Association of immune deficiency with prognosis and corticosteroid treatment benefits among patients with acute respiratory distress syndrome
Highlight box
Key findings
• Immune deficiency is an independent risk factor in acute respiratory distress syndrome (ARDS) and patients with immune deficiency with varying levels of severity of ARDS may benefit from different doses of corticosteroids.
What is known and what is new?
• The role of corticosteroids in the management of ARDS remains controversial. Many studies primarily focus on the effectiveness and optimal regimen of corticosteroids in treating ARDS patients, without deeply exploring the reasons for these variances.
• This study indicates that immune deficiency is an independent prognostic factor in ARDS. Patients with mild to moderate ARDS and immune deficiency may benefit from low-dose corticosteroid treatment, while those with severe ARDS and immune deficiency may benefit from both low and high-dose corticosteroid therapy.
What is the implication, and what should change now?
• Incorporating immune deficiency into the disease severity grading system based on the Berlin criteria may enhance personalized treatment approaches for ARDS patients.
• The findings warrant further validation through prospective, large-scale, multicenter randomized controlled trials.
Introduction
Acute respiratory distress syndrome (ARDS) is a common clinical syndrome characterized by acute hypoxic respiratory failure and non-cardiogenic lung infiltration (1). ARDS arises from a variety of causes, both infectious and non-infectious. These causes can lead to direct lung damage through local inflammation or indirect damage via systemic inflammation (2). Sepsis from different pathogens are notable causes of ARDS, among which pulmonary sepsis being the predominant source (3). Common non-infectious etiologies include pancreatitis, aspiration of stomach contents, severe traumatic injury with shock, transfusion-related acute lung injury (TRALI), and smoke inhalation or environment conditions, i.e., ARDS commonly seen in workers in coal mines “black lung” or gold mine workers (2). In the LUNG SAFE prospective cohort study, encompassing 459 intensive care units (ICUs) across 50 countries on five continents, 10.4% of ICU patients and 23.4% of those on mechanical ventilation met the Berlin definition criteria, a commonly used tool to assess the severity of ARDS by using respiratory parameters such as partial pressure of oxygen to fraction of inspired oxygen ratio (PaO2/FiO2) (3,4). The prevalence of ARDS has notably increased during the coronavirus disease 2019 (COVID-19) pandemic (2). The heterogeneity of ARDS, in terms of pathogenesis, clinical features, and treatment responses, presents challenges for clinical management. Advancements in understanding the epidemiology and risk factors, alongside differential diagnosis and personalized clinical management, are crucial for reducing the disease burden and enhancing patient quality of life.
Biomarkers are instrumental in identifying the main etiology of patients, stratifying risk, and predicting clinical outcomes (5). The discovery and verification of diagnostic or prognostic biomarkers for ARDS aid in screening high-risk populations and phenotypic analysis. Biomarkers used to identify alveolar and bronchiolar damage include surfactant protein D, Krebs von den lungen-6 (KL-6), and soluble receptor for advanced glycation end-products (sRAGEs); endothelial injury and coagulation markers like gelsolin, thrombomodulin, protein C, endocan, plasminogen activator inhibitor-1 (PAI or PAI-1), angiopoietin-2, von Willebrand factor (vWF); and treatment response biomarkers, particularly those related to lung inflammation, infection, C-reactive protein (CRP), and white blood cells (WBCs) (5). As understanding of ARDS deepens, research on biomarkers, particularly those related to pulmonary inflammation, is expanding. Current studies are increasingly focusing on the role of immune deficiency in patient prognosis, aligned with the immunopathological mechanisms of ARDS (5,6), such as inflammatory disorder and increased pulmonary endothelial and epithelial permeability (6).
Acute hypoxic respiratory failure is a leading cause for admission of immunocompromised patients to the ICU (7), with ARDS occurring in 35–75% of this population (8). A post hoc analysis of LUNG SAFE data revealed that patients with compromised immune function experienced higher in-hospital mortality compared to those with normal immune function (52.4% vs. 36.2%, P<0.001) (9), indicating that immunosuppression may independently predict mortality in ARDS patients (10). Current research primarily focuses on the causes of immune deficiency in ARDS patients and its impact on the efficacy of standard clinical treatments. Immune deficiency can result from a range of conditions, including genetic diseases, acquired diseases like acquired immune deficiency syndrome (AIDS) and hematologic malignancies, as well as treatments such as prolonged corticosteroid or chemotherapy use (11). Additionally, patients with various chronic and critical illnesses also exhibit degrees of immune suppression (11,12). Consequently, some researchers suggest classifying immune suppression based on specific immune system deficits, namely neutropenia, impaired humoral immunity mediated by B lymphocytes, and impaired cellular immunity mediated by T lymphocytes (11). In terms of treatment correlation, while researchers focus on non-invasive ventilation or extracorporeal membrane oxygenation (ECMO), it is argued that immune-compromised status might not independently predict ECMO weaning failure in ARDS patients (13).
