Dynamic decline of lymphocytes predicts extracorporeal membrane oxygenation-related infections: a retrospective observational study
Highlight box
Key findings
• A decrease in lymphocytes was significantly associated with an increased risk of extracorporeal membrane oxygenation (ECMO)-related infection.
What is known and what is new?
• The incidence of ECMO-related infection remains high.
• Dynamic decline of lymphocytes is associated with ECMO-related infection.
What is the implication, and what should change now?
• Early dynamic decline of lymphocytes is a risk factor for ECMO-related infection. Close attention should be given on immune monitoring in patients receiving ECMO support. Early identification of ECMO-related infection is critically important for patient management.
Introduction
Extracorporeal membrane oxygenation (ECMO) provides cardiopulmonary support to patients with reversible refractory respiratory and circulatory failure (1-3). The application of ECMO has dramatically increased worldwide over the last two decades (4). The mortality of patients supported by ECMO remains high despite great progress in the management of patients and advances in ECMO circuit technology (5-8).
Infection is a common complication during ECMO support. The overall incidence of infection varies greatly among centers between 9% and 65% during ECMO (9,10). Infections contribute to adverse outcomes including delayed lung recovery (11), prolonged hospital stays (12), treatment failure (13) and increased mortality (14,15). To develop clinical treatments and guide future studies on the prevention and management of high-risk patients, it is crucial to identify the risk factors for ECMO-related infections. More attention should be given to the immune system; however, the duration of ECMO (9) and age (15) are widely perceived as contributing to infection.
Lymphocytes are the cornerstone component of the adaptive immune system, which distinguishes itself from foreign cells and provides specialized immune defense. Lymphopenia is related to nosocomial infections and poor prognosis in several illnesses, including sepsis (16) and cancer (17). There is relatively little research on the correlation between lymphocyte count and infectious complications in patients on ECMO. Nevertheless, the degree of lymphopenia was associated with age and comorbidities (18). Due to the high heterogeneity of critically ill patients, it is appropriate to classify them by the dynamic changes in lymphocytes.
Hence, we conducted this retrospective study to examine the relationships between dynamic decreases in lymphocyte counts and ECMO-related infections as well as patient outcomes. We present this article in accordance with the STROBE reporting checklist (available at https://jtd.amegroups.com/article/view/10.21037/jtd-23-1912/rc) (19).
Methods
Ethical approval
The study was conducted in accordance with the Declaration of Helsinki (as revised in 2013) and was approved by the Ethics Committee of Zhongda Hospital Affiliated to Southeast University (No. 2022ZDSYLL177-P01). Informed consent was waived due to the retrospective observational study design requiring no intervention and posing no impact on the diagnosis and treatment of patients.
Study design and participants
This retrospective observational study was conducted using electronic health records data from the Chinese Database in Intensive Care (CDIC) database. The CDIC database contains more than 12,000 patients admitted to a general intensive care unit (ICU) in Zhongda Hospital, Southeast University, Nanjing, China, from January 2014 to July 2022.
Patients who were admitted to the general ICU from January 1, 2011 to December 31, 2019 and who received ECMO support were included in the study. The exclusion criteria were as follows: (I) under 18 years of age; (II) ECMO support duration less than 48 hours; and (III) occurrence of nosocomial infections before ECMO support. Cases beyond December 31, 2019 were not included because the severe acute respiratory syndrome coronavirus 2 (SARS-CoV-2) pandemic may have affected the provision of prehospital and intensive care services.
Diagnostic criteria for ECMO-related infection
The data were collected by S.L. ECMO-related infection was diagnosed by two intensivists (T.H. and C.W.). ECMO-related infection was defined as nosocomial infections which arose more than 24 hours after ECMO initiation and diagnosed less than 48 hours after weaning from ECMO (12), including ventilator-associated pneumonia (VAP), hospital-acquired blood stream infection (HA-BSI) and urinary tract infection (UTI) as listed in Table S1. Only the first episode of infection was included in the analysis. VAP was defined as pneumonia that developed more than 48–72 hours after endotracheal intubation or less than 48 hours after extubation. VAP was suspected if at least one of the following clinical manifestations were detected: new onset of fever, purulent sputum, a leukocyte count >12×109/L or <4×109/L and decreased oxygenation with at least two or more radiological evidence of new onset or progressive infiltrates. VAP was diagnosed based on clinical manifestations and radiological evidence plus quantitative distal bronchoalveolar lavage cultures growing ≥104 colony-forming units (CFU)/mL or quantitative endotracheal aspirate cultures growing ≥105 CFU/mL (20-22). HA-BSI was defined as the isolation of pathogenic organism(s) from at least one blood culture 48 hours or more after hospital admission (23). The detailed HA-BSI definitions are available in Table S1. UTI was defined as manifestations consistent with UTI along with ≥103 CFU/mL of at least one pathogenic organism in a single catheter urine sample or a midstream urine sample obtained within 48 hours after removal (24,25). Multidrug resistant (MDR) bacteria were defined according to the Centers for Disease Control (26).
