Size-matching in lung transplantation by computed tomography for end-stage interstitial disease: a retrospective analysis
Highlight box
Key findings
• We observed a strong correlation between computed tomography (CT) total lung capacity (TLC) (ctTLC) and measured TLC (mTLC) (Pearson r=0.762 with P<0.001) with the following regression equation: ctTLC (mL) = 0.6761 × mTLC (mL) + 460.
What is known and what is new?
• Size matching between donors and recipients is a major issue in lung transplantation, especially in patients with restrictive lung disease (RLD).
• CT-derived lung volumes correlate with TLC better than predicted TLC in patients with RLD.
What is the implication and what should change now?
• CT determination of TLC and respective volumes of the right and left lung is feasible in patients with end-staged interstitial lung disease awaiting transplantation and may be an interesting lead to choose the size of lung grafts.
Introduction
Lung transplantation (LTx) is a life-saving option for selected patients with end-stage interstitial lung disease (ILD), but the choice of adequate grafts has long been debated. According to the recommendations from the International Society of Heart and Lung Transplantation (ISHLT), the very rapid evolution of certain clinical forms has led teams to list patients more quickly (1). The size mismatch between donor and recipient total lung capacity (TLC) is a poor prognostic factor of lung function and survival after LTx, and a frequent restriction to graft acceptation (2,3).
In this setting, the definition of the appropriate graft size and the optimal method for defining it remains controversial. Chest diameter, chest X-ray, anthropometry, weight, and other factors have been used to predict lung volume (4,5). Predicted TLC (pTLC) using size and sex as independent variables, and measured TLC (mTLC) using plethysmography are used by most teams to match lung sizes (6,7). The limitations of these tools are well known and perioperative complications related to size mismatch remain and directly impact survival after LTx (8,9).
The recent development of computed tomography (CT) and image processing technologies makes it possible to accurately measure the volume of each lung (10-12). Some teams are already using this value as a reference for choosing the size of lung grafts (13), but no study has been specifically dedicated to ILD. The purpose of this work is to study the feasibility of using CT TLC (ctTLC) and to determine the correlation between ctTLC, mTLC, and pTLC in patients with end-stage ILD awaiting LTx. We present this article in accordance with the STROBE reporting checklist (available at https://jtd.amegroups.com/article/view/10.21037/jtd-23-1586/rc).
Methods
Study design
Medical records from patients who underwent LTx in Bichat University Hospital, Paris, France, were prospectively included in a local database. Characteristics of patients who underwent a first LTx for ILD between January 2014 and July 2018 were then retrospectively analyzed. ILD patients were defined by pre-transplantation pulmonary function test (PFT) showing a restrictive physiology, based on either an mTLC <80% predicted or a forced expiratory volume in 1 second (FEV1)/forced vital capacity (FVC) ratio >0.85 with a FEV1/FVC ratio >0.85 with a FVC <80% predicted (in the absence of TLC measurement) (14). Volumes of right and left lungs were calculated on the latest pre-transplantation CT scan to obtain ctTLC, and compare it to the latest pre-transplantation mTLC and pTLC. All patients signed an informed consent to authorize prospective data collection and retrospective data analysis. The database was declared to the French Commission for Informatics and Freedom [Commission National Informatique et Liberté (CNIL)] (authorization 1866407v0). The study of patients’ outcome was approved by the Regional Committee for Person Protection [Comité de Protection des Personnes-Ile de Franc, (CPP-IDF2)] (EudraCT/IRB 2017-A01714-49). The study was conducted in accordance with the Declaration of Helsinki (as revised in 2013). Primary endpoint is the correlation between ctTLC, mTLC, and pTLC in patients with end-stage ILD awaiting LTx.