Currently, ARDS treatment encompasses ventilation therapy, prone positioning, extracorporeal support, neuromuscular blockade, and corticosteroids (14). However, the role of corticosteroids in ARDS, septic shock, community-acquired pneumonia, and other severe diseases remains controversial (15). A multicenter randomized controlled trial (RCT) in 17 ICUs in Spain found that early dexamethasone administration reduced both the duration of mechanical ventilation (between-group difference 4.8 days, P<0.0001) and overall mortality (between-group difference 15.3%, P=0.005) in patients with moderate-to-severe ARDS (16). Another systematic review of 14 RCTs indicated that low doses (no more than 10 mg/kg/day methylprednisolone) and prolonged courses (more than 7 days) of corticosteroid treatment reduced ARDS mortality [lower dose: relative risk (RR) =0.69; 95% confidence interval (CI): 0.51–0.93; P=0.02; longer-course therapy: RR =0.60; 95% CI: 0.37–0.99; P=0.04] (17). The most common adverse event was hyperglycemia, though no statistical association was established (16). However, another RCT investigating early methylprednisolone use suggested that corticosteroids might increase long-term mortality if initiated 14 days after ARDS onset (18). These studies primarily focus on the effectiveness and optimal regimen of corticosteroids in treating ARDS patients, without deeply exploring the reasons for these variances in mortality associated with initiation of corticosteroids.
This study aims to investigate risk factors that may influence the effectiveness of corticosteroid treatment in ARDS patients through a retrospective cohort design. It further analyzes whether these factors can serve as independent risk factors for ARDS and identify patients who might benefit more from varying doses of corticosteroid treatment. These results could highlight the clinical significance of such risk factors in ARDS, providing a potential prediction system for ARDS prognosis assessment and a reference for personalized treatment of different ARDS subgroups. We present this article in accordance with the STROBE reporting checklist (available at https://jtd.amegroups.com/article/view/10.21037/jtd-24-109/rc).
Methods
Study patients and design
This single-center, retrospective cohort study was conducted at Zhongshan Hospital, Fudan University, Shanghai, China. We identified adult ARDS patients admitted between January 24, 2008, and September 12, 2022, according to the American European Consensus Conference (AECC) criteria, which defined the time of onset, imaging characteristics, oxygenation function, and so on (19). Considering the sample size distribution and data characteristics, we selected 2017 as the time node. The discovery dataset included patients admitted after 2017, while the validation dataset comprised those admitted before 2017. The primary source population consisted of patients experiencing respiratory failure during the study period. Eligible patients were 18 years or older, diagnosed with ARDS based on AECC criteria, which include initial clinical symptoms (such as pneumonia, aspiration, sepsis, and pancreatitis) or worsening respiratory symptoms within a week, bilateral lung infiltration on chest imaging, and hypoxemia. Exclusion criteria encompassed pregnancy or lactation, brain death, severe chronic obstructive pulmonary disease, or congestive heart failure. The study was conducted in accordance with the Declaration of Helsinki (as revised in 2013). The study was approved by the Ethics Committee of Zhongshan Hospital, Fudan University (No. 2021SQCJ2640) and individual consent for this retrospective analysis was waived.
In the discovery dataset, patients were categorized into “no corticosteroid treatment”, “low-dose corticosteroid treatment”, and “high-dose corticosteroid treatment” groups based on their maximum daily methylprednisolone equivalent. We compared baseline characteristics, clinical treatment courses, and outcomes across these groups and analyzed risk factors affecting the primary outcome. The study then examined the impact of this risk factor on the prognosis of different patient subgroups, with results further validated in the validation dataset. The study also discussed the impact on the efficacy of different corticosteroid doses, aiming to provide a basis for personalized medication in ARDS patients.
Definitions
We classified ARDS patients into different severity categories based on the Berlin criteria (4): mild ARDS as 200 mmHg > PaO2/FiO2 ≤300 mmHg, moderate ARDS as 100 mmHg > PaO2/FiO2 ≤200 mmHg, and severe ARDS as PaO2/FiO2 ≤100 mmHg.
The low-dose corticosteroid treatment group primarily comprised ARDS patients receiving a maximum daily dose of less than 80 mg of methylprednisolone equivalent. Conversely, the high-dose group included ARDS patients with a daily dose exceeding 80 mg. To ensure consistency, doses of various corticosteroids, including cortisone, dexamethasone, prednisone, and hydrocortisone, were converted to their methylprednisolone equivalents, considering that 4 mg of methylprednisolone is equivalent to 0.75 mg dexamethasone, 20 mg hydrocortisone, 25 mg cortisone, or 5 mg prednisone (20).
Patients classified as having immune deficiency included those on long-term (>3 months) or high-dose (>0.5 mg/kg/day) steroid therapy or other immunosuppressants, recipients of solid organ transplants, patients with solid tumors or hematological malignancies undergoing chemotherapy within the past 5 years, and those with primary immune deficiencies (i.e., X-linked agammaglobulinemia, DiGeorge syndrome, etc.), regardless of the time since diagnosis and treatment (21).
Data collection
Data were extracted and compiled from the medical record system, including: (I) basic demographic characteristics: age, sex, smoking status, immune deficiency status, pathogenesis, comorbidities, experimental examination results, and Acute Physiologic and Chronic Health Evaluation (APACHE) II score; (II) corticosteroid usage, administered intravenously (including cortisone, methylprednisolone, dexamethasone, prednisone, and hydrocortisone), with dosages converted to methylprednisolone equivalents, and duration of treatment for each patient; (III) respiratory support methods: noninvasive mechanical ventilation, invasive mechanical ventilation, and ECMO; and (IV) outcome measures: primary outcome was 28-day mortality post disease onset, with secondary outcomes including in-hospital mortality and hospitalization duration.
