Optimal methodology for percutaneous dilatational tracheostomy: a comparative analysis between conventional and multidisciplinary approaches utilizing ultrasound, flexible bronchoscopy, and microcatheter puncture in critically ill individuals of diminutive stature—a longitudinal single-institutional experience and retrospective analysis
Highlight box
Key findings
• Percutaneous dilatational tracheostomy (PDT) employing Ciaglia Blue Rhino, ultrasound guidance, flexible bronchoscopy, and 4.0-French (4.0-Fr) microcatheter puncture appears to demonstrate efficacy in individuals facing respiratory challenges, particularly those of shorter stature, and this approach may contribute to the avoidance of complications carrying potential morbidity and mortality.
What is known and what is new?
• In the context of PDT, it is noteworthy that a majority of devices and techniques have been tailored for Western patients, who generally exhibit larger physical dimensions compared to their Korean counterparts.
• Our ideal approach distinctly exhibited a smaller tracheostomy wound, less evidence of bleeding, and a tidier tracheostomy track by the time of the T-tube change at the 7-day mark, despite encountering increased difficulty in accessing the site due to the smaller stature of patients.
What is the implication, and what should change now?
• PDT utilizing a Ciaglia Blue Rhino, conducted through a collaborative effort of a multidisciplinary team, and employing minimal access through the assistance of pre- and intra-operative ultrasound, concurrent flexible bronchoscopy, and initial 4.0-Fr diameter microcatheter puncture, appears to be beneficial for patients experiencing respiratory issues. This approach can be successfully applied to smaller Korean patients, ensuring a more secure procedure with minimized potential for serious complications and without associated morbidity and mortality.
Introduction
Many patients requiring prolonged mechanical ventilation often undergo to facilitate the separation of the ventilator. Tracheotomy offers various benefits, including a reduction in infection rates, lung clearance, improved oral health, enhanced comfort, airway protection, prevention of fistula formation, decreased complications associated with endotracheal tubes, and the ability to resume oral nutrition. Additionally, tracheostomy facilitates easy nursing care, respiratory tract hygiene, improved communication, and reductions in dead space, respiratory effort, and sedative medication use (1,2). Despite the advantages associated with tracheostomy in cases of prolonged mechanical ventilation, the optimal timing for this procedure remains a topic of controversy. Traditionally, tracheostomies are often performed when a patient has been intubated for more than 10–14 days. Although the debate over the appropriate timing continues, it is generally considered after 14–21 days of intubation. Early tracheostomy, performed before this period, has shown potential in reducing complications, morbidity, and mortality (3). Numerous studies have demonstrated that early tracheostomy in mechanically ventilated patients is linked to a shortened weaning time (4-6). However, regardless of the timing of tracheostomy, the procedure carries inherent risks, including local bleeding, pneumothorax, infections, recurrent laryngeal nerve injury, loss of airway control, and potential morbidity/mortality. A recent systematic review and meta-analysis reported all-cause mortality rates of 40.9% for the early tracheostomy group and 45.1% for the late tracheostomy group in non-neurologically injured adult patients (7). While tracheostomy itself is a well-established surgical approach with a long history, it demands considerable experiences and surgical skills. In 1985, in order to overcome these difficulties in tracheostomy, Ciaglia et al. (8), a thoracic surgeon with 48 years of experience at St. Elizabeth’s Hospital in Utica, NY, introduced a significant advancement to overcome the challenges associated with tracheostomy. They reported the first bedside percutaneous dilatational tracheostomy (PDT) using multiple sequentially larger dilators over a guidewire, known as “the serial dilatational technique”. They stressed the importance of meticulous inspection and palpation to identify both the thyroid cartilage and the cricoid. This process entailed making a vertical incision, delicately manipulating the tissues with a mosquito clamp, inserting a fine needle through the space, placing a flexible guide wire, and sequentially dilating the trachea using a series of increasingly larger dilators over the guide wire to gradually enlarge the tracheal opening. This technique modified the level of tracheal interspace cannulation, incorporated concurrent bronchoscopy, and utilized a single tapered dilator. The insertion site was adjusted caudally from the cricoid cartilage by one or two tracheal interspaces. Ten years after the initial description, the Ciaglia Blue Rhino (Cook Critical Care, Bloomington, IN, USA), a single dilator, was introduced. We present this article in accordance with the TREND reporting checklist (available at https://jtd.amegroups.com/article/view/10.21037/jtd-24-172/rc).
Methods
Study subjects
A retrospective study design was employed to investigate the PDT program. Initiated in January 2010 at a single medical center, the program was conducted by a single investigator and focused on critically ill patients requiring prolonged ventilation support and necessitating ventilator disconnection. The PDT program adhered to three main principles: (I) safest approach: the procedure aimed to minimize disruption to essential medications, including antiplatelets (aspirin, clopidogrel, cilostazol, etc.) and anticoagulants (heparin, low molecular weight heparin, nafamostat, warfarin, non-vitamin K antagonist oral anticoagulants, etc.); (II) prompt access: PDT was performed without interrupting critical clinical processes such as extracorporeal membrane oxygenation (ECMO), continuous renal replacement therapy (CRRT), and hemodialysis, which are vital for patient survival and resuscitation and often require active anticoagulation; (III) multidisciplinary team approach: a collaborative effort involving various team members, including the main operator, assistant physician, scrub nurse, bronchoscopy specialist, airway keeper, and respiratory care supporter. To adhere to these principles, the following conceptions were implemented: (I) performance of PDT exclusively at the intensive care unit (ICU) bedside, without patient transfer to the operating theater, to ensure continuous treatment without interruption and eliminate transfer-related risks; (II) careful pre-operative evaluation of anatomical localization for PDT using ultrasound (Aplio400, Canon Medical, Inc., Otawara, Tochigi, Japan) and guiding lights from bronchoscopy to minimize adverse reactions; (III) active application of flexible bronchoscopy (BF-F260, Olympus Optical Co., Tokyo, Japan) for airway identification, secretion cleaning, proper withdrawal of the endotracheal tube, verification of trachea puncture site, checking for bleeding sites, and confirming the correct tracheal tube placement; (IV) minimal access and incision to avoid incorrect tracheal wall perforation and posterior tracheal mucosa, facilitated by preliminary tracheal puncture using 4.0-French (4.0-Fr) diameter microcatheters.