Data collection
The following baseline patient data and ECMO-related variables were collected: demographics (i.e., gender, age, body mass index), main diagnosis at admission, reason for ECMO initiation, type of ECMO support, comorbidities (i.e., hypertension, diabetes mellitus), immunocompromised status (27), broad-spectrum antibiotic use within 48 hours before ECMO initiation, illness severity at admission stratified according to the Sequential Organ Failure Assessment (SOFA) (28) and Acute Physiology and Chronic Health Evaluation II (APACHE II) score (29), renal replacement therapy (RRT) during ECMO support, and duration of ECMO and mechanical ventilation (MV) use. Laboratory results, including blood tests, coagulation variables during the first 3 days after EMCO initiation and infection-causing pathogens during ECMO, were collected.
Outcomes
The primary outcome was the incidence rate of ECMO-related infection. The episodes per 1,000 ECMO days was used as the measurement for the incidence rate of ECMO-related infection (12,30). The secondary outcomes were mortality at hospital discharge and 90 days after ECMO initiation, successful weaning from ECMO, and length of ICU and hospital stay.
Statistical analysis
Continuous variables are summarized as the means ± standard deviations or medians [interquartile ranges (IQRs)]. Categorical variables are expressed as percentages and the number of patients. Student’s t-test or the Kolmogorov-Smirnov test was used for analysis of continuous variables between groups, and the χ2 test or Fisher’s exact test was used for categorical variables. Backward stepwise multivariable logistic regression was used to assess risk factors for ECMO-related infection and 90-day mortality. Potential risk factors with P≤0.10 in the univariable logistic regression analysis, including age, SOFA score, clinical characteristics and laboratory results, were retained. Adjusted odds ratios (ORs) and 95% confidence intervals (CIs) were calculated. The area under the receiver operating characteristic curve (AUROC) and Hosmer-Lemeshow test results are also reported. Kaplan-Meier estimates of the cumulative probability of survival in different groups of ECMO patients were generated, and the log-rank test was used. No imputation was performed for missing data. All analyses were performed using Stata 15.0 (College Station, TX, USA). A two-sided P value of 0.05 was considered statistically significant.
Results
Patient characteristics
From January 1, 2011 to December 31, 2019, 210 patients were supported with ECMO. A total of 174 subjects (median age, 48.5 years; 73.6% male) were included in the final analysis. A total of 36 patients were excluded for the following reasons: 12 patients were younger than 18 years old, the duration of ECMO in 23 patients was less than 48 hours, and in one patient, medical records were unavailable. The study flowchart is summarized in Figure 1.
The 174 included patients underwent ECMO for 1,670 days [8.2 (IQR, 5.9–11.1) days] and MV for 3,319 days [11.0 (IQR, 6.7–20.2) days] for a total of 3,627 ICU days [16.6 (IQR, 10.5–27.8) days]. Ninety-seven (55.7%) patients received veno-venous ECMO (VV-ECMO) support. The most frequent indication for ECMO was acute respiratory distress syndrome, which was diagnosed in 96 patients (55.2%). Forty-six patients (26.4%) developed ECMO-related infections (Table 1). The incidence rate of the first ECMO-related infection was 27.5 first episodes/1,000 ECMO days.