Statistical analysis
Statistical analysis was performed using Stata, version 17.0, with continuous variables grouped using X-tile, version 3.6.1. Categorical variables were expressed as percentages. Baseline characteristics, interventions, and outcomes between different corticosteroid dose groups, and between patients with or without immune deficiency, were compared using Pearson chi-squared test or Fisher’s exact test. Continuous variables were presented as median and interquartile ranges. One-way analysis of variance (ANOVA) or Kruskal-Wallis tests were employed to compare groups receiving different corticosteroid doses, while unpaired, two-tailed Student’s t-test or Mann-Whitney U test were used for comparisons between groups with or without immune deficiency. Kaplan-Meier curves and log-rank tests assessed survival outcomes across different severity subgroup multivariate Cox regression analyzed risk factors impacting patient survival and the effect of immune deficiency on corticosteroid treatment efficacy in various severity groups. All statistical analyses were two-sided, with a P<0.05 considered statistically significant.
Results
Risk factors associated with survival across different corticosteroid dose groups
In the discovery dataset, we compared basic characteristics, clinical treatments, pathological parameters, and prognoses among groups receiving various corticosteroid doses, as detailed in Table S1. Risk factors potentially influencing survival in these groups were analyzed using chi-squared test or Fisher’s exact test (Table S2). This analysis revealed associations between PaO2/FiO2, immune deficiency, neutrophil and lymphocyte counts, and overall and subgroup outcomes, suggesting these factors might influence the efficacy of corticosteroid treatment. Subsequently, multivariate Cox regression analysis was utilized to examine the relationship between potential risk factors and survival (Table S3), encompassing age, sex, smoking status, PaO2/FiO2, disease etiology, comorbidities, immune deficiency, platelet count (PLT), procalcitonin (PCT), prothrombin time (PT), and CRP. The findings indicated that immune deficiency could be a significant risk factor affecting therapeutic outcomes at different dosages.
Baseline patient characteristics
The study flow is depicted in Figure 1. We screened 6,110 patients with respiratory failure for ARDS, ultimately including 657 ARDS patients in the discovery and validation datasets. In the discovery dataset (n=357), 244 (68.35%) patients were male, with a median age of 67 [57–75] years. Of these, 160 (44.82%) had immune deficiencies, and 197 (55.18%) did not. The validation dataset (n=300) comprised 196 (65.33%) male patients with a median age of 63 [50–73] years, including 101 (33.67%) with immune deficiencies and 199 (66.33%) without (Table 1). Within the discovery dataset, 193 (54.06%) patients died within 28 days, and 219 (61.34%) died in-hospital. In the validation dataset, these outcomes were observed in 161 (53.67%) and 192 (64.00%) patients, respectively (Table 2).
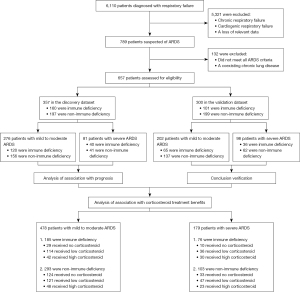
Table 1
Variables | Discovery dataset | Validation dataset | |||||||
---|---|---|---|---|---|---|---|---|---|
All patients (n=357) | Immune deficiency (n=160) | Non-immune deficiency (n=197) | P value | All patients (n=300) | Immune deficiency (n=101) | Non-immune deficiency (n=199) | P value | ||
Age (years) | 67 [57–75] | 64 [56–73] | 68 [59–78] | 0.009 | 63 [50–73] | 60 [42–69] | 64 [53–76] | 0.002 | |
Sex, male | 244 (68.35) | 104 (65.00) | 140 (71.07) | 0.22 | 196 (65.33) | 55 (54.46) | 141 (70.85) | 0.005 | |
Smoking | 101 (28.29) | 53 (33.13) | 48 (24.37) | 0.07 | 53 (17.67) | 15 (14.85) | 38 (19.10) | 0.36 | |
PaO2/FiO2 | 189.5 [111–259] | 165 [102–248] | 198 [117–266] | 0.06 | 121.5 [93–155.5] | 126 [82–165] | 116 [94–150] | 0.90 | |
APACHE II score | 19 [11–28] | 22.5 [14–31] | 17 [11–27] | 0.11 | 16 [11–21] | 18 [13–23] | 15 [11–20] | <0.001 | |
Cause of ARDS | |||||||||
Pneumonia | 265 (74.23) | 130 (81.25) | 135 (68.53) | 0.006 | 163 (54.33) | 65 (64.36) | 98 (49.25) | 0.01 | |
Sepsis | 17 (4.76) | 5 (3.13) | 12 (6.09) | 0.19 | 52 (17.33) | 22 (21.78) | 30 (15.08) | 0.15 | |
Aspiration | 12 (3.36) | 4 (2.50) | 8 (4.06) | 0.42 | 52 (17.33) | 11 (10.89) | 41 (20.60) | 0.04 | |
Trauma | 10 (2.80) | 4 (2.50) | 6 (3.05) | >0.99 | 36 (12.00) | 6 (5.94) | 30 (15.08) | 0.02 | |