The most common indications for tracheostomy were acute respiratory failure and the need for prolonged mechanical ventilation, accounting for two-thirds of all cases. Traumatic or catastrophic neurologic insults requiring airway support or mechanical ventilation were also common reasons for tracheostomy in the ICU. Patients in this study, suffering from critical conditions such as prolonged mechanical ventilation due to severe respiratory problems from various causes, were included between January 1, 2010, and December 31, 2022. All patients were aged 18 years or older.
Critical preoperative considerations for PDT included the ability to hyperextend the neck, a minimum 1.0-cm distance between the inferior cricoid cartilage and the suprasternal notch, ensuring re-intubation capability in case of accidental extubation, and patient tolerance of transient hypercarbia and hypoxemia. It is emphasized that PDT is generally an elective procedure, necessitating careful preoperative evaluations and risk-benefit analysis for each patient. However, relative contraindications were described, dependent on the clinical context, including emergency tracheostomy tube placement, challenging anatomy (e.g., morbid obesity with a short neck, limited neck movement, cervical spine injury), pediatric cases, existing infections or malignancy in the tracheostomy area, unstable cervical spine fractures, uncertainty about anatomical orientation, previous neck surgeries, increased bleeding risk, deep-seated trachea, hemodynamic instability, hypoxemia [partial pressure of oxygen (PaO2)/fractional inspired oxygen (FiO2) <200], high intracranial pressure (>20 mmHg), multi-organ failure, coagulopathy, history of major cervical surgery altering cervical flexion-extension, abnormal cervical anatomy, age under 18 years, and the need for proximal or distal extension tracheostomy tube placement.
Preoperative preparation
It is recommended to optimize the following conditions prior to the start of the procedure:
- Corroboration of signed informed consent: ensuring that signed informed consent is obtained, which comprehensively covers a wide range of risks, potential outcomes of the procedure, and postoperative complications.
- Verification of adequate functioning of suction systems: confirming the proper functioning of suction systems to address any potential secretions or complications during the procedure.
- Setting of the ventilator in volume control mode: adjusting the ventilator to operate in volume control mode to achieve optimal and controlled ventilation during the procedure.
- Setting of respiratory rate adjusted to arterial pH: adjusting the respiratory rate to maintain arterial pH within the desired range, ensuring respiratory parameters are aligned with the patient’s physiological needs.
- 100% FiO2 for adequate pre-oxygenation: administering 100% FiO2 to ensure thorough pre-oxygenation, enhancing oxygen reserves in the patient before the procedure.
- Setting of ventilator alarms: configuring ventilator alarms to promptly detect and alert to any deviations from the desired respiratory parameters, ensuring immediate response to potential issues.
- Turning off the alternating pressure mattress: disabling the alternating pressure mattress to avoid interference with the procedure and to maintain a stable environment during the tracheostomy.
- Interscapular projection for greater cervical extension: employing an interscapular projection to achieve enhanced cervical extension, facilitating the procedural positioning and ensuring optimal access to the tracheostomy site.
These optimization measures contribute to the overall safety and effectiveness of the PDT procedure.
Surgical procedure
In a surgical procedure, PDT assisted by a multidisciplinary team collaboration (Figure 1) was conducted using minimal access with integrated multilateral assistance, incorporating pre- and intra-operative ultrasound, concurrent flexible bronchoscopy, and preliminary microcatheter puncture. The detailed technique for patient preparation is outlined below. The patient underwent a thorough examination for anatomical localization that could impact the procedure, guided by ultrasound and meticulous physical examination (Figures 2,3). Patient preparation included checking for proper positioning, verifying endotracheal tube size, administering adequate sedation/anesthesia, adjusting ventilator settings, confirming monitor alarms, and titrating external ambient light. The patient was positioned supine with a pillow under the shoulders, head and neck fully extended, and the head end of the bed elevated by 30°–40°. For patients with cervical spine issues, neck extension and head-down positioning were omitted, maintaining only the supine position. The tracheostomy area skin was disinfected and covered with sterile drapes. Patients were carefully sedated and continuously monitored per hospital guidelines. Bronchoscopic exploration of the airway, secretion aspiration, and withdrawal of the endotracheal tube to the subglottic cone without cuff deflation were performed with flexible bronchoscopic assistance. An antegrade single-step dilation technique with a curved dilator and loading dilator was executed through the following steps (Figures 4,5):
- Administration of 2% lidocaine in the region of interest.
- A 6.0-mm vertical incision at the placement site for skin dilation, followed by sharp dissection of subcutaneous tissue, opening of cutaneous platysma muscle using electrocautery, careful divulsion of pretracheal muscles, and accurate palpation of target tracheal rings.
- Identification of tracheal puncture site, guided by pre-operative ultrasound and meticulous physical examination, typically between the 2nd and 3rd, or 3rd and 4th tracheal rings, in a caudal direction.
- Preliminary puncture through the trachea using 4.0-Fr diameter microcatheters, hair wire, and angio-catheter, guided and confirmed by flexible bronchoscopy.
- Indwelling of microcatheters into the targeted trachea, followed by a change to a 15-gauge introducer needle with sheath, tilted at a 60º angle for confirmation by flexible bronchoscopy.
- Advancement of a 0.035 inches-diameter, 145 cm-length J-shaped guide wire through the introducer sheath, reaching the carina level.
- Removal of the introducer sheath, leaving the guide wire in place, and insertion of a 14.0-Fr diameter introducer dilator to dilate the puncture site, monitored by bronchoscopy.
- Insertion of a guiding catheter with a safety stop over the guide wire until the safety stop reaches the skin level.
- Insertion of a dilator over the guiding catheter, expanding the puncture channel carefully in coordination with the guiding catheter and guide wire.
- Removal of the dilator, ensuring accurate placement of the tracheostomy tube with a size 7.0 Portex (Smiths Medical Inc., Plymouth, MN, USA) cuffed tracheostomy tube, cuff inflation, connection to ventilator tubing, fixation, endoscopic verification, and dressing confirmation.
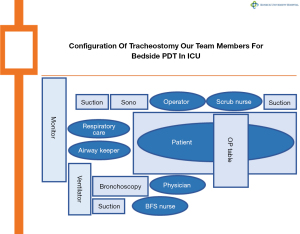
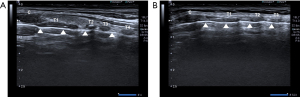
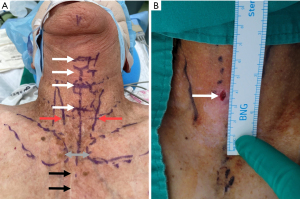

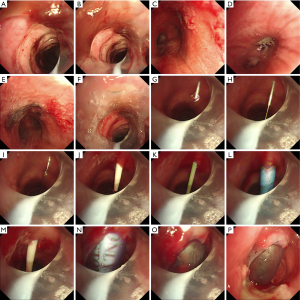
In the modified PDT groups, all surgical procedures were diligently executed, whereas in the conventional PDT groups, the identification of the tracheal puncture site guided by pre-operative ultrasound (surgical procedure #3) and the preliminary puncture through the trachea using 4.0-Fr diameter microcatheters (surgical procedure #4) were omitted. Additionally, bronchoscopy was employed sparingly.