Table 1
Variables | Infected patients (n=46) | Uninfected patients (n=128) | P value |
---|---|---|---|
Age (years) | 52 [39, 59] | 47 [33, 56] | 0.67 |
Gender (male) | 35 (76.1) | 93 (72.7) | 0.65 |
Body mass index (kg/m2) | 24.2 [22.9, 27.4] | 24.2 [22.4, 27.8] | 0.82 |
Comorbidities | |||
Hypertension | 16 (34.8) | 47 (36.7) | 0.81 |
Cardiocerebrovascular disease | 0 | 4 (3.1) | 0.57 |
Chronic pulmonary disease | 3 (6.5) | 5 (3.9) | 0.46 |
Renal disease | 3 (6.5) | 5 (3.9) | 0.43 |
Diabetes mellitus | 7 (15.2) | 21 (16.4) | 0.85 |
Connective tissue disease | 3 (6.5) | 1 (0.8) | 0.057 |
Malignancy | 4 (8.7) | 5 (3.9) | 0.24 |
Immunocompromised status | 9 (19.6) | 10 (7.8) | 0.02 |
Broad-spectrum antibiotics before ECMO cannulation | 30 (65.2) | 44 (34.4) | <0.001 |
Reason for ECMO | |||
ARDS | 33 (71.7) | 63 (49.2) | 0.008 |
Asthma | 0 | 3 (2.3) | 0.56 |
Myocarditis | 4 (8.7) | 26 (20.3) | 0.10 |
Acute myocardial infarction | 3 (6.5) | 21 (16.4) | 0.13 |
Cardiac arrest | 3 (6.5) | 11 (8.6) | 0.76 |
PE | 0 (6.5) | 2 (1.6) | >0.99 |
Others | 3 (6.5) | 2 (1.6) | 0.11 |
Support type | 0.02 | ||
Veno-arterial | 14 (30.4) | 63 (49.2) | |
Veno-venous | 32 (69.6) | 65 (50.8) | |
Severity | |||
SOFA | 11 [9, 14] | 10 [8, 13] | 0.28 |
APACHE II | 25 [15, 27] | 22 [17, 30] | 0.84 |
LOS of hospital (days) | 28.2±20.7 | 22.0±15.6 | 0.03 |
LOS of ICU (days) | 26.5±20.0 | 19.5±14.3 | 0.01 |
ECMO duration (days) | 11.6 [8.1, 17.3] | 7.6 [5.6, 9.7] | <0.001 |
Organ support therapy | |||
MV duration (days) | 20.2 [12.6, 30.7] | 9.0 [5.8, 14.7] | <0.001 |
RRT during ECMO | 30 (65.2) | 53 (41.4) | 0.07 |
Successful weaning | 21 (45.7) | 91 (71.1) | 0.002 |
Hospital mortality | 36 (78.3) | 50 (39.1) | <0.001 |
90-day mortality | 40 (87.0) | 52 (40.6) | <0.001 |
Data are expressed as median [interquartile range], n (%), or mean ± standard deviation. ECMO, extracorporeal membrane oxygenation; ARDS, acute respiratory distress syndrome; PE, pulmonary embolism; SOFA, Sequential Organ Failure Assessment; APACHE II, Acute Physiology and Chronic Health Evaluation II; LOS, length of stay; ICU, intensive care unit; MV, mechanical ventilation; RRT, renal replacement therapy.
Sites and pathogens of ECMO-related infection
Of the 174 patients included, 24 (13.8%) developed VAPs, 21 (12.1%) developed BSIs, two of which were endocarditis, and 1 (0.6%) developed a UTI during their ECMO course, corresponding to 14.4 episodes/1,000 ECMO days, 6.0 episodes/1,000 ECMO days, and 12.6 episodes/1,000 ECMO days, respectively. VAP occurred at a median of 6.2 (IQR, 4.4, 8.0) days, BSI occurred at a median of 8.9 (IQR, 4.8, 11.6) days, and UTI occurred at 10 days after ECMO initiation (Table S2). Gram-negative bacteria were the most common causes of infection, especially Acinetobacter baumannii and Klebsiella pneumoniae, accounting for 62.5% of VAP cases and 57.1% of BSI cases. In addition, fungi are also important pathogens in ECMO-related infections. Candida was identified in two patients with VAP and two patients with BSI (Table S3). Nineteen (41.3%) bacteria were MDR, with 46 confirmed infections.
Comparison between patients with and without ECMO-related infection
There were 32 patients (69.6%) who received VV-ECMO among the 46 patients with ECMO-related infections. There was no significant difference in the SOFA or APACHE II score between the infected and noninfected patient groups. Compared with noninfected patients, infected patients had longer durations of ECMO [7.6 (IQR, 5.6, 9.7) vs. 11.6 (IQR, 8.1, 17.3) days, P<0.001] and MV [9.0 (IQR, 5.8, 14.7) vs. 20.2 (IQR, 12.6, 30.7) days, P<0.001] (Table 1).
