The role of 3D printing in chest wall reconstruction
Introduction
The use of three-dimensional (3D) technology and preoperative simulation constitute modern technological and methodological resources that are being increasingly incorporated into the routine surgical practice of chest wall surgeons. Surgical specialties such as cardiac, vascular, orthopaedical, and craniomaxillofacial surgery have already begun to implement these tools with vast clinical implications (1-7).
Standardization of surgical procedures might minimize or even eradicate intraoperative improvisation. However, planning and standardization of the repair of complex chest wall malformations is challenging given their inherent heterogeneity. 3D technology offers a unique alternative for simultaneous treatment standardization and customization.
In the last few years, there has been increasing interest on this subject, though with publications mostly involving case reports or small case series. In this review, we explore the current knowledge about the application of 3D technology for simple and complex chest wall reconstructions.
Simple chest wall reconstruction
Under this term, we refer to the use of 3D technology to aid in the resolution of simple chest wall deformities such as pectus excavatum (PE) and pectus carinatum (PC). Although these are far more prevalent than complex chest wall reconstructions, few studies have reported the implementation of 3D technology for the resolution of these malformations.
Regarding the minimally invasive repair of PE (MIRPE), although there have been many improvements to the original technique, decisions related to the number, location, and direction of implants are still made in the operating room with the patient under general anesthesia. This results in a time-consuming procedure that may lead, especially in the case of less experienced surgeons, to a wrong selection of the length and configuration of the implants, thus requiring extensive re-bending, removal, and repetitive flipping of the bars. Also, bending the implant during surgery prompts the creation of scratches and notches that have been related to bleeding complications during the procedure or at bar removal (Figure 1).
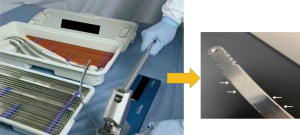
However, the aforementioned setbacks may be addressed using 3D technology. On one hand, the 3D virtual reconstruction from a computed tomography (CT) chest scan enables the surgical team to elaborate a detailed preoperative plan, including the number of implants required, their direction, and their entry points to the thoracic cavity (Figure 2). Computer programs have been developed to determine the precise length and shape of the prescribed implants and Standard Triangle Language (STL) files can be created for 3D printing of templates in materials such as polylactic acid that can be used for implant customization (Figure 3). On the other hand, 3D printing of real-size models of the chest wall or even the complete thoracic cage is being used for simulation, education, or tailored implant template manufacture as well.
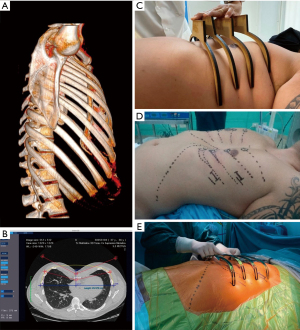
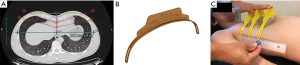
Using this approach, some authors have reported their initial experience in case reports or small case series. In 2018, Matsuo et al. published the 3D printing and reconstruction of the anterior chest wall of a PE patient, with preoperative simulation of the surgery, and further performing the procedure as planned (8). In 2022, Shan et al. described a larger cohort of 10 PE patients whose complete thoracic cages were 3D-printed for preoperative simulation and education of the patients and their families (9).
Other groups have extended the utility of this resource and used it not only for simulation but also for implant customization. Lai et al. reported in 2009 the use of CT versus the traditional method to determine implant length and the curvature of the malleable implant intraoperatively with increased accuracy and fewer errors than bending the bar intraoperatively (10). Lin et al. reported in 2018, 10 patients in whom they designed the implants virtually based on the CT 3D reconstruction, 3D printed the templates, and shaped the implants accordingly, through a process named 3DPMAN (11). In 2020 Wang et al. and Gaspar Perez et al. in 2021, they both reported 6 cases each performed with the same approach: 3D printing of the rib cage and the anterior aspect of the affected chest wall, respectively, and implant customization with optimal results (12,13).