PFTs
The PFTs were performed at the time of listing. TLC was measured by plethysmography, using a 2003 Jaeger® Masterscreen™ Body Diffusion device (The Surgical Company, Amsterdam, The Netherlands). FEV1, functional residual capacity and FVC were measured by spirometry using a 2015 Jaeger® Vyntus™ Master device (The Surgical Company). PFT lung volumes for each lung was calculated by using perfusion scintigraphy: in patients without pulmonary embolism, it is a reliable exam to assess each lung volume as it is recommended also to predict post-operative lung function in lung cancer patients undergoing pneumonectomy (15). Calculation of pTLC was carried out using the European Respiratory Society formulas defined in 1995 (5): for men: pTLC (mL) = (7.99 × H − 7.08) × 1,000, and for women: pTLC (mL) = (6.60 × H − 5.79) × 1,000, with H = height (m). The statistical analysis was done in 2020, before the publication by the ERS of the new global lung initiative TLC equations in 2021 (16). Measured and predicted right and left lung volumes [measured right lung volume (mRLV), predicted RLV (pRLV), measured left lung volume (mLLV), and predicted LLV (pLLV), respectively] were calculated from the TLC weighted by the results of lung perfusion scan.
CT volume analysis
CTs were performed on Aquilion ONE GENESIS 320 rows CT, Canon Medical system, Otawara, Japan. Parenchymal slides from the last CT before LTx were analyzed with CT Lung Density Analysis software (Vitrea Advanced Visualization software, Vital Images, Minnetonka, MN, USA). Lung volumes were calculated after semi-automatic contouring of the parenchyma. Interval chosen between each cut was 5 mm. Several measurements were performed by including and then excluding unventilated sub-pleural fibrosis areas. The surfaces were manually adjusted in the frontal and sagittal planes. Average densities and total volume of the parenchyma thus determined were calculated by the software (Figure S1).
Statistical analysis
Continuous variables with normal distribution were reported as mean and standard deviation, and compared using Student t-test. Continuous variables with non-normal distribution were reported as mean, median and interquartile range (IQR) and compared using Mann-Whitney test. Categorical variables were reported as count and proportion, and compared using Fisher or chi-squared tests when appropriate. Overall survival was defined as the time interval between the date of operation and the date of death or the last follow-up visit for censored patients. Follow-up information was obtained from the hospital case records. Median time of follow-up for the study group was 458 days (IQR, 199–948 days). Actuarial survival curves were estimated by the Kaplan-Meier method. Correlations were performed using Pearson and Spearman determination of the r coefficient in normal and non-normal variables, respectively. All data analyses were conducted with two-sided test. A P value <0.05 was considered significant. Statistical analyses were conducted using the Prism software (GraphPad Prism version 7.00, La Jolla, CA, USA).
Results
Study group
A total of 89 patients (male:female =68:21; mean age, 59.5±10.0 years) were included in the study. Demographic characteristics are summarized in Table 1. Histological diagnoses were grouped into four main groups following multidisciplinary discussion and pathological examination of native lungs. Idiopathic pulmonary fibrosis (IPF) was the most common diagnosis found with 55% (n=49) of cases. Among the nine cases with “other” diagnosis, there were three sarcoidosis, three unclassifiable fibrosis, two non-specific interstitial pneumonia, and one desquamative interstitial pneumonia. None of the patients had a history of major lung resection. Double LTx was performed in 46 patients (51.7%), and high emergency LTx in 11 patients (12.4%). Of the 43 patients who received a single LTx, 31 were transplanted on the right side (72.1%). After a mean follow-up of 583±483 days, 3-year survival was 61% (Figure S2).
Table 1
Demographic parameters | Values |
---|---|
Male | 68 (76.4) |
Age (years) | 59.5±10.0 |
Height (cm) | 171±8 |
Weight (kg) | 75±15 |
BMI (kg/m2) | 25.7±4.76 |
Diagnosis | |
IPF | 49 (55.1) |
Connective tissue disease-associated | 18 (20.2) |
Hypersensitivity pneumonitis | 7 (7.9) |
Pneumoconiosis | 6 (6.7) |
Other | 9 (10.1) |
Surgical history | 17 (19.1) |
Lung biopsy | 12 (13.5) |
Tube thoracostomy/pneumothorax | 2 (2.2) |
Pleurectomy/pneumothorax | 1 (1.1) |
Talc pleurodesis/pneumothorax | 1 (1.1) |
Childhood sarcoma metastasectomy | 1 (1.1) |
High emergency LTx | 16 (18.0) |
Single LTx | 43 (48.3) |
Values are presented as n (%) or mean ± SD. BMI, body mass index; IPF, idiopathic pulmonary fibrosis; LTx, lung transplantation; SD, standard deviation.