Postoperative care
The postoperative care following the PDT procedure involved several key measures for optimization:
- Discarding procedural material: proper disposal of materials used during the procedure.
- Adjustment of FiO2 to patient’s requirements: fine-tuning of the FiO2 to meet the specific oxygenation needs of the patient.
- Resetting of respirator alarms: calibration and resetting of alarms on the respirator to ensure accurate monitoring and response to changes in respiratory parameters.
- Reevaluation of target sedation level: review and adjustment of the sedation level to align with the patient’s postoperative condition.
- Checking closed suction system adaptation: verification of the correct adaptation of the closed suction system to the tracheostomy cannula to maintain a closed and sterile environment during suction.
- Intermittent subglottic suction through tracheostomy cannula: periodic suctioning of the subglottic region through the tracheostomy cannula, utilizing a closed suction system to remove secretions and maintain airway patency.
- Checking control chest radiograph: conducting a thorough examination of a control chest radiograph to assess the positioning of the tracheostomy tube, evaluate lung expansion, and ensure there are no complications post-procedure.
- Assessment of tracheostomy tube cuff pressure using a pressure manometer: maintaining the cuff pressure of the tracheostomy tube at approximately 20–25 cmH2O.
Ethical statement
The study was conducted in accordance with the Declaration of Helsinki (as revised in 2013). The study was approved by the Institutional Review Board of Konkuk University Chungju Hospital (IRB approval No. KUCH 2021-11-018) and individual consent for this retrospective analysis was waived.
Statistical analysis
Statistical evaluations were performed using MedCalc software for Windows version 17.11.5 (MedCalc Software, Ostend, Belgium) and IBM SPSS Statistics software version 21 (IBM Corp., Armonk, NY, USA). Data collection and further analysis were performed using Microsoft Excel (Microsoft Corporation, Redmond, WA, USA). The normality of continuous variables was assessed using the Kolmogorov-Smirnov test, with normally distributed variables analyzed via the Student’s t-test, reported as arithmetic means ± standard deviations. Variables not normally distributed were evaluated using the Mann-Whitney U test and reported as medians with interquartile ranges (25th–75th percentiles). Categorical variables were represented as frequency distributions and analyzed either by Pearson’s Chi-square test or Fisher’s exact test, depending on appropriateness. Chi-square or Fisher’s exact test was also used for univariate comparisons of categorical data between groups. To control for type I errors, Bonferroni post-hoc correction (B-corrected) was applied by adjusting the P values of significant findings based on the number of comparisons. The Cox proportional hazards model was utilized to determine independent predictors of successful weaning, while overall survival rates were computed using the Kaplan-Meier estimator. The same Cox model was also used to ascertain independent predictors of overall survival. A significance threshold was established at P<0.05. Univariate and multivariate stepwise logistic regression analyses were conducted to identify factors independently associated with patient mortality. Backward stepwise logistic regression was employed in the multivariate analysis. Variables showing a significance level of P<0.20 in the univariate analysis, as well as clinically significant variables, were considered as independent predictors in the multivariate model. The resulting data were presented as odds ratios (ORs) with standard errors (SEs), 95% confidence intervals (CI), and the corresponding P values. To evaluate the accuracy of the logistic regression model, receiver operating characteristic (ROC) curves were generated, and the area under the curve (AUC) was calculated. The Hosmer-Lemeshow test was used to check the fit of the logistic model by comparing observed versus predicted mortality across varying probabilities of death. The effectiveness of the model in differentiating outcomes was assessed via the area under the ROC curve. Kaplan-Meier survival curves were plotted over time and compared across groups using the log-rank test.
Results
We identified a total of 183 eligible cases for analysis based on the specified criteria at our institution between January 1, 2010, and October 31, 2021. The detailed demographic and clinical characteristics of the study participants are summarized, and a comprehensive comparison between modified and conventional PDT is presented in Tables 1-3. Among the 183 patients (128 males and 55 females), 133 were assigned to the modified PDT group (92 males and 41 females), while 50 patients were categorized into the conventional PDT group (36 males and 14 females).
Table 1
Demographics | Modified (N=133) | Conventional (N=50) | Total (N=183) | P |
---|---|---|---|---|
Age (years) | 69.5±14.2 | 63.5±14.1 | 67.8±14.4 | 0.01* |
Sex | 0.84 | |||
Male | 92 (69.2) | 36 (72.0) | 128 (69.9) | |
Female | 41 (30.8) | 14 (28.0) | 55 (30.1) | |
Body weight (kg) | 67.9±11.6 | 65.3±10.1 | 67.1±11.3 | 0.16 |
Height (cm) | 166.6±8.7 | 167.8±9.4 | 167.0±8.9 | 0.41 |
BMI (kg/m2) | 24.5±4.2 | 23.3±4.2 | 24.2±4.2 | 0.09 |
Obesity classification | 0.36 | |||
Normal | 56 (42.1) | 27 (54.0) | 83 (45.4) | |
Overweight | 16 (12.0) | 7 (14.0) | 23 (12.6) | |
Obesity | 46 (34.6) | 11 (22.0) | 57 (31.1) | |
Severe obesity | 15 (11.3) | 5 (10.0) | 20 (10.9) |
Values are expressed as mean ± standard deviation or n (%). Normal: BMI 18.5–22.9 kg/m2; overweight: BMI 23–24.9 kg/m2; obesity: BMI 25–29.9 kg/m2; severe obesity: BMI ≥30 kg/m2. *, P<0.05. PDT, percutaneous dilatational tracheostomy; BMI, body mass index.