However, the most extensive experiences were published by Vilaça et al. in 2014, Huang et al. in 2019, and Bellia-Munzon et al. in 2020 (14-16). Vilaça reported the creation of automatic pre-bent customized prostheses for patients undergoing MIRPE. They automatically manufactured and introduced 41 implants in 41 patients, and at the time of publication, 15 bars had been removed. In 2019, Huang performed 15 simulations of MIRPE in 3D printed real-size models and implant customization and compared them with 342 other MIRPE cases. They found that the 3D printing group had a shorter operative time (60 versus 74 minutes, P<0.001), fewer metallic bar placements (1.00 versus 1.36 bars, P<0.001), and better improvement percentages in the Haller indices (20.3% versus 10.1%, P<0.001) compared with the traditional Nuss procedure.
Finally, Bellia-Munzon et al. published the largest series including 130 patients systematically diagnosed and treated employing 3D technology in the process. This process consisted of the following:
- Preoperative planning using 3D CT scan reconstruction and printing of an implant template based on the CT data (Figure 4),
- An ambulatory fitting session with the custom 3D printed templates (Figure 5), and
- Manufacture of a final, custom-made, pre-bent metallic implant based on the template configuration (Figure 6).
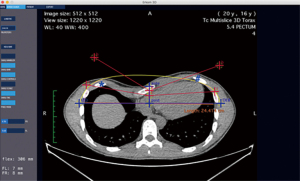
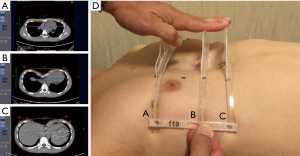
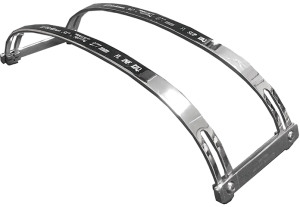
They found that 120 (92.3%) had an optimal “implant-deformity” anatomic match requiring no modification and minimal modifications in 10 cases, 7 minimal re-bending without bar flipping, 1 that required bar flipping and removal once, and 2 shorter than necessary. Also, they compared these cases with a historic cohort with the traditional approach and found a significant reduction in the operative time (87.6±49.9 vs. 125.4±30.7 minutes; P<0.0001) with a further decrease when adjusted to the number of bars per operation (41.8±14.7 vs. 78.1±31.7 minutes per implant; P<0.0001).
Regarding PC, the extensive use of braces has reduced the number of surgical candidates. However, in 2022 Martinez-Ferro et al. published the implementation of 3D technology in the minimally invasive repair of PC (17). They reported its use in the diagnosis with a 3D reconstruction of the chest CT, virtual implant design, template 3D printing and checking, and customized implant manufacture. They applied this process in 9 cases with no intraoperative complications.
Complex chest wall reconstruction
Complex chest wall deformities include the excavatum/carinatum complex, pectus arcuatum, and the Poland Syndrome. Other complex reconstructions comprise those after extensive oncological resections that involve three or more adjacent ribs or the sternum.
Reconstruction for complex deformities
Despite the increasing implementation and usefulness of 3D technology in chest wall reconstructions, publications on the resolution of complex deformities are relatively rare.
In 2019, Leng et al. reported using computer-aided designed (CAD), 3D printed cutting templates in four patients with pectus arcuatum with optimal results (18). The wedge sternotomies and cutting templates were planned and designed virtually using 3D reconstructions of CT scans and then 3D printed.
In 2021, Martinez et al. published seven cases with complex chest wall deformities approached with a process using 3D technology between 2015 and 2020 (19). Diagnosis included isolated Poland syndrome (n=1), pectus arcuatum (n=2), Poland syndrome associated with pectus arcuatum (n=3), and carinated deformity with complex sternal malformation (n=1).
The therapeutic process was comprised of the following steps:
- Preoperative planning.
Using 6 medical photographs and the results of chest CT and 3D reconstruction as well as optical surface scan, patients were discussed in a multidisciplinary setting (Figure 7). The surgical plan was elaborated, including approach, need for sternal osteotomies and remodeling, sternal plates, chondral resection, prosthetic ribs, or retrosternal implants (Figure 8). - CT post-processing and 3D printing, simulation, and implant check.
