg =0.133 kPa) (5,16). HFNC oxygen therapy was defined as a respiratory support that delivers a high flow (more than 15 L/min) of heated and humidified oxygen administered through a nasal cannula. COT was referred to relatively low-flow oxygen (≤15 L/min) through a nasal cannula, a simple face mask, a Venturi mask, or a high-concentration reservoir mask. Escalation of respiratory support was defined as an increase in oxygen support therapy, either to HFNC (if in the COT group), NIV, or invasive mechanical ventilation.
Study selection and quality assessment
Two independent investigators conducted a two-step screening process of all records: first, titles and abstracts were reviewed, and then full-text reviews were conducted. A modified Jadad score was used to evaluate the quality of the RCT included, with scores of 1 to 3 considered low quality and scores of 4 to 7 considered high quality. The risk of bias (RoB) for RCT was independently and in duplicate assessed by using the revised Cochrane RoB 2 tool (17), which classifies RoB across five bias domains: bias arising from the randomization process, bias due to deviations from intended interventions, bias due to missing outcome data, bias in measurement of the outcome, and bias in selection of the reported result, as “no information”, “no”, “probably no”, “probably yes”, or “yes”. Discussions were held to settle any disagreements about the quality of the RCT and RoB assessments.
Data extraction
Two investigators independently gathered data, and any discrepancies were settled by a third investigator, if necessary. To extract detailed study information, Navicat 16 for PostgreSQL, convenient graphical tool for PostgreSQL database development (https://www.navicat.com/en/products), was employed with a consistent data extraction form. This form comprised the author, year, study population, country, methods of oxygen delivery, study design, number of subjects, oxygen index, postoperative hypoxemia, escalation of respiratory support, ICU and hospital length of say, baseline characteristics, and patient-centered outcomes. In the attached file, we provided a unified format for data organization (Appendix 1: Figure S1). The categorical variables were determined by the number of patients who had the event and the total number of patients in each group, while continuous variables were obtained in terms of sample size, mean and standard deviation (SD), or median and interquartile range (IQR), as stated in the studies. The mean and SD were calculated when the results were given as IQR (18,19). If the relevant data were not presented in a table but in the form of a graph, we would use the DigitizeIt software (Braunschweig, Germany, https://www.digitizeit.xyz/) for precise data extraction. Obtaining the necessary data is detailed in the Appendix 1 (Table S6, Figures S2,S3 extracting the PaO2/FiO2; Table S7, Figures S4,S5 extracting the PaCO2). When multiple sets of results were reported, we chose to use the intention-to-treat dataset.
Statistical analysis
Our meta-analysis, conducted with an intention-to-treat approach, encompassed all participants who were assigned to any of the study groups. Data were obtained either through direct extraction or by calculating indirectly. The Mantel-Haenszel technique was employed to ascertain study weights for dichotomous results and inverse variances for continuous outcomes. Pooled relative risk (RR) was expressed as dichotomous outcomes, and mean difference (MD) was represented as continuous outcomes, both with 95% confidence interval (CI).
The statistical heterogeneity was determined by means of the Chi2 and I2 statistics. A Chi2 P value of less than 0.1 or an I2 greater than 50% was established as the criterion for significant heterogeneity (20). Sensitivity analyses were conducted to explore the significant heterogeneity between studies and to determine if any baseline factors had an effect on the treatment effects. When no significant statistical heterogeneity was observed, the outcomes of the fixed-effect model were reported; if this was not the case, the outcomes of the random-effects model were presented.
The meta-analysis was conducted by using the RevMan 5.4 software from the Cochrane Collaboration and TSA software (version 0.9.5.9 Beta, Copenhagen Trial Unit, Copenhagen, Denmark) for data analysis.
Sensitivity analysis
We performed a pre-specified sensitivity analysis, limiting the studies to those with no high RoB. A sensitivity analysis was carried out, omitting trials with a high RoB. Sensitivity analyses were conducted on the primary outcomes to test the robustness of the results by the following method: excluding studies with a high RoB.
Analyzing the certainty of evidence
The Grading of Recommendations, Assessment, Development and Evaluation (GRADE) approach was used to evaluate the level of certainty of evidence for all outcomes (21). GRADE assesses the RoB, inconsistency, indirectness, imprecision, and publication bias for each individual study. This outcome was assessed by two reviewers, working independently and in pairs. Evidence certainty was classified as very low, low, moderate, or high. GRADEpro software (https://www.gradepro.org/) was employed to formulate the Summary of Findings (SoF) table. All decisions were justified in the footnotes.