PFT
Plethismographic and spirometric data are summarized in Table 2, with a mean mTLC value of 3,530±1,077 mL. Perfusion scan found a mean perfusion of 52%±17% on the right side. The average time between the PFT and CT and between PFT and transplantation was respectively 162±270 days (median, 67 days; IQR, 0–233 days) and 295±286 days (median, 218 days; IQR, 127–377 days).
Table 2
PFT parameters | Values |
---|---|
FEV1 (mL) | 1,687±600 |
FEV1 (%) | 54±17 |
FVC (mL) | 1,991±780 |
FVC (%) | 52±18 |
VC (mL) | 2,088±837 |
VC (%) | 52±18 |
RV (mL) | 1,414±485 |
RV (%) | 66±22 |
FRC (mL) | 2,132±681 |
FRC (%) | 65±17 |
TLC (mL) | 3,530±1,077 |
pTLC (mL) | 6,381±955 |
TLC (%) | 75 |
Values are presented as mean ± SD or %. PFT, pulmonary function test; FEV1, forced expiratory volume in 1 second; FVC, forced vital capacity; VC, vital capacity; RV, residual volume; FRC, functional residual capacity; TLC, total lung capacity; pTLC, predicted total lung capacity; SD, standard deviation.
CT measurements
The data from the CT analysis are summarized in Table 3. The ctTLC could be measured in all patients, with an average of 2,880±989 mL. The average difference between total and ventilated volumes on the scanner was 329±323 mL. CT volumes of the right lung exhibited a normal distribution (D’Agostino and Pearson normality test, P=0.16) while CT volumes of the left lung did not (P=0.005). Statistical analysis revealed a significant difference between CT volume of the right and left lung (P<0.001, Figure 1). There was no significant difference between the average right and left densities [−556±111 vs. −553±126 Hounsfield unit (HU)].
Table 3
Parameters | Values |
---|---|
Lung volume (mL) | |
Right lung | |
Ventilated volume | 1,363±593 |
Total volume | 1,540±516 |
Left lung | |
Ventilated volume of left lung | 1,188±592 |
Total volume of left lung | 1,339±554/ 1,291 [957–1,609] |
ctTLC | 2,880±989 |
Lung density (HU) | |
Right lung | |
Ventilated mean density | −601±86 |
Ventilated standard deviation of density | −315±48 |
Whole mean density | −556±111 |
Whole standard deviation of density | −336±48 |
Left lung | |
Mean density of ventilated left lung | −593±107 |
Ventilated standard deviation of density | −309±46 |
Mean density of whole left lung | −553±126 |
Whole standard deviation of density | −329±48 |
Values are presented as mean ± SD or median [interquartile range]. CT, computed tomography; ctTLC, computed tomography total lung capacity; HU, Hounsfield unit; SD, standard deviation.
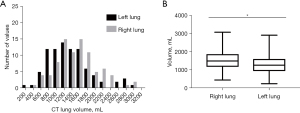
Comparison of pTLC, mTLC, and ctTLC
mTLC and ctTLC exhibited a normal distribution (P=0.23, P=0.054, respectively), while pTLC did not (P=0.02). CtTLC was significantly lower than mTLC and pTLC in three-variable comparison (Friedman test for paired measures, P<0.001) and in two-variable comparisons (Wilcoxon matched-pairs signed rank test, P<0.001 for all comparisons, Figure 2).
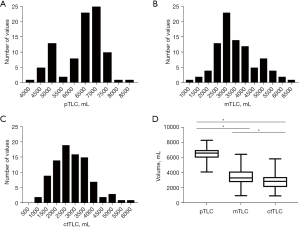
Assessment of right and left lung volume
We analyzed the respective volumes of the right and left lung according to the three modalities (Figure 3, Table 4). There was a more important difference between predicted lung volume, measured lung volume (mLV), and CT lung volume (ctLV) on the left side than on the right side.