CT post-processing and 3D printing of real-size models of the chest wall or areas of interest, such as the sternum and affected ribs in polylactic acid, were done. Simulation included wedge osteotomies using customized cutting guides, evaluation of thoracic stability, and concordance between plates and implants was checked (Figure 9). If intrathoracic implants were necessary, templates were 3d printed and checked on the patient during a fitting session. - Implant manufacturing and surgery.
Once the plates and templates were approved, titanium customized implants were manufactured (Figure 10). Later, the surgery was duly performed according to a detailed, stepwise plan (Figure 11).
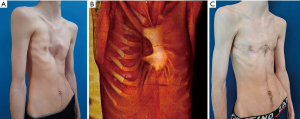
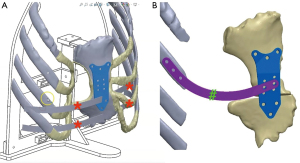
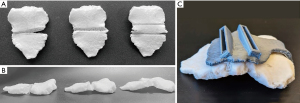
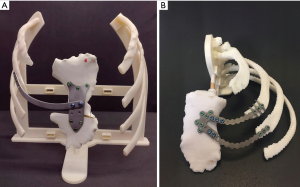
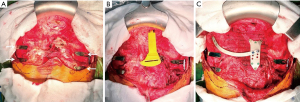
The authors reported complete agreement and no need to re-bend or modify the implants during surgery.
Finally, Chavoin et al. also reported the usefulness of 3D printing in chest wall deformities in 2022, in a study comprising 638 with PE and 151 with Poland syndrome (20). Assisted by a computer-aided design and manufacturing, they used customized silicone elastomer implants to reshape their chest walls.
Reconstruction after oncological chest wall resections
Unlike complex chest wall deformities, the use of 3D technology for reconstruction after wide oncological thoracic resections has been extensively published in the last ten years for surgical planning, simulation, prostheses design, 3D printing of implants, and also education (21-35). In general, the aims of reconstruction include defect coverage, adequate implant anchoring, and preservation of functionality (Figure 12), especially when lower chest wall resections are made and normal excursion may be impaired by rigid prosthesis. In this sense, several authors explore increasing implant flexibility by means of 3D printed prosthesis made of different materials.
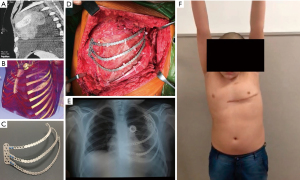
Among the main case series employing 3D technology, Wu et al. published in 2018, six cases with large chest wall tumors with a mean age of 43.2±23.8 years (28). They used CT scans to 3D print real-size models in which they simulated the resection and designed the prostheses. Subsequently, they manufactured conformal titanium plates, simulated the reconstruction, and posteriorly applied them in surgery. They reported good postoperative activity and respiratory movements. In 2019, Wang et al. reported for the first time sternal repair in 8 patients and rib reconstruction in 10 patients with large tumors using 3D printed polyetheretherketone (PEEK) prostheses (33). They fixated the PEEK implants with wire or titanium screws, on the inner surface a pericardial patch was densely suspended and sutured to the adjacent pleura, and a myofascial flap covered the outer surface. In 2020, Tan et al. treated 34 patients with sizeable chest wall tumors and randomly divided them into two groups: one with the conventional approach and the research group, approached with the inclusion of 3D technology for simulation and printing (23). The duration of surgery, the accuracy of surgical route prediction, and bleeding volume in the research group were significantly lower, although there was no difference in the length of stay. Lastly, Pontiki et al. published in 2022 their experience in ten patients who underwent large chest wall tumor resection (27). They 3D printed silicone molds of the planned resection areas and manufactured customized methyl methacrylate implants. Compared with a simultaneous cohort of patients repaired with non-rigid meshes, patients showed a trend towards better cosmetics, improved breathing mechanics, and a higher quality of life.