Trial sequential analysis (TSA)
To assess whether the required information size (RIS) to achieve statistical significance was met for the significant outcome of postoperative hypoxemia, we employed TSA (22) (version 0.9.5.10 Beta; Copenhagen Trial Unit, Copenhagen, Denmark; available at http://ctu.dk/tsa/). A random-effects model was utilized to conduct a TSA for the primary outcome of postoperative hypoxemia. The TSA utilized a statistical significance level of type 1 error, power, and RR reduction. By utilizing cumulative Z-scores and the RIS, we can determine the magnitude of the effect with certainty.
Results
Study identification and selection
Our study identified 760 relevant publications. After removing duplicate results, 724 publications were rescreened for titles and abstracts. Twenty-five studies were obtained for full-context review, and 20 studies were excluded. The details of the excluded studies and the reasons for exclusion were incorrect comparators, wrong populations, and incorrect article styles. Finally, we included five studies (5,23-26) with a total of 564 patients (median, 120 patients; range, 59–180 patients; HFNC group, 281 patients; COT group, 283 patients) in this meta-analysis. The selection process of the eligible studies was shown in Figure 1. The included study characteristics and baseline patient characteristics are shown in Table 1. All studies were restricted to those who had gone through lung resection surgery and they were parallel-group RCTs.
Table 1
Study | Country | Surgery type | Age (years), mean ± SD | No. of patients female/group |
BMI (kg/m2), mean ± SD |
HFNC parameters | COT parameters | Oxygen duration | PaO2/FiO2 (mmHg), mean ± SD |
Outcomes | ||||||||||||
---|---|---|---|---|---|---|---|---|---|---|---|---|---|---|---|---|---|---|---|---|---|---|
HFNC | COT | HFNC | COT | HFNC | COT | Initial flow (L/min) | FiO2 | Target SpO2 | Delivery method | Oxygen flow (L/min) | Target SpO2 | HFNC | COT | |||||||||
El-Nori 2023, (26) | Egypt | Lung resection | 45.4±15.5 | 48.0±14.7 | 28/90 | 35/90 | Null | Null | 35–60 | FiO2 was titrated | ≥95% | Nasal prongs or facemask | Oxygen flow was titrated | ≥92% | First 48 h after extubation | Null* | Null* | ①③⑥⑦ | ||||
Zhu 2022, (25) | China | Lobectomy resection | 69.0±5.2** | 66.5±4.8** | 32/60 | 35/60 | Null | Null | 40–50 | 50% | ≥95% | Nasal prongs | Null | ≥92% | First 12 h after extubation | Null* | Null* | ②⑤⑥ | ||||
Pennisi 2019, (5) | Italy | Lung resection | 66.0±10.0** | 68.0±9.0** | 20/47 | 21/48 | 26.0±4.0 | 27.0±4.0 | 50 | FiO2 was titrated | 92–98% | Venturi mask | Oxygen flow was titrated | 92–98% | First 48 h after extubation | Null* | Null* | ①②④⑤⑥ | ||||
Yu 2017, (24) | China | Lobectomy resection | 56.3±7.0 | 55.8±7.9 | 26/56 | 26/54 | 26.3±4.7 | 25.2±5.0 | 35–60 | FiO2 was titrated | ≥95% | Nasal prongs or facemask | Oxygen flow was titrated | ≥95% | First 72 h after extubation | 350.3±33.8 | 340.9±40.6 | ①②③④⑦ | ||||
Ansari 2016, (23) | United Kingdom | Lung resection | 68.3±8.5** | 65.7±13.0** | 14/28 | 17/31 | 26.8±5.0 | 27.6±5.5 | 20–50 | FiO2 was titrated | ≥93% | Facemask or nasal prongs | 2 to 4 L/min | ≥93% | First 24 h after extubation | Null* | Null* | ⑥ |
Outcome indicator: ① the incidence of postoperative hypoxemia; ② the differences of PaO2/FiO2 after extubation; ③ reintubation rate; ④ escalation of respiratory support; ⑤ the differences of PaCO2 after extubation; ⑥ hospital length of stay; ⑦ ICU length of stay. *, article baseline data is not recorded, but the article expresses baseline balance between two groups; **, high-risk factor with mean age ≥65 years. SD, standard deviation; BMI, body mass index; HFNC, high-flow nasal cannula; COT, conventional oxygen therapy; PaO2/FiO2, the partial pressure of arterial oxygen to the fraction of inspired oxygen; FiO2, fraction of inspired oxygen; SpO2, peripheral capillary oxygen saturation; Null, nothing was stated in the original article; PaCO2, partial pressure of carbon dioxide; ICU, intensive care unit.