Others | 53 (14.85) | 17 (10.63) | 36 (18.27) | 0.04 | – | – | – | – | |
Any comorbidity | |||||||||
Diabetes | 77 (21.57) | 31 (19.38) | 46 (23.35) | 0.36 | 37 (12.33) | 11 (10.89) | 26 (13.07) | 0.59 | |
Hypertension | 144 (40.34) | 61 (38.13) | 83 (42.13) | 0.44 | 115 (38.33) | 38 (37.62) | 77 (38.69) | 0.86 | |
Chronic liver disease | 41 (11.48) | 20 (12.50) | 21 (10.66) | 0.59 | 11 (3.67) | 4 (3.96) | 7 (3.52) | >0.99 | |
Respiratory support | |||||||||
NIMV | 281 (78.71) | 124 (77.50) | 157 (79.70) | 0.61 | 157 (52.33) | 41 (40.59) | 116 (58.29) | 0.004 | |
IMV | 184 (51.54) | 82 (51.25) | 102 (51.78) | 0.92 | 173 (57.67) | 47 (46.53) | 126 (63.32) | 0.005 | |
ECMO | 19 (5.32) | 11 (6.88) | 8 (4.06) | 0.24 | – | – | – | – |
Data are presented as n (%) or median [IQR]. For continuous variables, t-test or Mann-Whitney U test was used to calculate the P value unless otherwise noted. For categorical variables, chi-square test was used to calculate the P value unless otherwise noted. In the discovery dataset, most of the baseline characteristics were comparable between the immune deficient and non-immune deficient groups, whereas in the validation dataset, a greater number of baseline characteristics were unevenly distributed between the two groups. ARDS, acute respiratory distress syndrome; PaO2, partial pressure of oxygen; FiO2, fraction of inspired oxygen; APACHE II, Acute Physiologic and Chronic Health Evaluation II; NIMV, noninvasive mechanical ventilation; IMV, invasive mechanical ventilation; ECMO, extracorporeal membrane oxygenation; IQR, interquartile range.
Table 2
Outcomes | Discovery dataset | Validation dataset | |||||||
---|---|---|---|---|---|---|---|---|---|
All patients (n=357) | Immune deficiency (n=160) | Non-immune deficiency (n=197) | P value | All patients (n=300) | Immune deficiency (n=101) | Non-immune deficiency (n=199) | P value | ||
28-day mortality | 193 (54.06) | 102 (63.75) | 91 (46.19) | 0.001 | 161 (53.67) | 71 (70.30) | 90 (45.23) | <0.001 | |
In-hospital mortality | 219 (61.34) | 115 (71.88) | 104 (52.79) | <0.001 | 192 (64.00) | 83 (82.18) | 109 (54.77) | <0.001 | |
Length of hospitalization (days) | 14 [7–24] | 15 [7–25] | 13 [7–20.5] | 0.16 | 14 [7–24] | 11 [5–22] | 15 [9–25] | 0.04 |
Data are presented as n (%) or median [IQR]. For continuous variables, t-test or Mann-Whitney U test was used to calculate the P value unless otherwise noted. For categorical variables, chi-square test was used to calculate the P value unless otherwise noted. In the discovery dataset, the proportion of 28-day mortality and in-hospital mortality was different between the immune deficient and non-immune deficient groups. In the validation dataset, there are also differences in the distribution of length of hospitalization. ARDS, acute respiratory distress syndrome; IQR, interquartile range.
Additional comprehensive characteristics and clinicopathological indicators are presented in Table 1 and Figure 2. Age distribution differed significantly between groups in the discovery dataset (P=0.009). In the validation dataset, except for smoking status, PaO2/FiO2 and comorbidities, all other parameters showed significant differences between groups. Both datasets showed notable differences in PT and interleukin (IL)-10.
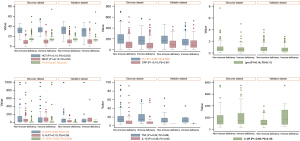
Independent risk factors for mortality in ARDS
After verifying the proportional hazards assumption, we conducted a multivariate Cox regression analysis (Figure 3), incorporating variables such as age, sex, smoking status, immune deficiency, disease severity, comorbidities (diabetes and chronic liver disease), and select physiological parameters (PLT, PCT, PT, CRP). IL-6 and IL-10 were excluded due to multicollinearity or significant data loss. The analysis identified immune deficiency [discovery dataset: hazard ratio (HR) =1.677; 95% CI: 1.192–2.359; P=0.003; validation dataset: HR =1.856; 95% CI: 1.266–2.721; P=0.002] and severe disease severity (discovery dataset: HR =1.551; 95% CI: 1.024–2.349; P=0.04; validation dataset: HR =3.104; 95% CI: 1.315–7.327; P=0.01) as independent risk factors for overall survival. Additionally, increased age in the discovery dataset (HR =1.013; 95% CI: 1.000–1.025; P=0.045) and elevated PCT in the validation dataset (HR =1.036; 95% CI: 1.013–1.059; P=0.002) were identified as potential, albeit weaker, risk factors for overall survival.