Future directions include increasing accessibility of 3D technology, lower costs, and the development of processes that reduce the time required to manufacture 3D models and implants. Also, evidence-based data is necessary to provide firm support in investment in new technologies that enhance optimal medical results.
Creation of a 3D model based on preoperative CT images
As an example of the application of 3D technology and printing in the repair of the chest wall, the following steps illustrate the creative process of PE implants in our clinical experience within an institution dedicated to chest malformations.
- Step 1: image acquisition: based on non-contrast chest CT scans at end-expiration (80 kV, slice thickness 2.0 mm, increment 1.0 mm) (36).
- Step 2: determination of the number of implants required depending on the patient’s age, presence of chondral calcifications, and Titanic Index (37). The direction and position of the implants are planned according to the definition of target areas necessary to treat in order to achieve total chest wall remodeling and whether the sternum is shaped as a banana or not (13).
- Step 3: prostheses design: using specially designed software (Erkom 3D Pro, Buenos Aires, Argentina), a semiautomatic process is performed to determine to precise length and shape of the implants. Next, STL files are created and the implants’ templates are 3D printed.
- Step 4: an ambulatory fitting session is organized to test the templates on the patient’s chest wall. If they fit correctly, metallic prebent implants are manufactured with steel o titanium depending on an allergy test.
- Step 5: the customized prebent mirror-polished sterile implants are delivered to the operation room for surgery.
Conclusions
3D technology has become increasingly available to the chest wall surgeon allowing for various practices that may enhance surgical planning through simulation in tailored, real-size phantoms, and the design and manufacture of customized implants. This approach might enable greater surgical precision and reduced improvisation, allowing for a better implant/deformity match, and potentially decreasing operative time. As this technology evolves and becomes available to a broader public, more chest wall teams should consider including them in their practices.
Acknowledgments
Funding: None.
Footnote
Provenance and Peer Review: This article was commissioned by the Guest Editors (Erik de Loos, José Ribas de Campos and Jean Daemen) for the series “Chest Wall Resections and Reconstructions” published in Journal of Thoracic Disease. The article has undergone external peer review.
Peer Review File: Available at https://jtd.amegroups.com/article/view/10.21037/jtd-23-881/prf
Conflicts of Interest: All authors have completed the ICMJE uniform disclosure form (available at https://jtd.amegroups.com/article/view/10.21037/jtd-23-881/coif). The special series “Chest Wall Resections and Reconstructions” was commissioned by the editorial office without any funding or sponsorship. M.M.F. reports ownership of Pampamed SRL. G.B.M. reports pending patents from Pampamed SRL. The authors have no other conflicts of interest to declare.
Ethical Statement: The authors are accountable for all aspects of the work in ensuring that questions related to the accuracy or integrity of any part of the work are appropriately investigated and resolved. All clinical procedures described in this study were conducted in accordance with the Declaration of Helsinki (as revised in 2013). As a narrative review, this manuscript does not require ethics committee approval. Informed consent was obtained from all individual participants whose images were used in this manuscript.
Open Access Statement: This is an Open Access article distributed in accordance with the Creative Commons Attribution-NonCommercial-NoDerivs 4.0 International License (CC BY-NC-ND 4.0), which permits the non-commercial replication and distribution of the article with the strict proviso that no changes or edits are made and the original work is properly cited (including links to both the formal publication through the relevant DOI and the license). See: https://creativecommons.org/licenses/by-nc-nd/4.0/.