Certainty of evidence grade
For the number of outcome estimates for pooled effect sizes, Cochrane recommends choosing up to seven main outcomes, as these are essential for decision-making, with emphasis on patient-important outcomes (Cochrane Handbook 2012, 5.4a). Therefore, we presented seven primary results in Table 2 and the other secondary results in Appendix 1 (Table S8). The ultimate conclusion was that the evidence for postoperative hypothermia is very low on the hierarchy of evidence. Moderate-level evidence was reflected in reintubation rates, escalation of respiratory support, and ICU length of stay. The indicators of low certainty included 1-hour PaO2/FiO2 ratio following extubation, 1-hour PaCO2 following extubation, and the hospital length of stay.
Table 2
Primary results | Certainty assessment | No. of patients | Effect | Certainty | ||||||||||
---|---|---|---|---|---|---|---|---|---|---|---|---|---|---|
No. of studies | Study design | Risk of bias | Inconsistency | Indirectness | Imprecision | Other considerations | HFNC | COT | RR (95% CI) | Absolute (95% CI) | ||||
Postoperative hypoxemia (assessed with: P/F ratio <300 mmHg) | 3 (5,24,26) | Randomised trials | Not seriousa | Seriousb,c | Not seriousd | Seriouse,f | None | 50/193 (25.9%) | 63/192 (32.8%) | 0.67 (0.30 to 1.49) | 108 fewer per 1,000 (from 230 fewer to 161 more) | ⨁⨁⊝⊝; low | ||
1-hour PaO2/FiO2 ratio after extubation | 3 (5,24,25) | Randomised trials | Not seriousa | Not seriousg | Not seriousd | Seriouse,f | None | 163 | 162 | Null | MD 19.77 higher (6.09 higher to 33.46 higher) | ⨁⨁⨁⊝; moderate | ||
Reintubation rate | 2 (24,26) | Randomised trials | Not seriousa | Not seriousg,h | Not seriousd | Not seriouse,i | None | 1/146 (0.7%) | 7/144 (4.9%) | 0.25 (0.04 to 1.54) | 36 fewer per 1,000 (from 47 fewer to 26 more) | ⨁⨁⨁⨁; high | ||
Escalation of respiratory support | 2 (5,24) | Randomised trials | Not seriousa | Not seriousg,h | Not seriousd | Not seriouse,i | None | 4/103 (3.9%) | 12/102 (11.8%) | 0.35 (0.11 to 1.08) | 76 fewer per 1,000 (from 105 fewer to 9 more) | ⨁⨁⨁⨁; high | ||
1-hour PaCO2 after extubation | 3 (5,24,25) | Randomised trials | Not seriousa | Seriousb | Not seriousd | Not seriouse,i | None | 163 | 162 | Null | MD 1.22 lower (2.94 lower to 0.49 higher) | ⨁⨁⨁⊝; moderate | ||
Hospital length of stay (assessed with: days) | 4 (5,23,24,26) | Randomised trials | Not seriousa | Not seriousg,h | Not seriousd | Seriouse,f | None | 221 | 223 | Null | MD 0.19 lower (0.44 lower to 0.06 higher) | ⨁⨁⨁⊝; moderate | ||
ICU length of stay (assessed with: days) | 2 (24,26) | Randomised trials | Not seriousa | Not seriousg,h | Not seriousd | Not seriouse,i | None | 146 | 144 | Null | MD 0.02 higher (0.16 lower to 0.19 higher) | ⨁⨁⨁⨁; high |
Explanations: a, the overall risk of bias of the included articles is some concerns by using the modified Cochrane RoB 2; b, point estimates that are significantly different and I-squared values greater than 50%; c, after sensitive analysis of removing age risk factor (age ≥65 years), it has good homogeneity; d, there were direct outcomes in terms of population, intervention, outcome assessment, and intervention modalities, with no indirect outcomes; e, imprecision varied slightly between the different evidence assessors, and we judged imprecision by assessing the width and narrowness of the 95% CI between studies; f, the width of the 95% CI varied widely between studies; g, the between-study confidence intervals had good overlap; h, good homogeneity and I-squared =0%; i, the width of 95% CI was consistent between studies. Quality of the evidence (GRADE): ⨁⊝⊝⊝: very low quality of the evidence; ⨁⨁⊝⊝: low quality of the evidence; ⨁⨁⨁⊝: moderate quality of the evidence; ⨁⨁⨁⨁: high quality of the evidence. HFNC, high-flow nasal cannula; COT, conventional oxygen therapy; CI, confidence interval; P/F, PaCO2/FiO2; RR, relative risk; Null, representation of effect magnitude for continuous data is not feasible using the RR; MD, mean difference; ICU, intensive care unit; PaCO2, partial pressure of carbon dioxide; FiO2, fraction of inspired oxygen; RoB 2, risk of bias evaluation tool 2.0; GRADE, Grading of Recommendations, Assessment, Development and Evaluation.