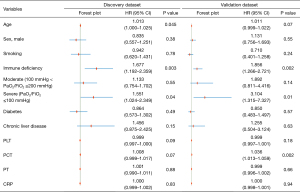
Relationship between immune deficiency and prognosis in patients with different severities of ARDS
To assess the impact of immune deficiency on the prognosis of ARDS patients of varying severities, Kaplan-Meier survival analysis was employed (Figure 4). In both the discovery (HR =1.638; 95% CI: 1.233–2.174) and validation datasets (HR =1.830; 95% CI: 1.334–2.510), non-immune deficiency patients exhibited significantly better overall survival compared to their immune-deficient counterparts, with the log-rank test results of both were P<0.001. These findings indicate that immune deficiency is potentially clinically significant for the survival outcome of ARDS patients.
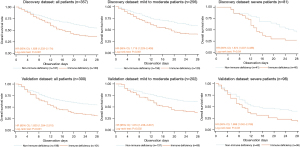
We then categorized patients into mild-to-moderate and severe disease subgroups. Among those with mild-to-moderate ARDS, immune-deficient patients showed poorer survival in both the discovery (HR =1.719; 95% CI: 1.229–2.406; log-rank test P=0.001) and validation datasets (HR =1.874; 95% CI: 1.238–2.837; log-rank test P=0.002). Similar trends were observed in patients with severe ARDS (discovery dataset: HR =1.874; 95% CI: 1.007–3.488; log-rank test P=0.04; validation dataset: HR =1.698; 95% CI: 1.042–2.768; log-rank test P=0.03).
Relationship between immune deficiency and corticosteroid treatment benefits in patients with different severities of ARDS
Furthermore, we explored whether ARDS patients with immune deficiency could benefit from corticosteroid treatment. For immune-deficient ARDS patients, a relationship was noted between corticosteroid use and mortality risk. Compared to patients not receiving corticosteroids or those on high-dose treatment, those on low-dose corticosteroid therapy exhibited a lower mortality risk. This trend was consistent across both mild-to-moderate and severe ARDS patients (Table 3).
Table 3
Patients | 28-day mortality, n/total (%) | P value | ||||||
---|---|---|---|---|---|---|---|---|
No corticosteroid | Low corticosteroid | High corticosteroid | Total | No vs. low | No vs. high | Low vs. high | ||
Patients with mild to moderate ARDS (n=478) | ||||||||
Immune deficiency (n=185) | 24/29 (82.76) | 57/114 (50.00) | 32/42 (76.19) | <0.001 | 0.001 | 0.51 | 0.003 | |
Non-Immune deficiency (n=293) | 46/124 (37.10) | 49/121 (40.50) | 23/48 (47.92) | 0.43 | 0.59 | 0.19 | 0.38 | |
Patients with severe ARDS (n=179) | ||||||||
Immune deficiency (n=76) | 10/10 (100.00) | 23/36 (63.89) | 27/30 (90.00) | 0.007 | 0.04 | 0.56 | 0.01 | |
Non-Immune deficiency (n=103) | 17/33 (51.52) | 30/47 (63.83) | 16/23 (69.57) | 0.35 | 0.27 | 0.18 | 0.64 |
The efficacy of corticosteroid was analyzed by chi-square test among different subgroups. For ARDS patients with immune deficiency, the probability of death was lower when low-dose glucocorticoids were used than no corticosteroid, but the probability was increased when high-dose corticosteroid were used compared with low-dose corticosteroid, and this conclusion was independent of the severity of the disease. ARDS, acute respiratory distress syndrome.
Further analysis revealed that immune deficiency and corticosteroid treatment may interactively affect overall patient survival. The interaction term statistics were lower than 0.001 and 0.005 for patients with mild-to-moderate and severe ARDS, respectively (Table 4). Considering these interactions, it was observed that patients with mild-to-moderate ARDS and immune deficiency could benefit from low-dose corticosteroid treatment (HR =0.409; 95% CI: 0.249–0.671). Similarly, patients with severe ARDS and immune deficiency might benefit from both low-dose (HR =0.299; 95% CI: 0.136–0.654) and high-dose corticosteroid treatments (HR =0.458; 95% CI: 0.214–0.981).
Table 4
Patients | Patients with mild to moderate ARDS (n=478) | Patients with severe ARDS (n=179) | |||||||||||
---|---|---|---|---|---|---|---|---|---|---|---|---|---|
P value† |
Low corticosteroid | High corticosteroid | P value† |
Low corticosteroid | High corticosteroid | ||||||||
HR (95% CI) | P value | HR (95% CI) | P value | HR (95% CI) | P value | HR (95% CI) | P value | ||||||
Immune deficiency | <0.001 | 0.409 (0.249–0.671) |
<0.001 | 0.730 (0.424–1.258) |
0.26 | 0.005 | 0.299 (0.136–0.654) |
0.002 | 0.458 (0.214–0.981) |
0.04 | |||
Non-immune deficiency | 1.089 (0.728–1.628) |
0.68 | 1.334 (0.809–2.202) |
0.26 | 1.171 (0.645–2.125) |
0.60 | 1.507 (0.760–2.989) |
0.24 |
The effect of immune deficiency on the efficacy of corticosteroid was analyzed by multivariate Cox regression. For mild to moderate ARDS patients, the benefit of low dose corticosteroid was more obvious in immune deficiency patients. However, for patients with severe ARDS, both low-dose and high-dose corticosteroid benefit significantly in immune deficiency patients. †, the interaction between immune deficiency and glucocorticoid treatment. ARDS, acute respiratory distress syndrome; HR, hazard ratio; CI, confidence interval.