Lymphocyte counts were significantly lower in infected patients than in noninfected patients during the first 3 days after ECMO initiation [day 1: 0.60 (IQR, 0.36, 0.87) vs. 0.92 (IQR, 0.49, 1.42) 109/L, P=0.005; day 2: 0.52 (IQR, 0.36, 1.09) vs. 0.86 (IQR, 0.60, 1.41) 109/L, P<0.001; day 3: 0.52 (IQR, 0.37, 0.72) vs. 0.89 (IQR, 0.55, 1.24) 109/L, P<0.001] (Table S4). A decrease in lymphocytes was more obvious in infected patients than in patients without ECMO-related infection [−0.13 (IQR, −0.46, 0.09) vs. −0.02 (IQR, −0.49, 0.39) 109/L, P=0.03]. Other laboratory variables measured during the first 3 days after ECMO initiation are shown in Table S5.
Secondary outcomes
A total of 174 patients were followed up for 90 days after ECMO initiation. The overall 90-day mortality was 52.9% (92 of 174). Noninfected patients had a greater rate of successful ECMO weaning (71.1% vs. 45.7%, P=0.002) (Table 1). Length of ICU (26.5±20.0 vs. 19.5±14.3 days, P=0.01) and hospital stay (28.2±20.7 vs. 22.0±15.6 days, P=0.03) were significantly longer in infected patients. Compared with noninfected patients, infected patients had higher hospital mortality (39.1% vs. 78.3%, P<0.001) and 90-day mortality (40.6% vs. 87.0%, P<0.001) (Figure S1).
Associations between dynamic decreases in lymphocyte counts and ECMO-related infections
A dynamic decrease in the lymphocyte count was defined as a decrease in the lymphocyte count on the third day compared with that on the first day of ECMO. Patients were divided into two groups according to the difference in lymphocyte counts between the first and third days of ECMO. We estimated the unadjusted cumulative probability of developing ECMO-related infection within 30 days after ECMO initiation by using a Kaplan-Meier curve with regard to the longest ECMO duration of 28 days. The cumulative probability of ECMO-related infection was significantly greater in patients with a decrease in lymphocytes than in those without a decrease in lymphocytes (log rank test P<0.001) (Figure 2).
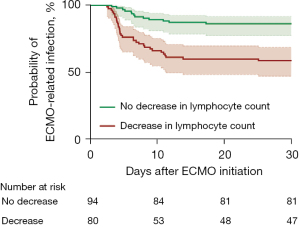
Univariate analysis revealed that ECMO support type [veno-arterial ECMO (VA-ECMO)], immunocompromised status, SOFA score, broad-spectrum antibiotics before ECMO cannulation, ECMO duration, MV duration, RRT during ECMO support, decrease in neutrophil count and decrease in lymphocytes were significantly associated with ECMO-related infection (Table S6). According to the multivariate logistic regression analysis, the factors independently associated with ECMO-related infection were decreased lymphocytes (adjusted OR =3.578, 95% CI: 2.175–4.906, P<0.001) and ECMO duration (adjusted OR =1.207, 95% CI: 1.096–1.330, P<0.001) [model: AUROC =0.851 (95% CI: 0.797, 0.902), Hosmer-Lemeshow test P=0.566] (Figure 3, Table S6, Figure S2).
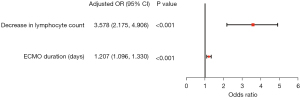
Risk factors for 90-day mortality
We assessed the risk factors for 90-day mortality. Univariate logistic regression analysis revealed that ECMO-related infection, age, SOFA score, diabetes mellitus status, dynamic decline in lymphocytes, ECMO duration, and RRT while on ECMO were significantly associated with 90-day mortality.
Of these factors, multivariable logistic regression analysis revealed that ECMO-related infection (OR =4.208, 95% CI: 3.197–6.524, P<0.001), age (OR =1.039, 95% CI: 1.011–1.068, P=0.006), SOFA score (OR =1.154, 95% CI: 1.021–1.305, P=0.02) and RRT while on ECMO (OR =4.632, 95% CI: 2.055–10.444, P<0.001) were significantly associated with 90-day mortality [model: AUROC =0.857 (95% CI: 0.802, 0.911), Hosmer-Lemeshow test P=0.273] (Table S7, Figure S3).
Subgroup analysis and sensitivity analysis
Subgroup analysis of the association between a decrease in lymphocytes and ECMO-related infection is shown in Figure 4. A correlation between a decrease in lymphocytes and ECMO-related infection remained for patients who received VV-ECMO or VA-ECMO (Tables S8-S11). After the exclusion of immunocompromised patients (n=19) from the analysis, the OR for the association between a decrease in lymphocytes and ECMO-related infection remained broadly consistent with the primary outcome (Table S12).