Quality and RoB in studies
We used the modified Jadad score to evaluate the included studies in order to determine the quality of them. Four articles covering the concealment of allocation were 1 point (5,24-26), and one study was two points (23). No blinding to be used for all research interventions of oxygen style. The result of this meta-analysis reveals that four articles (5,24-26) were scored 4, and one article (23) was scored 5 (Appendix 1: Table S9).
The results of all studies showed there were some concerns found by the RoB 2 (the modified Cochrane RoB evaluation tool 2) (Figure 2, Table S10). Within Domain 2, one article is an open-label study (5), another is an unblinded study (24), two articles mention random allocation without specifying blinding (25,26), and one article notes blinding for anesthesiologists and surgeons (23). None of the five articles mentioned implementing blinding for the intervener or the patient (5,23-26), potentially leading to the intervener or participant being aware of the intervention allocation plan. Hence, the assessment outcomes in this domain are some concerns. In Domain 4, the absence of any mention of blinding implementation for outcome evaluators in all articles creates uncertainty about their awareness of the allocation of intervention measures (5,23-26). Consequently, Domain 4 of RoB 2 raises some concerns. In Domain 5 section, three articles revealed certain findings, because they did not mention a pre-specified analysis plan. The supplementary materials section contains detailed evaluation content for RoB 2 (Appendix 1: Tables S11-S15).
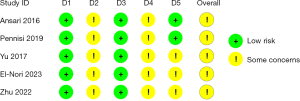
Outcomes
Postoperative hypoxemia
The data on postoperative hypoxemia were available from three studies (5,24,26). There was no statistically significant difference in the incidence of postoperative hypoxemia in three RCTs involving 385 patients (RR, 0.67; 95% CI: 0.30–1.49; low certainty; Figure 3). There was significant heterogeneity among the studies (Chi2=8.58, df=2, P=0.01, I2=77%) which might be due to a heterogeneous population of patients among the included studies (Table 1). Omitting the high-risk factor (mean age ≥65 years) from the analysis, sensitivity analysis revealed that HFNC could reduce the risk of postoperative hypoxemia (RR, 0.47; 95% CI: 0.25–0.89; P=0.02; very low certainty) with no heterogeneity (Chi2=0.17, P=0.68, I2=0%) (Appendix 1: Figure S6). The absolute risk difference was −10.8% (95% CI: −2.3% to 1.6%) (Table 2).
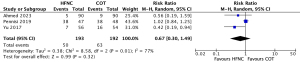
PaO2/FiO2
Results from three studies (n=325) were pooled to determine a significant difference in the change in PaO2/FiO2 ratio at 1 hour (MD, 19.77; 95% CI: 6.09–33.46; I2=35%; low certainty), 3 to 6 hours (MD, 23.87; 95% CI: 8.41–39.34; I2=29%; low certainty), 12 hours (MD, 28.41; 95% CI: 24.96–31.87; I2=0%; low certainty) between those treated with HFNC and COT (Figure 4), however, no significant difference was seen at 24 hours (MD, 19.03; 95% CI: −9.37 to 47.42; I2=73%; very low certainty) (Figure 4) (5,24,25).
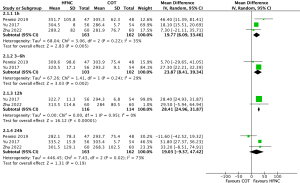
Reintubation rate
Two studies (n=290) reported on the reintubation rate (24,26). The reintubation rate between the two interventions did not differ statistically (RR, 0.25; 95% CI: 0.04–1.54; I2=0%; high certainty) (Appendix 1: Figure S7). The absolute risk difference was estimated at −3.6% (95% CI: −4.7% to 2.6%) (Table 2).
Escalation of respiratory support
Two studies (n=205) explored the concept of escalating oxygen therapy (5,24). The Escalation in Oxygen Therapy did not show any statistical variation between the two intervention groups (RR, 0.35; 95% CI: 0.11–1.08; I2=0%; high certainty) (Appendix 1: Figure S8). The absolute risk difference was −7.6% (95% CI: −10.5% to 9%) (Table 2).