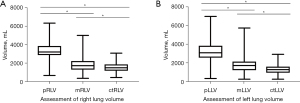
Table 4
Lung volumes | Right lung | Left lung |
---|---|---|
mLV vs. pLV | ||
Median (mL) | −1,432 | −1,388 |
P value | <0.001 | <0.001 |
ctLV vs. pLV | ||
Median (mL) | −1,664 | −1,899 |
P value | <0.001 | <0.001 |
ctLV vs. mLV | ||
Median (mL) | −260 | −351 |
Mean ± SD (mL) | −301±653 | −457±767 |
95% CI | −439 to −164 | −619 to −296 |
Relative difference (%) | 16.3 | 25.4 |
P value | <0.001 | <0.001 |
Relative difference = (mean ctLV − mean mLV)/mean mLV. mLV, measured lung volume; pLV, predicted lung volume; ctLV, computed tomography lung volume; SD, standard deviation; CI, confidence interval.
Correlations between pTLC, mTLC, and ctTLC
Results of correlation and linear regression analyses are presented in Figure 4. We first analyzed raw data and found a weak correlation between all continuous variables (ctTLC vs. mTLC: Pearson r=0.466 with P<0.001; ctRLV vs. mRLV: Spearman r=0.483 with P<0.001; ctLLV vs. mLLV: Spearman r=0.499 with P<0.001) (Figure 4A). After exclusion of two outliers on CT measurements (one bilateral pneumothorax listed in high emergency, one emphysema of upper lobes as histological comorbidity), we observed a strong correlation between ctTLC and mTLC (Pearson r=0.762 with P<0.001) with the following regression equation: ctTLC (mL) = 0.6761 mTLC (mL) + 460 (Figure 4B). The agreement between the two measurements are provided in Figure S3.
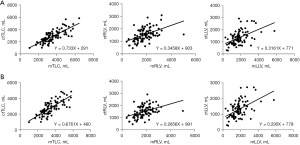
Discussion
Main result reminder
In our study, we found a significant difference between each of the three modalities studied for assessing TLC. Thus, average ctTLC was lower than the mTLC and much lower than the pTLC. Significant differences were also observed according to pulmonary side: the left lung CT volume was significantly lower than that of the right lung. Differences between the volumes measured on CT and those measured on PFT was greater on the left side. Analysis of the correlations between the different TLC measures revealed a strong correlation between mTLC and ctTLC. This confirms the findings of a previous study which showed that CT-derived lung volumes correlate with TLC better than pTLC in patients with restrictive lung disease (RLD) (17). It could be a potential surrogate marker for size matching in LTx.
The impact of graft sizing
Size of grafts in single or double LTx is decisive for both short-term and long-term outcomes (2). When implanted organ is oversized, surgical difficulties encountered may result in need of achieving a lung-volume reduction with the risk of prolonged air leaks, pulmonary atelectasis, pneumopathy, hemodynamic failures at chest closure, and even decreased survival (9). On the other hand, undersized grafts expose to risks of empyema, acute respiratory distress syndrome with weaning difficulties from mechanical ventilation and primary graft dysfunction (PGD), especially after single LTx (3). In addition to more complicated postoperative outcomes, size-mismatch has a negative impact on long-term survival. If all series report an excess mortality in case of undersizing, effects of oversizing are more discussed. Eberlein et al. described a beneficial effect of oversizing that reduces complications such as PGD (18). However, these results are obtained from series of patients with heterogeneous pathologies [chronic obstructive pulmonary disease (COPD), emphysema, fibrosis, etc.] and procedures (single and double lung transplants): oversizing is limited by the capacity of rib cage to contain the grafts and the necessity to close the chest at the end of surgery. In restricted pathologies, Riddell et al. found an overall survival and a chronic lung allograft dysfunction (CLAD)-free survival significantly improved following a size-matched double LTx, compared to those with donor-recipient (D-R) pTLC ratio <0.8 or >1.2. But for single LTx, size-matching based on a D-R pTLC ratio >0.8 and <1.0 did not significantly improve survival (19): the authors report non-significant trends towards improvement in grade 3 PGD and post-LTx survival.