Table 2
Parameters | Modified (N=133) | Conventional (N=50) | Total (N=183) | P |
---|---|---|---|---|
Survival | 0.01* | |||
Survivor | 41 (30.8) | 26 (52.0) | 67 (36.6) | |
Non-survivor | 92 (69.2) | 24 (48.0) | 116 (63.4) | |
SOFA | 7.1±2.3 | 6.7±2.3 | 7.0±2.3 | 0.31 |
SAPS II | 46.7±9.0 | 44.0±9.1 | 45.9±9.1 | 0.08 |
HD (days) | 50.4±40.0 | 62.7±62.9 | 53.7±47.5 | 0.20 |
POD (days) | 33.4±34.9 | 39.9±56.8 | 35.2±42.0 | 0.44 |
ICU stay (days) | 34.8±29.8 | 47.6±39.9 | 38.3±33.2 | 0.043* |
Days for PDT after intubation (days) | 12.4±8.9 | 15.7±11.3 | 13.3±9.7 | 0.06 |
Ventilator weaning | 0.07 | |||
Success | 77 (57.9) | 21 (42.0) | 98 (53.6) | |
Failure | 56 (42.1) | 29 (58.0) | 85 (46.4) | |
Days for ventilator weaning after PDT (days) | 6.9±10.1 | 9.0±10.3 | 7.4±10.2 | 0.40 |
Underlying disease | 0.74 | |||
Underlying DM | 36 (27.1) | 16 (32.0) | 52 (28.6) | 0.57 |
Underlying HTN | 66 (49.6) | 19 (38.0) | 85 (46.7) | 0.25 |
Underlying COPD | 6 (4.5) | 3 (6.0) | 9 (4.9) | 0.97 |
Underlying CVD | 20 (15.0) | 11 (22.0) | 31 (16.9) | 0.36 |
Underlying ESRD | 12 (9.0) | 7 (14.0) | 19 (10.4) | 0.47 |
Underlying CAD | 3 (2.3) | 3 (6.0) | 6 (3.3) | 0.42 |
Underlying SOM | 12 (9.0) | 4 (8.0) | 16 (8.7) | >0.99 |
Underlying A. Fib | 9 (6.8) | 6 (12.0) | 15 (8.2) | 0.39 |
Underlying CHF | 10 (7.5) | 9 (18.0) | 19 (10.4) | 0.07 |
Underlying DVT/PE | 3 (2.3) | 2 (4.0) | 5 (2.7) | 0.89 |
Underlying LC | 5 (3.8) | 3 (6.0) | 8 (4.4) | 0.79 |
Concurrent medications | ||||
Heparin | 13 (9.8) | 4 (8.0) | 17 (9.3) | 0.93 |
LMWH | 41 (30.8) | 13 (26.0) | 54 (29.5) | 0.64 |
Warfarin | 5 (3.8) | 4 (8.0) | 9 (4.9) | 0.42 |
Aspirin | 18 (13.5) | 4 (8.0) | 22 (12.0) | 0.44 |
Clopidogrel | 6 (4.5) | 3 (6.0) | 9 (4.9) | 0.97 |
Surgical time (minutes) | 16.7±4.9 | 15.9±5.1 | 16.5±4.9 | 0.32 |
Total surgical time (minutes) | 25.6±7.5 | 19.9±6.5 | 24.1±7.6 | <0.001* |
Bleeding | ||||
Major bleeding | 0 | 0 | 0 | >0.99 |
Minor bleeding | 2 (1.5) | 7 (14.0) | 9 (4.9) | 0.002* |
Wound infection | 1 (0.8) | 2 (4.0) | 3 (1.6) | 0.37 |
Stromal inflammation | 2 (1.5) | 3 (6.0) | 5 (2.7) | 0.24 |
Previous median sternotomy | 3 (2.3) | 2 (4.0) | 5 (2.7) | 0.89 |
Success of first tracheal puncture | 133 (100.0) | 46 (92.0) | 179 (97.8) | 0.006* |
Intra-OP bleeding | 0 | 3 (6.0) | 3 (1.6) | 0.02* |
Conversion to surgical tracheostomy | 0 | 0 | 0 | >0.99 |
Failure of tracheal puncture | 0 | 0 | 0 | >0.99 |
Mortality during PDT | 0 | 0 | 0 | >0.99 |
Cardiac arrest during PDT | 0 | 0 | 0 | >0.99 |
Atelectasis after PDT | 0 | 0 | 0 | >0.99 |
ECMO | 3 (2.3) | 0 | 3 (1.6) | 0.67 |
CRRT | 6 (4.5) | 2 (4.0) | 8 (4.4) | >0.99 |
Values are expressed as mean ± standard deviation or n (%). *, significance at P<0.05. PDT, percutaneous dilatational tracheostomy; SOFA, sequential organ failure assessment; SAPS, simplified acute physiology score; HD, hospital day; POD, postoperative day; ICU, intensive care unit; DM, diabetes mellitus; HTN, hypertension; COPD, chronic obstructive pulmonary disease; CVD, cerebrovascular disease; ESRD, end-stage renal disease; CAD, coronary artery disease; SOM, solid organ malignancy; A. Fib, atrial fibrillation; CHF, congestive heart failure; DVT, deep vein thrombosis; PE, pulmonary embolism; LC, liver cirrhosis; LMWH, low-molecular-weight heparin; OP, operation; ECMO, extracorporeal membrane oxygenation; CRRT, continuous renal replacement therapy.