Postoperative PaCO2
Three studies (n=325) were conducted to measure change in PaO2 after extubation at 1 hour (MD, −1.22; 95% CI: −2.94 to 0.49; I2=82%; low certainty), 3 to 6 hours (MD, −0.79; 95% CI: −5.29 to 3.72; I2=93%; very low certainty), 12 hours (MD, 0.07; 95% CI: −0.5 to 0.64; I2=0%; low certainty), and 24 hours (MD, −0.82; 95% CI: −2.81 to 1.17; I2=86%; very low certainty), with no reported distinction in PaO2 levels at any of the time points (Appendix 1: Figure S9) (5,24,25).
Hospital length of stay
Four studies (n=444) measured hospital length of stay (5,23,24,26). The length of hospital stay for those who received HFNC was not significantly different from those who received COT (MD, −0.19 days; 95% CI: –0.44 to 0.06; I2=0%; moderate certainty) (Appendix 1: Figure S10).
ICU length of stay
An analysis of two studies (n=290) revealed that the use of HFNC did not significantly reduce the duration of ICU length of stay in comparison to COT (MD, 0.02 days; 95% CI: −0.16 to 0.19; I2=0%; high certainty) (Appendix 1: Figure S11) (24,26).
Sensitivity analysis and TSA
Owing to the limited number of articles and the absence of subgroup grouping conditions, subgroup analysis could not be conducted. Sensitivity analysis excluding the high risk of age (mean age ≥65 years) in the incidence of postoperative hypoxemia altered the results of the analyzed outcomes (Appendix 1: Figure S6).
The TSA utilized a statistical significance level of 5%, a power of 80%, and an RR reduction of 21%. According to TSA, the amount of RIS for conclusive result was 1,372. In the accompanying document, we have outlined the procedure for calculating the RR reduction (Appendix 1: Figures S12,S13).
Discussion
This systematic review and meta-analysis of five RCTs (n=564 patients) conducted in patients undergoing lung resection revealed that preemptive use of HFNC after extubation, as compared to COT, did not lead to a reduced the risk of postoperative hypoxemia (very low certainty) nor had any effect on other pre-specified secondary outcomes such as reintubation rate (moderate certainty), escalation of respiratory support (moderate certainty), change in postoperative PaCO2 (low certainty), hospital length of stay (low certainty) and ICU length of stay (moderate certainty). However, HFNC did lead to a significant improvement in PaO2/FiO2 ratio within the first 12 hours in patients extubated after lung resection surgery (low certainty). Drawing from the conclusion above, we have supplied very comprehensive materials in the Appendix 1 for other researchers to repeat this meta-analysis.
According to European Respiratory Society (ERS) clinical practice guidelines (16), the implementation of non-invasive positive pressure ventilation (NIPPV) is recommended for those who have gone through pneumonectomy and experienced hypoxemia to avert atelectasis. Furthermore, the guidelines make a conditional suggestion to use HFNC instead of COT in hypoxemic ARF. The ERS guidelines do not address the prevention of hypoxemia in patients after lung resection surgery; however, they did recommend improving postoperative oxygenation to lower the risk of pulmonary issues such as atelectasis and pneumonia. Of note, there was limited evidence for the prevention of hypoxaemia after a pneumonectomy, and more evidence was needed to demonstrate that the benefits of HFNC are superior to those of COT.
Studies have demonstrated that HFNC is more beneficial than low-flow oxygen when used preemptively in critically ill patients (27,28), yet the evidence is not consistent when applied to the postoperative period. Our meta-analysis displays that preemptive high-flow nasal oxygen did not prove to be effective in reducing the incidence of postoperative hypoxemia when compared to COT in patients extubated after lung resection surgery. Yu et al.’s study (24) revealed that the rate of postoperative hypoxemia occurrence was twice as high in the COT compared to the HFNC, and the PaO2/FiO2 ratio was significantly improved within the initial 72 hours post-extubation. El-Nori et al.’s examination affirms the conclusions of the aforesaid study (26). When compared to the usage of a Venturi mask after thoracotomic lung resection, a study conducted by Pennisi et al. (5) discovered that the implementation of preemptive high-flow nasal oxygen did not lessen the occurrence of postoperative hypoxemia. The disparity in the conclusions on the prevalence of postoperative hypoxia between sensitivity analysis and the investigation of baseline data in the article may be attributed to the age of the population; the Pennisi et al.’s study revealed that the mean age (mean age ≥65 years) was a major risk factor. To confirm our primary outcome, more extensive and multi-site studies are required.