Definition of graft sizing
In most teams, pTLC is used to adapt the size of the graft. Calculation of this value is based on equations whose only variables are sex and height. Adapted for healthy European individuals, this tool is much less suitable for predicting lung volumes in patients with fibrosis, and does not take into account neither weight incidence, thoracic surgical history nor ethnic differences (8). In their literature review in 2013, Barnard et al. (2) established new size matching recommendations for the ISHLT: in the absence so far of reliable guidelines for assessing recipient’s actual TLC, the authors suggest that it is acceptable to select a transplant from a donor whose pTLC is within 15% to 20% of the mean of the recipient’s pTLC and mTLC. Loizzi et al. also recommend using this ratio to choose between whole LTx and lobar transplantation (20), despite poor outcomes for lobar LTx. Although chest diameter, chest X-ray, anthropometry, weight and other factors have been used in the past to predict lung volume (4,5), LTx teams are still looking for a new and more accurate indicator of recipients’ actual chest cavity volume (21). Delayed chest closure cannot be considered a safe option when graft oversizing is predictable (22).
CT scan for graft sizing
The difficulty of graft sizing urged the need for new tools to better evaluate lung volumes in transplantation. Among them, volumetric measures by CT scan is a reliable way to evaluate the volumes of each lung under forced inspiration and thus obtain an approximation of the TLC (10,12,23,24). This technique has already been applied in the particular setting of living donor LTx. Thus, Kojima et al. and Chen-Yoshikawa et al. described the use of 3D CT scan reconstructions for the elaboration of surgical strategy and sizing matching in this specific indication (13,25). However, these interesting results cannot be generalized to regular bilateral or single LTx techniques. Benefits from CT scan has already been highlighted for deceased donors, but in a very heterogeneous and non-Western recipient population (17,26). Since all transplant recipients have at least one CT scan in their pre-operative assessment and all potential donors are evaluated with a CT scan, this may allow a better fitting than plethysmography or anthropometry (27). This benefit may be particularly true for single LTx during which it may allow an accurate measurement of lung volume on the transplanted side (28). This can provide a better match than an overall analysis of both lungs and the patient’s respiratory function tests (29,30). In our experience of single LTx for pulmonary fibrosis, main intra-operative difficulties are related to oversizing of the pulmonary graft on the left side. Our study confirms this clinical feeling: measured size of the rib cage in recipients with ILD is much smaller than the mTLC and pTLC values that are currently used as calibration for graft selection, especially on the left side. The significant correlation between ctTLC and mTLC is another element showing that CT volume corresponds to a functional reality and should make this variable an essential tool for graft selection: similar correlation has been highlighted by Hussain et al. in ILD (31). Jung et al. found that ctLV showed a better correlation with mTLC compared to pTLC in patients with obstructive disease but a similar correlation with mTLC compared to pTLC in patients with restrictive pulmonary disease: the number of patients included in the restrictive group were limited (n=37) compared to the obstructive group (n=110), which might explain the absence of a significant difference in the restrictive group (17).
Study limitations
Our study has technical and methodological limitations. From the technical standpoint, determination of mTLC and ctTLC might be difficult to obtain in patients with end-staged lung disease. In this setting, the apnea required for CT scanning is short and might be easier to obtain than the quiet breath with obstructed mouth required for plethysmography. Moreover, the volumes measured by CT volumetry and plethysmography may differ because of the way CT acquisition was made. In our hospital, patients are breath-hold-coached by a standard voice recording during CT acquisition, but it has been shown in a study in severe chronic obstructive pneumopathy disease patients with emphysema that spirometry gating in CT helps to improve agreement with body plethysmography results (32). Indeed, in the study by Bakker et al., the mean ± SD CT-derived TLC was 280±340 mL lower compared to body plethysmography in the gated group (n=100), and 590±430 mL lower for the non-gated group (n=100) (both P<0.001) (32). Such difference needs to be confirmed in restrictive patients.