Table 3
Parameters | Modified (N=133) | Conventional (N=50) | Total (N=183) | P |
---|---|---|---|---|
WBC (×103/μL) | 11.8±5.0 | 12.5±5.7 | 12.0±5.2 | 0.40 |
RBC (×106/μL) | 3.3±0.6 | 3.3±0.4 | 3.3±0.5 | 0.66 |
Hemoglobin (g/dL) | 10.1±1.7 | 10.1±1.4 | 10.1±1.7 | 0.90 |
Hematocrit (%) | 30.6±5.1 | 30.0±4.3 | 30.4±4.9 | 0.50 |
Platelet (×103/μL) | 236.9±151.4 | 220.2±140.9 | 232.4±148.4 | 0.49 |
Neutrophil (%) | 80.3±9.2 | 81.1±9.1 | 80.6±9.2 | 0.60 |
PT (seconds) | 14.5±2.6 | 14.3±2.3 | 14.4±2.5 | 0.60 |
aPTT (seconds) | 38.2±12.4 | 41.8±22.3 | 39.2±15.8 | 0.27 |
INR | 1.3±0.3 | 1.4±0.3 | 1.3±0.3 | 0.15 |
Fibrinogen (mg/dL) | 394.3±199.5 | 355.3±183.2 | 383.6±195.5 | 0.23 |
Na (mmol/L) | 138.7±6.7 | 136.9±6.6 | 138.2±6.7 | 0.10 |
K (mmol/L) | 3.7±0.6 | 3.7±0.5 | 3.7±0.6 | 0.90 |
Cl (mmol/L) | 102.6±6.9 | 103.2±6.7 | 102.7±6.8 | 0.60 |
pH (ABGA) | 7.5±0.1 | 7.5±0.1 | 7.5±0.1 | 0.39 |
pO2 (ABGA) (mmHg) | 113.5±36.3 | 115.5±32.0 | 114.0±35.1 | 0.72 |
pCO2 (ABGA) (mmHg) | 40.3±13.6 | 39.1±9.0 | 39.9±12.5 | 0.48 |
cHCO3 (ABGA) (mmHg) | 28.2±6.2 | 27.0±5.0 | 27.9±5.9 | 0.22 |
Base excess (ABGA) (mmol/L) | 4.0±5.3 | 2.9±4.6 | 3.7±5.1 | 0.19 |
SpO2 (ABGA) (%) | 97.1±4.7 | 97.5±3.3 | 97.2±4.4 | 0.51 |
Glucose | 138.7±47.5 | 129.2±27.7 | 136.1±43.2 | 0.09 |
iCa (mg/dL) | 2.4±1.4 | 1.0±0.6 | 2.0±1.4 | <0.001* |
Lactate (mmol/L) | 1.2±0.7 | 1.2±0.5 | 1.2±0.6 | 0.80 |
AST (IU/L) | 50.8±52.0 | 43.8±40.1 | 48.9±49.0 | 0.33 |
ALT (IU/L) | 46.5±54.3 | 32.4±25.5 | 42.6±48.5 | 0.01* |
Total bilirubin (mg/dL) | 1.9±3.5 | 1.5±1.2 | 1.8±3.1 | 0.18 |
Total protein (g/dL) | 5.7±0.8 | 5.7±0.9 | 5.7±0.8 | 0.94 |
Albumin (g/dL) | 2.7±0.6 | 2.5±0.7 | 2.7±0.7 | 0.040* |
BUN (mg/dL) | 26.8±20.8 | 25.6±12.8 | 26.5±19.0 | 0.64 |
Creatinine (mg/dL) | 0.9±0.8 | 0.8±0.4 | 0.9±0.7 | 0.17 |
CRP (mg/dL) | 9.3±6.6 | 8.7±5.5 | 9.1±6.3 | 0.58 |
Procalcitonin (ng/mL) | 0.7±1.6 | 1.1±1.3 | 0.7±1.5 | 0.77 |
Values are expressed as mean ± standard deviation. *, P<0.05. PDT, percutaneous dilatational tracheostomy; WBC, white blood cell; RBC, red blood cell; PT, prothrombin time; aPTT, activated partial thromboplastin time; INR, international normalized ratio; pO2, partial pressure of oxygen; pCO2, partial pressure of carbon dioxide; ABGA, arterial blood gas analysis; iCa, ionized calcium; SpO2, O2 saturation; AST, aspartate aminotransferase; ALT, alanine aminotransferase; IU, international unit; BUN, blood urea nitrogen; CRP, C-reactive protein.
The age was found to be higher in the modified PDT group compared to the conventional PDT group, exhibiting a statistically significant difference in age (69.5±14.2 vs. 63.5±14.1 years, P=0.01). Baseline parameters in the modified and conventional PDT groups were similar, with no significant differences observed in sex distribution (male/female: 92/41 vs. 36/14, P=0.84), body weight (67.9±11.6 vs. 65.3±10.1 kg, P=0.16), height (166.6±8.7 vs. 167.8±9.4 cm, P=0.41), body mass index (24.5±4.2 vs. 23.3±4.2 kg/m2, P=0.09), obesity classification (normal/overweight/obesity/severe obesity: 56/16/46/15 vs. 27/7/11/5, P=0.36), sequential organ failure assessment (SOFA) (7.1±2.3 vs. 6.7±2.3, P=0.31), and simplified acute physiology score (SAPS) II (46.7±9.0 vs. 44.0±9.1, P=0.08), days for PDT after intubation (12.4±8.9 vs. 15.7±11.3 days, P=0.06), ventilator weaning (P=0.07), and underlying disease (P=0.74). The survival rate was higher in the conventional PDT group, showing a significant difference between the modified and conventional PDT groups [41/133 (30.8%) vs. 26/50 (52.0%), P=0.01]. Hospital days, post-operative days, and days for PDT after intubation were longer in the conventional PDT group, but these differences were not statistically significant between the modified and conventional PDT groups (50.4±40.0 vs. 62.7±62.9 days, P=0.20; 33.4±34.9 vs. 39.9±56.8 days, P=0.44; 12.4±8.9 vs. 15.7±11.3 days, P=0.06, respectively). The ICU stay days were longer in the conventional PDT group, showing a significant difference between the modified and conventional PDT groups (34.8±29.8 vs. 47.6±39.9 days, P=0.043). There was no statistically significant difference between the modified and conventional PDT groups in concurrent medications, such as heparin, low-molecular-weight heparin (LMWH), warfarin, aspirin, and clopidogrel [13 (9.8%) vs. 4 (8.0%), P=0.93; 41 (30.8%) vs. 13 (26.0%), P=0.64; 5 (3.8%) vs. 4 (8.0%), P=0.42; 18 (13.5%) vs. 4 (8.0%), P=0.44; 6 (4.5%) vs. 3 (6.0%), P=0.97, respectively]. No definite statistically significant difference was observed between the modified and conventional PDT groups in surgical time, major bleeding, wound infection, and stromal inflammation (Table 2). Minor bleeding was lower in the modified PDT group than in the conventional PDT group [2 (1.5%) vs. 7 (14.0%), P=0.002]. Furthermore, the total surgical time was longer in the modified PDT group, and the success rate of the first tracheal puncture was higher in the modified PDT group, both of which showed a statistically significant difference between the modified and conventional PDT groups [25.6±7.5 vs. 19.9±6.5 minutes, P<0.001; 133 (100.0%) vs. 46 (92.0%), P=0.006, respectively]. In both groups, there were no cases of conversion to surgical tracheostomy, failure of tracheal puncture, mortality during PDT, cardiac arrest during PDT, atelectasis after PDT, or the need for accompanying ECMO and CRRT (Table 2). Intra-OP bleeding was only observed in the conventional PDT group [0 vs. 3 (6.0%), P=0.02]. There was no definite statistically significant difference between the modified and conventional PDT groups in laboratory findings, including complete blood cell count with differential count, coagulation parameters, electrolyte levels, arterial blood gas analysis, and chemical makers such as glucose, ionized calcium (iCa), lactate, aspartate aminotransferase (AST) [glutamate oxaloacetate transaminase (GOT), alanine aminotransferase (ALT) [glutamate pyruvate transaminase (GPT)], total bilirubin, total protein, blood urea nitrogen (BUN), creatinine, and inflammation markers such as C-reactive protein (CRP) and procalcitonin. However, the levels of albumin were significantly lower in the conventional PDT group than in the modified PDT group (2.7±0.6 vs. 2.5±0.7 g/dL, P=0.040) (Table 3).