Utilizing HFNC rather than COT may be a viable option for increasing oxygenation in patients after extubation. Our results, which were determined through the PaO2/FiO2 ratio, were in line with those of Zhu and colleagues (10), who measured oxygenation after extubation through PaO2. There was no statistically significant difference between HFNC and COT in terms of reintubation rate, escalation of respiratory support, change in PaCO2, ICU, and hospital length of stay for patients extubated after lung resection surgery. Zhu and colleagues’ study (10) verified that, for individuals with planned extubation, HFNC was not as effective as COT in terms of reintubation rate, PaCO2, length of ICU stay, and hospital stay. Results from Chaudhuri et al.’s research (29) demonstrated that HFNC was more advantageous than COT in terms of reintubation rate and the escalation of respiratory support in the immediate postoperative period. The Chaudhuri et al.’s study (29) may have varied from other studies due to the inclusion of the scoping population and the utilization of different surgical methods.
According to the German S3 Guideline (30), nasal prongs are recommended as the first-line option for low oxygen flow rates (<6 L/min); alternatively, Venturi masks can be utilized with low oxygen flow rates. Venturi masks operate on the basis of Bernoulli’s principle, whereby oxygen is delivered through a tapered nozzle, creating a swirling air/oxygen mix at a high flow rate for inhalation. The Venturi mask, equipped with an FiO2 conversion indicator, may deliver a gas flow rate insufficient to meet the patients' inspiratory demands. In this scenario, the patient inhales additional ambient air, resulting in a lower oxygen concentration being delivered to the patient than the intended set concentration (31). The high-flow system maintains a constant FiO2 by delivering gas at flow rates exceeding the patient’s peak inspiratory flow rate. Hence, the oxygen concentration ultimately delivered to the patient equals the predetermined FiO2. The classification of Venturi masks within the spectrum of oxygen therapy methods is a subject of controversy. Uygur et al. identified Venturi mask as high-flow oxygen delivery systems in comparison to ordinary oxygen mask (32). In contrast, Maggiore et al.’s research demonstrates that the Venturi mask functions as a low-flow system when compared to HFNC (31). This study employed a grouping method consistent with several previous studies (10,33,34), classifying the Venturi mask as part of COT. Readers may find the classification of the Venturi mask as COT confusing, and we recommend approaching it with a dialectical mindset.
This review is commendable for its extensive literature search, strict adherence to the pre-registered protocol, exclusive focus on patients extubated after lung resection surgery, and utilization of GRADE methodology to assess the certainty of pooled estimates of effect. It is noteworthy that we have created a comprehensive two-tier catalogue of Appendix 1, providing readers with easy access to research materials that demonstrate scientific precision, accuracy, and repeatability. Our meta-analysis is restricted by a number of limitations. First, the limited number of studies included in the comparison between HFNC and COT for postoperative hypoxemia makes it difficult to draw any decisive conclusions. Second, despite the fact that the modified Jadad score of the five articles was 4–5 points, indicating a high-quality article, the evaluation result of the revised RoB 2 tool for RCT reveals that all of them presented an uncertain risk classification. The divergence in evaluation findings can be attributed to the enhanced and more stringent criteria of RoB 2, as delineated in Appendix 1 (Tables S11-S15). Third, this study lacked sufficient data to analyze the effects of pertinent subgroups. Finally, the TSA results indicated a RIS of 1,372, thereby necessitating further examination to establish the effectiveness of HFNC as opposed to COT in diminishing the risk of postoperative hypoxemia in patients extubated after lung resection surgery.
Conclusions
In summary, this meta-analysis indicated that the preemptive use of HFNC, as opposed to a COT, in patients extubated after lung resection surgery might not make a significant difference in the incidence of postoperative hypoxemia, reintubation rate, escalation of respiratory support, difference in postoperative PaCO2, hospital and ICU length of stay. However, HFNC may significantly enhance the oxygenation index within the first 12 hours post-extubation following lung resection surgery. Further studies are needed to confirm the findings of this meta-analysis.
Acknowledgments
Thanks to Dr. Ling Liu for the guidance on research methods.
Funding: None.
Footnote
Reporting Checklist: The authors have completed the PRISMA reporting checklist. Available at https://jtd.amegroups.com/article/view/10.21037/jtd-23-1758/rc
Peer Review File: Available at https://jtd.amegroups.com/article/view/10.21037/jtd-23-1758/prf
Conflicts of Interest: All authors have completed the ICMJE uniform disclosure form (available at https://jtd.amegroups.com/article/view/10.21037/jtd-23-1758/coif). The authors have no conflicts of interest to declare.