Discussion
This study initially analyzed risk factors affecting the prognosis of ARDS patients undergoing varying doses of corticosteroid treatment. Immune deficiency was found to influence the 28-day mortality and in-hospital mortality of these patients, demonstrating a beneficial response to different corticosteroid dosages. Additionally, subgroup analysis evaluating the severity of ARDS based on the Berlin criteria (PaO2/FiO2) indicated that immune deficiency acted as an independent risk factor for overall survival. Mild to moderate ARDS patients with immune deficiency benefited from low-dose corticosteroid treatment, while severe ARDS patients with immune deficiency benefited from both low and high doses.
Comparing immune-deficient and non-immune-deficient patients revealed that the former had a lower average age (discovery dataset: P=0.009; validation dataset: P=0.002), aligning with findings from Cortegiani et al. (9). ARDS patients with immune deficiency were more commonly affected by pulmonary inflammation (discovery dataset: 81.25% vs. 68.53%, P=0.006; validation dataset: 64.36% vs. 49.25%, P=0.01), and exhibited higher levels of PLT, PT, IL-6, and IL-10. These findings align with the conclusions of most current studies (9,10,13,22). However, Rilinger et al. (10) observed lower WBC, PLT, hemoglobin (HB), and hematocrit (HCT) levels in the immune-deficient group, speculating these as potential causes for increased mortality risk in these patients. Their study, however, only analyzed the impact of immune deficiency on patients with severe ARDS. The in-hospital mortality among our immune-compromised ARDS patients was higher than that in a post hoc analysis of LUNG SAFE data by Cortegiani et al. (9), where 584 (20.8%) of 2,813 ARDS patients were immune deficient.
The body’s immune defense mechanism plays a crucial role in the development of ARDS. Proinflammatory structural components and endogenous molecules related to cell damage can attach to Toll-like receptors on lung epithelial cells and alveolar macrophages, activating the innate immune system (23). The ensuing formation of neutrophil extracellular traps and release of histone proteins aid in capturing pathogenic microorganisms (24), along with the generation of reactive oxygen species, leukocyte proteases, chemokines, and cytokines to neutralize pathogens (25). However, while these mechanisms inhibit inflammation, they may also exacerbate lung injury. Thus, balancing their anti-infective effects and potential for alveolar damage is vital. Corticosteroids, widely used in ARDS since their inception (26,27), are linked to significant improvements in alveolar-capillary membrane permeability and the regulation of inflammation and tissue repair mediators (28). Ameliorating inflammation is fundamental to restoring homeostasis in ARDS patients (29). Variations in inflammation-related factors among immune-deficient patients may influence their prognosis and response to corticosteroid therapy.
Previous research indicates that certain cytokine levels are elevated in immune-deficient ARDS patients, yet levels of C-C motif chemokine ligand 22 (CCL22) are low (30). CCL22 recruits immunosuppressive regulatory T cells (Tregs) to modulate the local immune response (31,32), and diminished Treg levels may increase the likelihood of T lymphocyte dysregulation, potentially explaining the higher mortality in these patients. Additionally, considering the role of neutrophils in both ARDS pathogenesis and immune deficiency (1,33-35), changes in various biomarkers, pro-inflammatory cytokines, and chemokines in neutropenic patients are also noteworthy.
Most current studies have focused on the relationship between immune deficiency and non-pharmacological treatments, such as ventilation strategies, with less exploration into the response of immune-deficient patients to corticosteroid treatment. Our study integrated immune deficiency with disease severity to more accurately predict patient outcomes. Moreover, in analyzing the response to corticosteroids, we accounted for different dosages, potentially advancing personalized management of ARDS.
The study has several limitations. First, as a single-center retrospective cohort study, there are inherent risks of selection and reporting biases, and the potential for unknown confounders affecting the analysis. To mitigate this, patients were divided into discovery and validation datasets to enhance the reliability of the conclusions. Second, the study did not use the widely accepted Berlin definition to enroll ARDS patients, primarily due to limitations of the Berlin definition and the prolonged enrollment period, during which some patients in the validation cohort were diagnosed with ARDS prior to the introduction of the Berlin definition. Third, the classification of the immune-deficient population within the ARDS cohort was solely based on the definition and baseline characteristics of patients. Aside from malignancies and long-term corticosteroid use, current data struggle to accurately differentiate between immune-deficient and non-immune-deficient patients, potentially leading to misclassification bias. Fourth, the study did not consider the use of pharmacological and non-pharmacological treatments other than corticosteroids. However, the prognosis of patients with immune deficiency may depend on the nature of the underlying disease and associated treatments. The exclusion of these factors could limit the generalizability of our findings. Therefore, while our results may offer a foundation for personalized treatment of ARDS patients, due to the retrospective design of our study, the findings require validation through large-scale, multicenter, prospective studies.