These results suggest that modified PDT may be a more reliable approach than conventional PDT, despite facing various challenges such as poor general condition with high SAPS II and SOFA, relatively long total surgical time, and concurrent aspirin/heparin medication. Our study also indicates that modified PDT might be a less harmful surgical technique, with fewer instances of bleeding, wound infection, and stromal inflammation. Additionally, it reveals a superior success rate of the first tracheal puncture without major complications.
Discussion
Tracheotomy, a procedure involving the temporary opening of the trachea, entails the exteriorization of the trachea to the skin of the neck, resulting in the creation of a more permanent fistula and opening. The earliest known depiction of tracheostomy dates back to 3600 BC, found on Egyptian tablets. The first scientifically reliable description of a successful tracheostomy was provided by Antonio Musa Brasavola in 1546, aimed at relieving airway obstruction caused by enlarged tonsils. In the early 20th century, significant advancements were made in tracheostomy safety, with detailed technical descriptions provided by the renowned surgeon Chevalier Jackson. Annually, over 800,000 residents in the United States undergo mechanical ventilation for acute respiratory insufficiency, often for days or weeks. The average number of tracheostomies performed in the United States exceeds 100,000 (1,9). Traditionally, conventional tracheostomy was carried out by surgeons in the operating room. However, advancements in techniques have made it a routine procedure feasible in most ICUs. Surgical open tracheostomy involves a 3-cm vertical skin incision below the inferior cricoid cartilage, lateral retraction of the strap muscles, and superior or inferior retraction or division of the thyroid isthmus. An incision in the anterior trachea at the first or second tracheal rings, with a sideways “H” incision at the level of the second tracheal ring, is considered ideal, providing an open-book exposure without resection. Tracheostomy, whether performed as a form of bedside ICU percutaneous dilatation tracheostomy or surgical tracheostomy in the operating room, serves as a critical procedure (10). Surgical tracheostomy in the operating room requires anesthesia, skin incision, tracheal ring cutting, and direct placement of the tracheostomy tube. It may lead to early complications, including cuff leak, tracheal obstruction, bleeding, and fistula (4). PDT contributes to the reduction of overall tracheostomy procedure time by eliminating the need for transportation to the operating room and the associated waiting time, as well as the risks associated with general anesthesia. PDT is increasingly considered the procedure of choice due to its efficiency and cost-effectiveness (11-13). Several high-quality prospective randomized studies support the superiority of PDT over surgical techniques in critically ill patient (14-17). In the evolution of percutaneous tracheostomy, the Ciaglia single dilator technique, an improvement over the original serial dilatational technique, employs a simpler kit with a single-beveled curved hydrophilic dilator. This modification significantly reduces tidal volume loss during the procedure, as there is no need for serial changes of the dilator. Griggs et al. introduced the guidewire dilator forceps tracheostomy technique in 1990, using a modified Howard Kelly forceps over the guidewire for a single-step dilatation of the tracheostomy site. Following dilatation, a tracheostomy tube is introduced over the guidewire. Despite a higher incidence of soft tissue damage, this technique, due to the reusability of forceps and the absence of a need for a specialized kit, remains potentially useful in situations of resource constraints (18,19). In 1997, Fantoni and Ripamonti introduced the translaryngeal tracheostomy, a procedure requiring a highly skilled operator. This technique involves a retrograde passage of a guidewire from the vocal cords to a needle puncture site in the trachea. The dilator and tracheostomy tube are then railroaded over the guidewire into the larynx, out through the anterior tracheal wall, and finally rotated by 180°, positioning the tracheostomy tube facing the carina (20). In 2002, Frova’s percutaneous tracheostomy introduced a single-step screw-type dilator, commercially available as the PercuTwistTM percutaneous tracheostomy kit. The dilator is rotated clockwise using a lifting motion over the guidewire. Unfortunately, this technique is associated with a higher risk of tracheal ring fractures and damage to the posterior tracheal wall (21). Balloon dilatational tracheostomy with Ciaglia Blue Dolphin (Cook Critical Care, Bloomington, IN, USA), a technical revolution of the original Ciaglia technique, inflates a modified angioplasty balloon over a guidewire with saline to 11 atmospheric pressure for 15 seconds. Following deflation, the tracheostomy tube is inserted in a single-staged approach. This technique offers several advantages over conventional Ciaglia single dilator tracheostomy, including the absence of the need for dilator removal before inserting the tracheostomy tube through the stroma and minimized pressure on the tracheal wall during balloon dilation (22). Comparisons of different PDT techniques reveal unique characteristics and advantages for each. A meta-analysis in 2012, involving 13 studies comparing various PDT techniques, indicated no major differences except for Fantoni’s technique, which was associated with more serious complications necessitating a switch to an alternative PDT technique. Ciaglia Blue Rhino technique showed better outcomes than other PDT techniques, such as PercuTwist, Blue Dolphin, and Griggs technique (1,23-26).
Recent advances in technology have led to the evolution of different techniques for PDT, promoting minimally invasive procedures. The duration of ventilation and the length of ICU stay after percutaneous dilatation tracheostomy are significantly reduced (27). Worldwide, percutaneous dilatation tracheostomy has gained widespread acceptance as the preferred method for tracheostomy in critically ill patients (28,29). Delaney et al. have suggested that PDT reduces the incidence of wound infection, clinically relevant bleeding, and mortality compared to surgical tracheostomy (30). Another study by Youssef et al. has revealed the safety and effectiveness of PDT, with low incidence of post-operative complications, comparable to classical surgical tracheostomy (17). Several types of PDT techniques have been described, often performed with fiberoptic bronchoscopy guidance for precision and safety. The single dilation technique, particularly in the Ciaglia and Griggs procedures from the 1980s and 1990s, has been widely used. Recent evidence supports the notion that the single dilation technique enhances safety and achieves higher success rates compared to the guidewire dilator forceps tracheostomy technique (23,31).