Ethical Statement: The authors are accountable for all aspects of the work in ensuring that questions related to the accuracy or integrity of any part of the work are appropriately investigated and resolved.
Open Access Statement: This is an Open Access article distributed in accordance with the Creative Commons Attribution-NonCommercial-NoDerivs 4.0 International License (CC BY-NC-ND 4.0), which permits the non-commercial replication and distribution of the article with the strict proviso that no changes or edits are made and the original work is properly cited (including links to both the formal publication through the relevant DOI and the license). See: https://creativecommons.org/licenses/by-nc-nd/4.0/.
References
- Memtsoudis SG, Besculides MC, Zellos L, et al. Trends in lung surgery: United States 1988 to 2002. Chest 2006;130:1462-70. [Crossref] [PubMed]
- Jaber S, Lescot T, Futier E, et al. Effect of Noninvasive Ventilation on Tracheal Reintubation Among Patients With Hypoxemic Respiratory Failure Following Abdominal Surgery: A Randomized Clinical Trial. JAMA 2016;315:1345-53. [Crossref] [PubMed]
- Fernandez-Bustamante A, Frendl G, Sprung J, et al. Postoperative Pulmonary Complications, Early Mortality, and Hospital Stay Following Noncardiothoracic Surgery: A Multicenter Study by the Perioperative Research Network Investigators. JAMA Surg 2017;152:157-66. [Crossref] [PubMed]
- Melamed R, Boland LL, Normington JP, et al. Postoperative respiratory failure necessitating transfer to the intensive care unit in orthopedic surgery patients: risk factors, costs, and outcomes. Perioper Med (Lond) 2016;5:19. [Crossref] [PubMed]
- Pennisi MA, Bello G, Congedo MT, et al. Early nasal high-flow versus Venturi mask oxygen therapy after lung resection: a randomized trial. Crit Care 2019;23:68. [Crossref] [PubMed]
- Jaber S, Antonelli M. Preventive or curative postoperative noninvasive ventilation after thoracic surgery: still a grey zone? Intensive Care Med 2014;40:280-3. [Crossref] [PubMed]
- Perrin C, Jullien V, Vénissac N, et al. Prophylactic use of noninvasive ventilation in patients undergoing lung resectional surgery. Respir Med 2007;101:1572-8. [Crossref] [PubMed]
- Lorut C, Lefebvre A, Planquette B, et al. Early postoperative prophylactic noninvasive ventilation after major lung resection in COPD patients: a randomized controlled trial. Intensive Care Med 2014;40:220-7. [Crossref] [PubMed]
- Lefebvre A, Lorut C, Alifano M, et al. Noninvasive ventilation for acute respiratory failure after lung resection: an observational study. Intensive Care Med 2009;35:663-70. [Crossref] [PubMed]
- Zhu Y, Yin H, Zhang R, et al. High-flow nasal cannula oxygen therapy versus conventional oxygen therapy in patients after planned extubation: a systematic review and meta-analysis. Crit Care 2019;23:180. [Crossref] [PubMed]
- Ovtcharenko N, Ho E, Alhazzani W, et al. High-flow nasal cannula versus non-invasive ventilation for acute hypercapnic respiratory failure in adults: a systematic review and meta-analysis of randomized trials. Crit Care 2022;26:348. [Crossref] [PubMed]
- Corley A, Caruana LR, Barnett AG, et al. Oxygen delivery through high-flow nasal cannulae increase end-expiratory lung volume and reduce respiratory rate in post-cardiac surgical patients. Br J Anaesth 2011;107:998-1004. [Crossref] [PubMed]
- Parke R, McGuinness S, Eccleston M. Nasal high-flow therapy delivers low level positive airway pressure. Br J Anaesth 2009;103:886-90. [Crossref] [PubMed]
- Schwabbauer N, Berg B, Blumenstock G, et al. Nasal high-flow oxygen therapy in patients with hypoxic respiratory failure: effect on functional and subjective respiratory parameters compared to conventional oxygen therapy and non-invasive ventilation (NIV). BMC Anesthesiol 2014;14:66. [Crossref] [PubMed]
- Page MJ, McKenzie JE, Bossuyt PM, et al. The PRISMA 2020 statement: an updated guideline for reporting systematic reviews. BMJ 2021;372: [PubMed]