Conclusions
This study showed that immune deficiency is an independent risk factor for mortality in ARDS. Patients with mild to moderate ARDS and immune deficiency may benefit from low-dose glucocorticoid treatment, while those with severe ARDS and immune deficiency may benefit from both low and high-dose glucocorticoid therapy, providing a basis for personalized medication in ARDS management.
Acknowledgments
Funding: This study was supported by
Footnote
Reporting Checklist: The authors have completed the STROBE reporting checklist. Available at https://jtd.amegroups.com/article/view/10.21037/jtd-24-109/rc
Data Sharing Statement: Available at https://jtd.amegroups.com/article/view/10.21037/jtd-24-109/dss
Peer Review File: Available at https://jtd.amegroups.com/article/view/10.21037/jtd-24-109/prf
Conflicts of Interest: All authors have completed the ICMJE uniform disclosure form (available at https://jtd.amegroups.com/article/view/10.21037/jtd-24-109/coif). Y.W. reports that this study was supported by the National Natural Science Foundation of China (No. 82200090). Y.S. reports that this study was supported by the Shanghai Municipal Science and Technology Major Project (No. ZD2021CY001), the National Natural Science Foundation of China (Nos. 82130001 and 82272243), the Science and Technology Commission of Shanghai Municipality (Nos. 20Z11901000, 20DZ2261200, 20XD1401200, and 22Y11900800), the Clinical Research Plan of SHDC (No. SHDC2020CR5010-002), the Shanghai Municipal Key Clinical Specialty (No. shslczdzk02201), and the Shanghai Municipal Health Commission and Shanghai Municipal Administrator of Traditional Chinese Medicine [No. ZY(2021-2023)-0207-01]. The other authors have no conflicts of interest to declare.
Ethical Statement: The authors are accountable for all aspects of the work in ensuring that questions related to the accuracy or integrity of any part of the work are appropriately investigated and resolved. The study was conducted in accordance with the Declaration of Helsinki (as revised in 2013). The study was approved by the Ethics Committee of Zhongshan Hospital, Fudan University (No. 2021SQCJ2640) and individual consent for this retrospective analysis was waived.
Open Access Statement: This is an Open Access article distributed in accordance with the Creative Commons Attribution-NonCommercial-NoDerivs 4.0 International License (CC BY-NC-ND 4.0), which permits the non-commercial replication and distribution of the article with the strict proviso that no changes or edits are made and the original work is properly cited (including links to both the formal publication through the relevant DOI and the license). See: https://creativecommons.org/licenses/by-nc-nd/4.0/.
References
- Meyer NJ, Gattinoni L, Calfee CS. Acute respiratory distress syndrome. Lancet 2021;398:622-37. [Crossref] [PubMed]
- Bos LDJ, Ware LB. Acute respiratory distress syndrome: causes, pathophysiology, and phenotypes. Lancet 2022;400:1145-56. [Crossref] [PubMed]
- Bellani G, Laffey JG, Pham T, et al. Epidemiology, Patterns of Care, and Mortality for Patients With Acute Respiratory Distress Syndrome in Intensive Care Units in 50 Countries. JAMA 2016;315:788-800. [Crossref] [PubMed]
- ARDS Definition Task Force. Acute respiratory distress syndrome: the Berlin Definition. JAMA 2012;307:2526-33. [PubMed]
- Sivapalan P, Bonnesen B, Jensen JU. Novel Perspectives Regarding the Pathology, Inflammation, and Biomarkers of Acute Respiratory Distress Syndrome. Int J Mol Sci 2020;22:205. [Crossref] [PubMed]
- Huppert LA, Matthay MA, Ware LB. Pathogenesis of Acute Respiratory Distress Syndrome. Semin Respir Crit Care Med 2019;40:31-9. [Crossref] [PubMed]
- Azoulay E, Pène F, Darmon M, et al. Managing critically Ill hematology patients: Time to think differently. Blood Rev 2015;29:359-67. [Crossref] [PubMed]
- Azoulay E, Lemiale V, Mokart D, et al. Acute respiratory distress syndrome in patients with malignancies. Intensive Care Med 2014;40:1106-14. [Crossref] [PubMed]
- Cortegiani A, Madotto F, Gregoretti C, et al. Immunocompromised patients with acute respiratory distress syndrome: secondary analysis of the LUNG SAFE database. Crit Care 2018;22:157. [Crossref] [PubMed]
- Rilinger J, Zotzmann V, Bemtgen X, et al. Influence of immunosuppression in patients with severe acute respiratory distress syndrome on veno-venous extracorporeal membrane oxygenation therapy. Artif Organs 2021;45:1050-60. [Crossref] [PubMed]
- Ferreyro BL, Munshi L. Causes of acute respiratory failure in the immunocompromised host. Curr Opin Crit Care 2019;25:21-8. [Crossref] [PubMed]