References
- Khorsandi D, Fahimipour A, Abasian P, et al. 3D and 4D printing in dentistry and maxillofacial surgery: Printing techniques, materials, and applications. Acta Biomater 2021;122:26-49. [Crossref] [PubMed]
- Zoabi A, Redenski I, Oren D, et al. 3D Printing and Virtual Surgical Planning in Oral and Maxillofacial Surgery. J Clin Med 2022;11:2385. [Crossref] [PubMed]
- Rodriguez Colon R, Nayak VV, Parente PEL, et al. The presence of 3D printing in orthopedics: A clinical and material review. J Orthop Res 2023;41:601-13. [Crossref] [PubMed]
- Feng Y, Zhu S, Mei D, et al. Application of 3D Printing Technology in Bone Tissue Engineering: A Review. Curr Drug Deliv 2021;18:847-61. [Crossref] [PubMed]
- El Sabbagh A, Eleid MF, Al-Hijji M, et al. The Various Applications of 3D Printing in Cardiovascular Diseases. Curr Cardiol Rep 2018;20:47. [Crossref] [PubMed]
- Verghi E, Catanese V, Nenna A, et al. 3D Printing in Cardiovascular Disease: Current Applications and Future Perspectives. Surg Technol Int 2021;38:314-24. [Crossref] [PubMed]
- Ganguli A, Pagan-Diaz GJ, Grant L, et al. 3D printing for preoperative planning and surgical training: a review. Biomed Microdevices 2018;20:65. [Crossref] [PubMed]
- Matsuo N, Matsumoto K, Taura Y, et al. Initial experience with a 3D printed model for preoperative simulation of the Nuss procedure for pectus excavatum. J Thorac Dis 2018;10:E120-4. [Crossref] [PubMed]
- Shan Y, Yu G, Lu Y, et al. Application of three-dimensional reconstruction technology combined with three-dimensional printing in the treatment of pectus excavatum. Ann Thorac Med 2022;17:173-9. [Crossref] [PubMed]
- Lai JY, Wang CJ, Chang PY. The measurement and designation of the pectus bar by computed tomography. J Pediatr Surg 2009;44:2287-90. [Crossref] [PubMed]
- Lin KH, Huang YJ, Hsu HH, et al. The Role of Three-Dimensional Printing in the Nuss Procedure: Three-Dimensional Printed Model-Assisted Nuss Procedure. Ann Thorac Surg 2018;105:413-7. [Crossref] [PubMed]
- Wang L, Guo T, Zhang H, et al. Three-dimensional printing flexible models: a novel technique for Nuss procedure planning of pectus excavatum repair. Ann Transl Med 2020;8:110. [Crossref] [PubMed]
- Gaspar Pérez M, Núñez García B, Álvarez García N, et al. Initial experience with 3D printing in the use of customized Nuss bars in pectus excavatum surgery. Cir Pediatr 2021;34:186-90. [PubMed]
- Bellia-Munzon G, Martinez J, Toselli L, et al. From bench to bedside: 3D reconstruction and printing as a valuable tool for the chest wall surgeon. J Pediatr Surg 2020;55:2703-9. [Crossref] [PubMed]
- Vilaça JL, Rodrigues PL, Soares TR, et al. Automatic prebent customized prosthesis for pectus excavatum minimally invasive surgery correction. Surg Innov 2014;21:290-6. [Crossref] [PubMed]
- Huang YJ, Lin KH, Chen YY, et al. Feasibility and Clinical Effectiveness of Three-Dimensional Printed Model-Assisted Nuss Procedure. Ann Thorac Surg 2019;107:1089-96. [Crossref] [PubMed]
- Martinez-ferro M. Minimally Invasive Pectus Carinatum Repair. Second Ed. Holcomb III GW, Rothenberg SS, editors. Atlas of Pediatric Laparoscopy and Thoracoscopy. Elsevier Inc.; 2022. 1-6 p.