The other technical difficulty encountered in our study was in patients transplanted in high emergency with bilateral pneumothoraces and subcutaneous emphysema, requiring manual contouring of the rib cage. From the methodological point of view, interpretation of the results must take into account time between completion of PFTs, CT scan, and transplantation; rapid deterioration of lung function in some of these patients may have caused confusion bias. However, this reflects everyday practice, where LTx candidates undergo PFTs at the time of listing and CT scan during a median waiting time of 7 months. The results from this study could benefit a prospective trial evaluating impact of size matching by D-R ctTLC ratio on postoperative outcomes. Indeed, by improving size matching with CT volume, we may better anticipate short and long-term outcomes. Prabhu et al. have demonstrated, by comparing pTLC ratio (calculated by dividing the donor pTLC by the recipient pTLC) with volumetric ratio (calculated by dividing the donor pTLC by the recipient ctTLC), that undersizing was independently associated with significantly lower odds of PGD unlike the pTLC ratio (33): this was confirmerd by Vazquez Guillamet et al. who found that larger donor and smaller recipient lung volumes estimated by CT predicted a need for surgical graft reduction and were associated with higher PGD grade (34). Concerning long-term outcomes, using the volumetric ratio, oversizing was independently associated with a higher risk of death unlike the pTLC ratio (33).
Conclusions
CT determination of TLC and respective volumes of the right and left lung is feasible in patients with end-staged ILD awaiting transplantation. The obtained ctTLC is much lower than mTLC and pTLC, with ctTLC being significantly correlated to mTLC. Every donor in France has a Thorax CT before the organs are proposed to the transplantation team. Using thorax CT from both donors and recipient to assess size-matching instead of relying only on pTLC from donor and both pTLC and mTLC from recipients might reduce both oversizing and undersizing and therefore have an impact on outcomes. This study highlights potential size mismatch for graft selection before LTx, in ILD and opens the perspective of a prospective trial evaluating impact of size matching by D-R ctTLC ratio on postoperative outcomes.
Acknowledgments
The authors would like to thank the members of the Bichat Lung Transplant Group (nurses: Dahbia Benhammada, Elena Peiracchia, Sabrina Trigueiros, Lucie Genet; respiratory therapists: Cindy Chevalier, Nicolas Doumecq-Lacoste; dietician: Agnès Abadie; psychologist: Alice Savary; rehabilitation: Linda Hajouji-Idrissi, Valeria Velea; intensive care unit: Enora Atchade, Sebastien Tanaka, Aurélie Snauwert, Parvine Tashk, Sylvain Jean-Baptiste, Brice Lortat-Jacob; anesthesiology: Sandrine Boudinet, Nils Carrara, Aurélie Gouel, Adela Harpan, Mouna Ben Rehouma, Elie Kantor, Fabian Padin; pulmonology: Tiphaine Goletto, Gaëlle Weisenburger, Vincent Bunel, Gilles Jebrak, Armelle Marceau, Adrian Crutu, Domitille Mouren; surgery: Antoine Girault; data managers: Miassa Berkal, Isabelle Kouidri).
Funding: None.
Footnote
Reporting Checklist: The authors have completed the STROBE reporting checklist. Available at https://jtd.amegroups.com/article/view/10.21037/jtd-23-1586/rc
Data Sharing Statement: Available at https://jtd.amegroups.com/article/view/10.21037/jtd-23-1586/dss
Peer Review File: Available at https://jtd.amegroups.com/article/view/10.21037/jtd-23-1586/prf
Conflicts of Interest: All authors have completed the ICMJE uniform disclosure form (available at https://jtd.amegroups.com/article/view/10.21037/jtd-23-1586/coif). The authors have no conflicts of interest to declare.
Ethical Statement: The authors are accountable for all aspects of the work in ensuring that questions related to the accuracy or integrity of any part of the work are appropriately investigated and resolved. All patients signed an informed consent to authorize prospective data collection and retrospective data analysis. The database was declared to the French Commission for Informatics and Freedom (Commission National Informatique et Liberté, CNIL, authorization 1866407v0). The study of patients’ outcome was approved by the Regional Committee for Person Protection (Comité de Protection des Personnes - Ile de France, CPP-IDF2, EudraCT/IRB 2017-A01714-49). The study was conducted in accordance with the Declaration of Helsinki (as revised in 2013).