- Oczkowski S, Ergan B, Bos L, et al. ERS clinical practice guidelines: high-flow nasal cannula in acute respiratory failure. Eur Respir J 2022;59:2101574. [Crossref] [PubMed]
- Sterne JAC, Savović J, Page MJ, et al. RoB 2: a revised tool for assessing risk of bias in randomised trials. BMJ 2019;366:l4898. [Crossref] [PubMed]
- Luo D, Wan X, Liu J, et al. Optimally estimating the sample mean from the sample size, median, mid-range, and/or mid-quartile range. Stat Methods Med Res 2018;27:1785-805. [Crossref] [PubMed]
- Wan X, Wang W, Liu J, et al. Estimating the sample mean and standard deviation from the sample size, median, range and/or interquartile range. BMC Med Res Methodol 2014;14:135. [Crossref] [PubMed]
- Higgins JP, Thompson SG, Deeks JJ, et al. Measuring inconsistency in meta-analyses. BMJ 2003;327:557-60. [Crossref] [PubMed]
- Guyatt GH, Oxman AD, Vist GE, et al. GRADE: an emerging consensus on rating quality of evidence and strength of recommendations. BMJ 2008;336:924-6. [Crossref] [PubMed]
- Wetterslev J, Jakobsen JC, Gluud C. Trial Sequential Analysis in systematic reviews with meta-analysis. BMC Med Res Methodol 2017;17:39. [Crossref] [PubMed]
- Ansari BM, Hogan MP, Collier TJ, et al. A Randomized Controlled Trial of High-Flow Nasal Oxygen (Optiflow) as Part of an Enhanced Recovery Program After Lung Resection Surgery. Ann Thorac Surg 2016;101:459-64. [Crossref] [PubMed]
- Yu Y, Qian X, Liu C, et al. Effect of High-Flow Nasal Cannula versus Conventional Oxygen Therapy for Patients with Thoracoscopic Lobectomy after Extubation. Can Respir J 2017;2017:7894631. [Crossref] [PubMed]
- Zhu X, Wang X, Jin X, et al. Efficacy Analysis of High-flow Nasal Oxygen Therapy in Patients Accepting Single-port Video-assisted Thoracoscopic Lobectomy. Zhongguo Fei Ai Za Zhi 2022;25:642-50. [PubMed]
- El-Nori AA, El-Sayed HH, Mostafa AMM, et al. A randomized controlled trial to compare the outcome of high-flow oxygen versus conventional oxygen in extubated patients after lung resection. The Egyptian Journal of Surgery 2023;41:1428-35.
- Hernández G, Vaquero C, Colinas L, et al. Effect of Postextubation High-Flow Nasal Cannula vs Noninvasive Ventilation on Reintubation and Postextubation Respiratory Failure in High-Risk Patients: A Randomized Clinical Trial. JAMA 2016;316:1565-74. [Crossref] [PubMed]
- Hernández G, Vaquero C, González P, et al. Effect of Postextubation High-Flow Nasal Cannula vs Conventional Oxygen Therapy on Reintubation in Low-Risk Patients: A Randomized Clinical Trial. JAMA 2016;315:1354-61. [Crossref] [PubMed]
- Chaudhuri D, Granton D, Wang DX, et al. High-Flow Nasal Cannula in the Immediate Postoperative Period: A Systematic Review and Meta-analysis. Chest 2020;158:1934-46. [Crossref] [PubMed]
- Gottlieb J, Capetian P, Hamsen U, et al. German S3 Guideline: Oxygen Therapy in the Acute Care of Adult Patients. Respiration 2022;101:214-52. [Crossref] [PubMed]
- Maggiore SM, Idone FA, Vaschetto R, et al. Nasal high-flow versus Venturi mask oxygen therapy after extubation. Effects on oxygenation, comfort, and clinical outcome. Am J Respir Crit Care Med 2014;190:282-8. [Crossref] [PubMed]
- Uygur P, Oktem S, Boran P, et al. Low- versus high-flow oxygen delivery systems in children with lower respiratory infection. Pediatr Int 2016;58:49-52. [Crossref] [PubMed]
- Ospina-Tascón GA, Calderón-Tapia LE, García AF, et al. Effect of High-Flow Oxygen Therapy vs Conventional Oxygen Therapy on Invasive Mechanical Ventilation and Clinical Recovery in Patients With Severe COVID-19: A Randomized Clinical Trial. JAMA 2021;326:2161-71. [Crossref] [PubMed]
- Crimi C, Noto A, Madotto F, et al. High-flow nasal oxygen versus conventional oxygen therapy in patients with COVID-19 pneumonia and mild hypoxaemia: a randomised controlled trial. Thorax 2023;78:354-61. [Crossref] [PubMed]