Our investigation underscores the utility of ultrasound guidance throughout the entire PDT procedure, especially in challenging cases. Ultrasound proves especially beneficial in ensuring accurate anatomical landmarks in patients with unique neck characteristics or comorbidities. Additionally, the use of flexible bronchoscopy during PDT is highlighted as a valuable tool for facilitating the surgical process. This includes direct observation of the surgical field, securing the airway, ensuring adequate oxygen supply, preventing endotracheal tube displacement, and reducing intra-tracheostomy complications. This study underscores the critical role of pre-tracheostomy ultrasound evaluation for precise anatomical landmark confirmation. Key anatomical structures, including surface landmarks such as the hyoid bone, thyroid cartilage, cricothyroid ligament, cricoid cartilage, thyroid gland, and sternohyoid muscle, are accurately identified. The confirmation of tracheal cartilage rings, layers of dissection, and vessels to be avoided during tracheostomy is highlighted, emphasizing the importance of careful evaluation. The study emphasizes the value of ultrasound guidance throughout the entire PDT procedure, particularly in specific patient populations. This includes cases with extremely short thick necks, a history of neck surgery, and those at high risk of bleeding due to antiplatelet (e.g., aspirin, clopidogrel, ticagrelor, prasugrel, dipyridamole, ticlopidine) and anticoagulation medications (e.g., heparin, warfarin, rivaroxaban, dabigatran, apixaban, edoxaban, enoxaparin, fondaparinux). The precise identification of trachea diameter/depth for the tracheostomy site is noted as a crucial aspect of ultrasound-guided PDT. Furthermore, the study strongly advocates for the use of flexible bronchoscopy during PDT, emphasizing its facilitative role in the surgical process. Flexible bronchoscopy enables direct observation of the surgical field, ensures a secure airway tract, aids in cases of neurological disease and traumatic brain injury, and helps prevent complications such as endotracheal tube displacement or deflation. The application of flexible bronchoscopy is suggested to reduce intra-tracheostomy complications, including posterior tracheal wall perforation, esophageal injury, airway fire, bleeding, pneumothorax, and pneumomediastinum.
In conclusion, this investigation highlights the indispensable nature of pre-tracheostomy ultrasound evaluation and the advantageous use of ultrasound guidance and flexible bronchoscopy in PDT procedures. These approaches are deemed especially beneficial in challenging cases and patient populations with specific characteristics, ultimately contributing to enhanced safety and reduced intra-tracheostomy complications.
The flexible bronchoscopy guidance in PDT helps with meticulous preparation, patient positioning, assessment of orotracheal tube size, selection of an appropriate bronchoscope size, administration of adequate anesthesia, and adjustment of ventilator parameters. The guidance also allows for a thorough pre-procedural check of airway anatomy, transillumination of the desired puncture site, and continuous endoscopic monitoring of the procedure. Post-procedural checks, such as confirming tracheostomy tube insertion, assessing correct airway positioning, checking for bleeding points or cartilage fractures, and suctioning blood clots and secretions, are facilitated by flexible bronchoscopy. In PDT operations using the single-stage dilator technique with Ciaglia blue rhino®, employing a microcatheter pre-puncture with a fine needle guided by flexible bronchoscopy in the initial approach significantly reduces the risk of inappropriate tracheal puncture and puncture site bleeding. Even in the case of a failure in the first puncture, the procedure can be safely continued with a low risk of puncture site bleeding.
However, the study has certain limitations. It was conducted at a single institution with a single investigator, limiting the generalizability of the results. The study’s retrospective and observational nature introduces potential residual confounding, and the small, heterogeneous study population may limit statistical significance. Another limitation is that only minor complications were significantly reduced by the modified technique, and this suggests potential safety improvements but underscores the necessity for larger-scale studies to assess the reduction in major complications. Generalizing the results to other indications for tracheostomy and diverse clinical situations is challenging. Multicenter, multinational, randomized, controlled trials, and prospective cohort studies are recommended to validate these approaches, particularly in different patient populations and clinical settings.
Conclusions
The conclusive results of our study underscore the advantages of PDT with a Ciaglia Blue Rhino, conducted through a collaborative effort involving a multidisciplinary team. Utilizing minimal access techniques, including pre- and intra-operative ultrasound, concurrent flexible bronchoscopy, and a preliminary 4.0-Fr diameter microcatheter puncture, the procedure demonstrates several notable benefits. Despite the inherent challenge of accessing small-bodied Korean patients, our study reveals a smaller tracheostomy wound, reduced bleeding, and a more orderly tracheostomy track observed at the 7-day follow-up during the t-tube change. This meticulously guided approach to PDT is suggested to be especially beneficial for patients with respiratory issues, particularly among smaller Korean patients. The procedure can be executed with enhanced safety, minimizing the occurrence of serious complications and avoiding morbidity and mortality. The multidisciplinary collaboration and the incorporation of advanced techniques, such as ultrasound guidance and flexible bronchoscopy, contribute significantly to the overall success of the procedure. In summary, the study highlights the efficacy and safety of the proposed PDT approach, emphasizing its potential benefits for smaller Korean patients facing respiratory challenges. The integration of advanced technologies and a multidisciplinary team approach plays a crucial role in ensuring minimized complications and improved patient outcomes.
Acknowledgments
Funding: This paper was supported by
Footnote
Reporting Checklist: The authors have completed the TREND reporting checklist. Available at https://jtd.amegroups.com/article/view/10.21037/jtd-24-172/rc
Data Sharing Statement: Available at https://jtd.amegroups.com/article/view/10.21037/jtd-24-172/dss
Peer Review File: Available at https://jtd.amegroups.com/article/view/10.21037/jtd-24-172/prf
Conflicts of Interest: All authors have completed the ICMJE uniform disclosure form (available at https://jtd.amegroups.com/article/view/10.21037/jtd-24-172/coif). All authors report that this paper was supported by Konkuk University in 2021. The authors have no other conflicts of interest to declare.
Ethical Statement: The authors are accountable for all aspects of the work in ensuring that questions related to the accuracy or integrity of any part of the work are appropriately investigated and resolved. The study was conducted in accordance with the Declaration of Helsinki (as revised in 2013). The study was approved by the Institutional Review Board of Konkuk University Chungju Hospital (IRB approval No. KUCH 2021-11-018) and individual consent for this retrospective analysis was waived.
Open Access Statement: This is an Open Access article distributed in accordance with the Creative Commons Attribution-NonCommercial-NoDerivs 4.0 International License (CC BY-NC-ND 4.0), which permits the non-commercial replication and distribution of the article with the strict proviso that no changes or edits are made and the original work is properly cited (including links to both the formal publication through the relevant DOI and the license). See: https://creativecommons.org/licenses/by-nc-nd/4.0/.