Open Access Statement: This is an Open Access article distributed in accordance with the Creative Commons Attribution-NonCommercial-NoDerivs 4.0 International License (CC BY-NC-ND 4.0), which permits the non-commercial replication and distribution of the article with the strict proviso that no changes or edits are made and the original work is properly cited (including links to both the formal publication through the relevant DOI and the license). See: https://creativecommons.org/licenses/by-nc-nd/4.0/.
References
- Weill D. Lung transplantation: indications and contraindications. J Thorac Dis 2018;10:4574-87. [Crossref] [PubMed]
- Barnard JB, Davies O, Curry P, et al. Size matching in lung transplantation: an evidence-based review. J Heart Lung Transplant 2013;32:849-60. [Crossref] [PubMed]
- Ganapathi AM, Mulvihill MS, Englum BR, et al. Transplant size mismatch in restrictive lung disease. Transpl Int 2017;30:378-87. [Crossref] [PubMed]
- Massard G, Badier M, Guillot C, et al. Lung size matching for double lung transplantation based on the submammary thoracic perimeter. Accuracy and functional results. The Joint Marseille-Montreal Lung Transplant Program. J Thorac Cardiovasc Surg 1993;105:9-14. [Crossref] [PubMed]
- Stocks J, Quanjer PH. Reference values for residual volume, functional residual capacity and total lung capacity. ATS Workshop on Lung Volume Measurements. Official Statement of The European Respiratory Society. Eur Respir J 1995;8:492-506. [Crossref] [PubMed]
- Orens JB, Estenne M, Arcasoy S, et al. International guidelines for the selection of lung transplant candidates: 2006 update--a consensus report from the Pulmonary Scientific Council of the International Society for Heart and Lung Transplantation. J Heart Lung Transplant 2006;25:745-55. [Crossref] [PubMed]
- Konheim JA, Kon ZN, Pasrija C, et al. Predictive equations for lung volumes from computed tomography for size matching in pulmonary transplantation. J Thorac Cardiovasc Surg 2016;151:1163-9.e1. [Crossref] [PubMed]
- Harik-Khan RI, Fleg JL, Muller DC, et al. The effect of anthropometric and socioeconomic factors on the racial difference in lung function. Am J Respir Crit Care Med 2001;164:1647-54. [Crossref] [PubMed]
- Soresi S, Sabashnikov A, Weymann A, et al. When the Battle is Lost and Won: Delayed Chest Closure After Bilateral Lung Transplantation. Med Sci Monit Basic Res 2015;21:222-5. [Crossref] [PubMed]
- Wanger J, Clausen JL, Coates A, et al. Standardisation of the measurement of lung volumes. Eur Respir J 2005;26:511-22. [Crossref] [PubMed]
- O'Donnell CR, Bankier AA, Stiebellehner L, et al. Comparison of plethysmographic and helium dilution lung volumes: which is best for COPD? Chest 2010;137:1108-15. [Crossref] [PubMed]
- Matsumoto AJ, Bartholmai BJ, Wylam ME. Comparison of Total Lung Capacity Determined by Plethysmography With Computed Tomographic Segmentation Using CALIPER. J Thorac Imaging 2017;32:101-6. [Crossref] [PubMed]
- Kojima K, Kato K, Oto T, et al. Preoperative graft volume assessment with 3D-CT volumetry in living-donor lobar lung transplantations. Acta Med Okayama 2011;65:265-8. [PubMed]
- Pellegrino R, Viegi G, Brusasco V, et al. Interpretative strategies for lung function tests. Eur Respir J 2005;26:948-68. [Crossref] [PubMed]
- Brunelli A, Charloux A, Bolliger CT, et al. ERS/ESTS clinical guidelines on fitness for radical therapy in lung cancer patients (surgery and chemo-radiotherapy). Eur Respir J 2009;34:17-41. [Crossref] [PubMed]
- Hall GL, Filipow N, Ruppel G, et al. Official ERS technical standard: Global Lung Function Initiative reference values for static lung volumes in individuals of European ancestry. Eur Respir J 2021;57:2000289. [Crossref] [PubMed]