- Leng S, Bici K, Facchini F, et al. Customized Cutting Template to Assist Sternotomy in Pectus Arcuatum. Ann Thorac Surg 2019;107:1253-8. [Crossref] [PubMed]
- Martinez J, Toselli L, Aleixandre CG, et al. Surgical Planning, Simulation, and Prosthesis Customization for Complex Chest Wall Malformations. Ann Thorac Surg 2021;112:e295-8. [Crossref] [PubMed]
- Chavoin JP, Facchini F, Martinot-Duquennoy V, et al. Congenital thoracic deformities and 3D custom-made implants. New classification based on a series of 789 treated cases. Ann Chir Plast Esthet 2022;67:393-403. [Crossref] [PubMed]
- Aranda JL, Jiménez MF, Rodríguez M, et al. Tridimensional titanium-printed custom-made prosthesis for sternocostal reconstruction. Eur J Cardiothorac Surg 2015;48:e92-4. [Crossref] [PubMed]
- Moradiellos J, Amor S, Córdoba M, et al. Functional Chest Wall Reconstruction With a Biomechanical Three-Dimensionally Printed Implant. Ann Thorac Surg 2017;103:e389-91. [Crossref] [PubMed]
- Tan D, Yao J, Hua X, et al. Application of 3D modeling and printing technology in accurate resection of complicated thoracic tumors. Ann Transl Med 2020;8:1342. [Crossref] [PubMed]
- Smelt J, Pontiki A, Jahangiri M, et al. Three-Dimensional Printing for Chest Wall Reconstruction in Thoracic Surgery: Building on Experience. Thorac Cardiovasc Surg 2020;68:352-6. [Crossref] [PubMed]
- Liu D, Wang Z, Qiu T, et al. Resection of a giant sternal chondrosarcoma and chest wall reconstruction: a case report. Ann Transl Med 2021;9:1706. [Crossref] [PubMed]
- Goldsmith I, Dovgalski L, Evans PL. 3D Printing Technology for Chest Wall Reconstruction With a Sternum-Ribs-Cartilage Titanium Implant: From Ideation to Creation. Innovations (Phila) 2023;18:67-72. [Crossref] [PubMed]
- Pontiki AA, Natarajan S, Parker FNH, et al. Chest Wall Reconstruction Using 3-Dimensional Printing: Functional and Mechanical Results. Ann Thorac Surg 2022;114:979-88. [Crossref] [PubMed]
- Wu Y, Chen N, Xu Z, et al. Application of 3D printing technology to thoracic wall tumor resection and thoracic wall reconstruction. J Thorac Dis 2018;10:6880-90. [Crossref] [PubMed]
- Sharaf B, Sabbagh MD, Vijayasekaran A, et al. Virtual surgical planning and three-dimensional printing in multidisciplinary oncologic chest wall resection and reconstruction: A case report. Int J Surg Case Rep 2018;47:52-6. [Crossref] [PubMed]
- Wen X, Gao S, Feng J, et al. Chest-wall reconstruction with a customized titanium-alloy prosthesis fabricated by 3D printing and rapid prototyping. J Cardiothorac Surg 2018;13:4. [Crossref] [PubMed]
- Cano JR, Escobar FH, Alonso DP, et al. Reconstruction of the anterior chest wall with a 3-dimensionally printed biodynamic prosthesis. J Thorac Cardiovasc Surg 2018;155:e59-60. [Crossref] [PubMed]
- Oswald N, Senanayake E, Naidu B, et al. Chest Wall Mechanics In Vivo With a New Custom-Made Three-Dimensional-Printed Sternal Prosthesis. Ann Thorac Surg 2018;105:1272-6. [Crossref] [PubMed]
- Wang L, Huang L, Li X, et al. Three-Dimensional Printing PEEK Implant: A Novel Choice for the Reconstruction of Chest Wall Defect. Ann Thorac Surg 2019;107:921-8. [Crossref] [PubMed]
- Wang B, Guo Y, Tang J, et al. Three-dimensional custom-made carbon-fiber prosthesis for sternal reconstruction after sarcoma resection. Thorac Cancer 2019;10:1500-2. [Crossref] [PubMed]
- Vannucci J, Scarnecchia E, Potenza R, et al. Dynamic titanium prosthesis based on 3D-printed replica for chest wall resection and reconstruction. Transl Lung Cancer Res 2020;9:2027-32. [Crossref] [PubMed]
- Albertal M, Vallejos J, Bellia G, et al. Changes in chest compression indexes with breathing underestimate surgical candidacy in patients with pectus excavatum: a computed tomography pilot study. J Pediatr Surg 2013;48:2011-6. [Crossref] [PubMed]
- Bellía-Munzón G, Sanjurjo D, Toselli L, et al. Novel index to estimate the cephalocaudal extent of the excavation in pectus excavatum: The Titanic index. J Pediatr Surg 2023;58:605-7. [Crossref] [PubMed]