References
- Ghattas C, Alsunaid S, Pickering EM, et al. State of the art: percutaneous tracheostomy in the intensive care unit. J Thorac Dis 2021;13:5261-76. [Crossref] [PubMed]
- Griffiths J, Barber VS, Morgan L, et al. Systematic review and meta-analysis of studies of the timing of tracheostomy in adult patients undergoing artificial ventilation. BMJ 2005;330:1243. [Crossref] [PubMed]
- Freeman BD, Kennedy C, Robertson TE, et al. Tracheostomy protocol: experience with development and potential utility. Crit Care Med 2008;36:1742-8. [Crossref] [PubMed]
- Bouderka MA, Fakhir B, Bouaggad A, et al. Early tracheostomy versus prolonged endotracheal intubation in severe head injury. J Trauma 2004;57:251-4. [Crossref] [PubMed]
- Arabi Y, Haddad S, Shirawi N, et al. Early tracheostomy in intensive care trauma patients improves resource utilization: a cohort study and literature review. Crit Care 2004;8:R347-52. [Crossref] [PubMed]
- Kanna B, Ayman HA, Soni A. Early tracheostomy in intensive care trauma patient improves resource utilization: a cohort study and literature review. Crit Care 2005;9:414-6; author reply 414-6; discussion 414-6.
- Villemure-Poliquin N, Lessard Bonaventure P, Costerousse O, et al. Impact of Early Tracheostomy Versus Late or No Tracheostomy in Nonneurologically Injured Adult Patients: A Systematic Review and Meta-Analysis. Crit Care Med 2023;51:310-8. [Crossref] [PubMed]
- Ciaglia P, Firsching R, Syniec C. Elective percutaneous dilatational tracheostomy. A new simple bedside procedure; preliminary report. Chest 1985;87:715-9. [Crossref] [PubMed]
- Cheung NH, Napolitano LM. Tracheostomy: epidemiology, indications, timing, technique, and outcomes. Respir Care 2014;59:895-915; discussion 916-9. [Crossref] [PubMed]
- Durbin CG Jr. Techniques for performing tracheostomy. Respir Care 2005;50:488-96. [PubMed]
- Tai HP, Lee DL, Chen CF, et al. The effect of tracheostomy delay time on outcome of patients with prolonged mechanical ventilation: A STROBE-compliant retrospective cohort study. Medicine (Baltimore) 2019;98:e16939. [Crossref] [PubMed]
- Pauliny M, Christova E, Mackova J, et al. Percutaneous dilation tracheostomy versus surgical tracheostomy in critically ill patients. Bratisl Lek Listy 2012;113:409-11. [Crossref] [PubMed]
- Khaja M, Haider A, Alapati A, et al. Percutaneous Tracheostomy: A Bedside Procedure. Cureus 2022;14:e24083. [PubMed]
- Heikkinen M, Aarnio P, Hannukainen J. Percutaneous dilational tracheostomy or conventional surgical tracheostomy? Crit Care Med 2000;28:1399-402. [Crossref] [PubMed]
- Kaylie DM, Andersen PE, Wax MK. An analysis of time and staff utilization for open versus percutaneous tracheostomies. Otolaryngol Head Neck Surg 2003;128:109-14. [Crossref] [PubMed]
- Melloni G, Muttini S, Gallioli G, et al. Surgical tracheostomy versus percutaneous dilatational tracheostomy. A prospective-randomized study with long-term follow-up. J Cardiovasc Surg (Torino) 2002;43:113-21. [PubMed]
- Youssef TF, Ahmed MR, Saber A. Percutaneous dilatational versus conventional surgical tracheostomy in intensive care patients. N Am J Med Sci 2011;3:508-12. [Crossref] [PubMed]
- Ciaglia P. Technique, complications, and improvements in percutaneous dilatational tracheostomy. Chest 1999;115:1229-30. [Crossref] [PubMed]
- Griggs WM, Worthley LI, Gilligan JE, et al. A simple percutaneous tracheostomy technique. Surg Gynecol Obstet 1990;170:543-5. [PubMed]
- Fantoni A, Ripamonti D. A non-derivative, non-surgical tracheostomy: the translaryngeal method. Intensive Care Med 1997;23:386-92. [Crossref] [PubMed]
- Frova G, Quintel M. A new simple method for percutaneous tracheostomy: controlled rotating dilation. A preliminary report. Intensive Care Med 2002;28:299-303. [Crossref] [PubMed]
- Zgoda MA, Berger R. Balloon-facilitated percutaneous dilational tracheostomy tube placement: preliminary report of a novel technique. Chest 2005;128:3688-90. [Crossref] [PubMed]
- Cabrini L, Monti G, Landoni G, et al. Percutaneous tracheostomy, a systematic review. Acta Anaesthesiol Scand 2012;56:270-81. [Crossref] [PubMed]
- Byhahn C, Wilke HJ, Halbig S, et al. Percutaneous tracheostomy: ciaglia blue rhino versus the basic ciaglia technique of percutaneous dilational tracheostomy. Anesth Analg 2000;91:882-6. [Crossref] [PubMed]
- Van Heurn LW, Mastboom WB, Scheeren CI, et al. Comparative clinical trial of progressive dilatational and forceps dilatational tracheostomy. Intensive Care Med 2001;27:292-5. [Crossref] [PubMed]
- Cianchi G, Zagli G, Bonizzoli M, et al. Comparison between single-step and balloon dilatational tracheostomy in intensive care unit: a single-centre, randomized controlled study. Br J Anaesth 2010;104:728-32. [Crossref] [PubMed]
- Huang H, Li Y, Ariani F, et al. Timing of tracheostomy in critically ill patients: a meta-analysis. PLoS One 2014;9:e92981. [Crossref] [PubMed]
- Fikkers BG, Fransen GA, van der Hoeven JG, et al. Tracheostomy for long-term ventilated patients: a postal survey of ICU practice in The Netherlands. Intensive Care Med 2003;29:1390-3. [Crossref] [PubMed]
- Krishnan K, Elliot SC, Mallick A. The current practice of tracheostomy in the United Kingdom: a postal survey. Anaesthesia 2005;60:360-4. [Crossref] [PubMed]
- Delaney A, Bagshaw SM, Nalos M. Percutaneous dilatational tracheostomy versus surgical tracheostomy in critically ill patients: a systematic review and meta-analysis. Crit Care 2006;10:R55. [Crossref] [PubMed]
- Dempsey GA, Grant CA, Jones TM. Percutaneous tracheostomy: a 6 yr prospective evaluation of the single tapered dilator technique. Br J Anaesth 2010;105:782-8. [Crossref] [PubMed]