- Jung WS, Haam S, Shin JM, et al. The feasibility of CT lung volume as a surrogate marker of donor-recipient size matching in lung transplantation. Medicine (Baltimore) 2016;95:e3957. [Crossref] [PubMed]
- Eberlein M, Reed RM, Bolukbas S, et al. Lung size mismatch and primary graft dysfunction after bilateral lung transplantation. J Heart Lung Transplant 2015;34:233-40. [Crossref] [PubMed]
- Riddell P, Ma J, Dunne B, et al. A simplified strategy for donor-recipient size-matching in lung transplant for interstitial lung disease. J Heart Lung Transplant 2021;40:1422-30. [Crossref] [PubMed]
- Loizzi D, Aigner C, Jaksch P, et al. A scale for decision making between whole lung transplantation or lobar transplantation. Eur J Cardiothorac Surg 2010;37:1122-5. [Crossref] [PubMed]
- Eberlein M, Reed RM. Donor to recipient sizing in thoracic organ transplantation. World J Transplant 2016;6:155-64. [Crossref] [PubMed]
- Chen C, Zheng Q, Wu D, et al. Review of outcomes of delayed chest closure following lung transplantation: a meta-analysis. J Cardiothorac Surg 2022;17:122. [Crossref] [PubMed]
- Tantucci C, Bottone D, Borghesi A, et al. Methods for Measuring Lung Volumes: Is There a Better One? Respiration 2016;91:273-80. [Crossref] [PubMed]
- Shin JM, Kim TH, Haam S, et al. The repeatability of computed tomography lung volume measurements: Comparisons in healthy subjects, patients with obstructive lung disease, and patients with restrictive lung disease. PLoS One 2017;12:e0182849. [Crossref] [PubMed]
- Chen-Yoshikawa TF, Date H. Three-dimensional image in lung transplantation. Gen Thorac Cardiovasc Surg 2018;66:19-26. [Crossref] [PubMed]
- Hwang SH, Lee JG, Kim TH, et al. Comparison of Predicted Total Lung Capacity and Total Lung Capacity by Computed Tomography in Lung Transplantation Candidates. Yonsei Med J 2016;57:963-7. [Crossref] [PubMed]
- Iwano S, Okada T, Satake H, et al. 3D-CT volumetry of the lung using multidetector row CT: comparison with pulmonary function tests. Acad Radiol 2009;16:250-6. [Crossref] [PubMed]
- Park SJ, Houck J, Pifarre R, et al. Optimal size matching in single lung transplantation. J Heart Lung Transplant 1995;14:671-5. [PubMed]
- Raghu G, Collard HR, Egan JJ, et al. An official ATS/ERS/JRS/ALAT statement: idiopathic pulmonary fibrosis: evidence-based guidelines for diagnosis and management. Am J Respir Crit Care Med 2011;183:788-824. [Crossref] [PubMed]
- Hansell DM, Goldin JG, King TE Jr, et al. CT staging and monitoring of fibrotic interstitial lung diseases in clinical practice and treatment trials: a position paper from the Fleischner Society. Lancet Respir Med 2015;3:483-96. [Crossref] [PubMed]
- Hussain M, Thornton M, Hussain T, et al. Evaluating the Use of CT-Derived Lung Volumes in Donor-Recipient Lung Size Matching for Lung Transplantation in Patients With Interstitial Lung Disease and/or Idiopathic Pulmonary Fibrosis. Transplant Proc 2023;55:623-8. [Crossref] [PubMed]
- Bakker JT, Klooster K, Bouwman J, et al. Evaluation of spirometry-gated computed tomography to measure lung volumes in emphysema patients. ERJ Open Res 2022;8:00492-2021. [Crossref] [PubMed]
- Prabhu NK, Wong MK, Klapper JA, et al. Computed Tomography Volumetrics for Size Matching in Lung Transplantation for Restrictive Disease. Ann Thorac Surg 2024;117:413-21. [Crossref] [PubMed]
- Vazquez Guillamet R, Rjob A, Bierhals A, et al. Potential Role of Computed Tomography Volumetry in Size Matching in Lung Transplantation. Transplant Proc 2023;55:432-9. [Crossref] [PubMed]