- Angus DC, Opal S. Immunosuppression and Secondary Infection in Sepsis: Part, Not All, of the Story. JAMA 2016;315:1457-9. [Crossref] [PubMed]
- Cheng CF, Chen YY, Shih MC, et al. Extracorporeal Membrane Oxygenation in Immunocompromised Patients With Acute Respiratory Distress Syndrome-A Retrospective Cohort Study. Front Med (Lausanne) 2021;8:755147. [Crossref] [PubMed]
- Gorman EA, O'Kane CM, McAuley DF. Acute respiratory distress syndrome in adults: diagnosis, outcomes, long-term sequelae, and management. Lancet 2022;400:1157-70. [Crossref] [PubMed]
- Chan ED, Chan MM, Chan MM, et al. Use of glucocorticoids in the critical care setting: Science and clinical evidence. Pharmacol Ther 2020;206:107428. [Crossref] [PubMed]
- Villar J, Ferrando C, Martínez D, et al. Dexamethasone treatment for the acute respiratory distress syndrome: a multicentre, randomised controlled trial. Lancet Respir Med 2020;8:267-76. [Crossref] [PubMed]
- Junhai Z, Bangchuan H, Shijin G, et al. Glucocorticoids for acute respiratory distress syndrome: A systematic review with meta-analysis and trial sequential analysis. Eur J Clin Invest 2021;51:e13496. [Crossref] [PubMed]
- Steinberg KP, Hudson LD, Goodman RB, et al. Efficacy and safety of corticosteroids for persistent acute respiratory distress syndrome. N Engl J Med 2006;354:1671-84. [Crossref] [PubMed]
- Bernard GR, Artigas A, Brigham KL, et al. The American-European Consensus Conference on ARDS. Definitions, mechanisms, relevant outcomes, and clinical trial coordination. Am J Respir Crit Care Med 1994;149:818-24. [Crossref] [PubMed]
- Liu J, Zhang S, Dong X, et al. Corticosteroid treatment in severe COVID-19 patients with acute respiratory distress syndrome. J Clin Invest 2020;130:6417-28. [Crossref] [PubMed]
- Azoulay E, Russell L, Van de Louw A, et al. Diagnosis of severe respiratory infections in immunocompromised patients. Intensive Care Med 2020;46:298-314. [Crossref] [PubMed]
- Coudroy R, Frat JP, Ehrmann S, et al. High-flow nasal oxygen alone or alternating with non-invasive ventilation in critically ill immunocompromised patients with acute respiratory failure: a randomised controlled trial. Lancet Respir Med 2022;10:641-9. [Crossref] [PubMed]
- Opitz B, van Laak V, Eitel J, et al. Innate immune recognition in infectious and noninfectious diseases of the lung. Am J Respir Crit Care Med 2010;181:1294-309. [Crossref] [PubMed]
- Mantovani A, Cassatella MA, Costantini C, et al. Neutrophils in the activation and regulation of innate and adaptive immunity. Nat Rev Immunol 2011;11:519-31. [Crossref] [PubMed]
- Imai Y, Kuba K, Neely GG, et al. Identification of oxidative stress and Toll-like receptor 4 signaling as a key pathway of acute lung injury. Cell 2008;133:235-49. [Crossref] [PubMed]
- Ashbaugh DG, Bigelow DB, Petty TL, et al. Acute respiratory distress in adults. Lancet 1967;2:319-23. [Crossref] [PubMed]
- Ashbaugh DG, Maier RV. Idiopathic pulmonary fibrosis in adult respiratory distress syndrome. Diagnosis and treatment. Arch Surg 1985;120:530-5. [Crossref] [PubMed]
- Meduri GU, Annane D, Chrousos GP, et al. Activation and regulation of systemic inflammation in ARDS: rationale for prolonged glucocorticoid therapy. Chest 2009;136:1631-43. [Crossref] [PubMed]
- Annane D, Pastores SM, Arlt W, et al. Critical illness-related corticosteroid insufficiency (CIRCI): a narrative review from a Multispecialty Task Force of the Society of Critical Care Medicine (SCCM) and the European Society of Intensive Care Medicine (ESICM). Intensive Care Med 2017;43:1781-92. [Crossref] [PubMed]
- Nguyen J, Thompson JM, Balcarcel DR, et al. Immunocompromised Children With Acute Respiratory Distress Syndrome Possess a Distinct Circulating Inflammatory Profile. Crit Care Explor 2023;5:e0844. [Crossref] [PubMed]
- Anz D, Rapp M, Eiber S, et al. Suppression of intratumoral CCL22 by type i interferon inhibits migration of regulatory T cells and blocks cancer progression. Cancer Res 2015;75:4483-93. [Crossref] [PubMed]
- Wiedemann GM, Knott MM, Vetter VK, et al. Cancer cell-derived IL-1α induces CCL22 and the recruitment of regulatory T cells. Oncoimmunology 2016;5:e1175794. [Crossref] [PubMed]
- Ware LB, Matthay MA. The acute respiratory distress syndrome. N Engl J Med 2000;342:1334-49. [Crossref] [PubMed]
- Matthay MA, Ware LB, Zimmerman GA. The acute respiratory distress syndrome. J Clin Invest 2012;122:2731-40. [Crossref] [PubMed]
- Chen Y, Huang B, Zhao Y, et al. Increased p300/CBP expression in acute respiratory distress syndrome is associated with interleukin-17 and prognosis. Clin Respir J 2020;14:791-9. [Crossref] [PubMed]