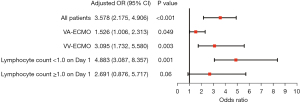
Discussion
In this retrospective study, we analyzed the incidence, microbial etiology and risk factors for ECMO-related infection in a large cohort of patients who received ECMO. Among patients who received ECMO support and who were at high risk of developing infectious complications, 46 (26.4%) developed ECMO-related infections. We clarified the relationship between a dynamic decrease in lymphocyte counts and an increased risk of ECMO-related infection. ECMO-related infection was an independent risk factor for 90-day mortality.
Gram-negative bacteria were the most common cause of infection in ECMO patients, accounting for up to 73.9% of ECMO-related infections. Acinetobacter baumannii and Klebsiella pneumoniae increased dramatically, accounting for 62.5% of VAP and 57.1% of BSI, respectively. Microorganisms associated with VAP and BSI in patients receiving ECMO support did not differ from classical epidemiology in non-ECMO patients, as published before. Xie et al. reported that Gram-negative bacteria were involved in 71.9% of VAP (31) and 59.7% of BSI (32). Tabah et al. also underscored this pattern by showing that approximately 60% of BSI cases were caused by Gram-negative bacteria (23). They also noted that Acinetobacter baumannii and Klebsiella pneumoniae were the most common pathogens. Similarly, Grasselli et al. reported that a higher incidence of infections was due to Gram-negative bacteria, which were involved in 63% of VAP cases and 53% of BSI cases (15). We also observed a high incidence of infections caused by MDR bacteria. Patients receiving ECMO support are often immunosuppressed (12,33), have a prolonged duration of MV and ICU stay (32) and are exposed to broad-spectrum antibiotics (15).
Identification of risk factors for ECMO-related infection is important but challenging. Our study showed that a dynamic decrease in lymphocyte count was significantly associated with a higher risk of ECMO-related infection. Patients with acute critical illness frequently display symptoms of systemic inflammatory response syndrome alongside compensatory anti-inflammatory response syndrome (33). Several studies on critically ill patients revealed increased release of anti-inflammatory cytokines, such as IL-10, and immune cell exhaustion (34,35). Hence, the immune response can exhibit a variety of patterns, including an anti-inflammatory response, global immunological depression, and a combination of anti-inflammatory and proinflammatory responses. Early immunological changes caused by VA-ECMO also contribute to innate and adaptive immune modifications that may increase the risk of infection (36,37). Secondary immune impairment, including acquired immunity, is compromised, and numerous studies have linked it to unfavorable outcomes. Lymphocytopenia is an independent predictor of 90-day mortality in non-immunocompromised patients with ICU-acquired pneumonia (38,39). Lymphopenia was associated with a 1.4-fold increased risk of hospitalization with infection and a 1.7-fold increased risk of infection-related death (40). Age and comorbidities were related to lymphopenia severity. The dynamic change in lymphocytes is an appropriate classification method due to the significant degree of heterogeneity among critically sick patients and therapeutic regimens. Adrie et al. noted that lymphopenia at ICU admission and its persistence on the third day were related to an increased risk of ICU-acquired infection, while only persistent lymphopenia predicted increased 28-day mortality (41). The tracking of a patient’s immunological state via lymphocyte trajectory is a practical method (42).
Patients who developed an infection during ECMO had a significantly longer duration of ECMO. The association between the risk of nosocomial infections and ECMO duration has been reported in many studies (9,43-45). The prevalence of nosocomial infections in patients who received ECMO for less than 1 week, 8 to 14 days, or more than 2 weeks was 6.1%, 15.7%, and 30.3%, respectively (46). Whether infection complications cause prolonged ECMO support or whether longer ECMO support increases the probability of infection complications is still under debate. These two elements were mutually causative. The risk of infection complications increased as ECMO support was prolonged; this, in turn, led to a longer period of ECMO support. In our study, patients who were infected experienced approximately 1.5-fold more usual ECMO and almost twice the usual MV duration.
ECMO-related infection was associated with increased mortality rates. A study showed a significant association between the occurrence of infectious complications and the death rate, as the chance of death was twice as high in infected patients than in noninfected patients (15). Consistent with these findings, our study showed that ECMO infection was significantly associated with an increased risk of death after adjusting for age and SOFA score. Hypotension, impaired red-cell deformability, and microvascular thrombosis lead to reduced oxygen delivery, contributing to more organ dysfunction (47). The vascular endothelium may become dysfunctional due to inflammation, which is also associated with cell death and a loss of barrier integrity, leading to subcutaneous and body cavity edema (48). Moreover, oxidative stress and other mechanisms that damage mitochondria decrease cellular oxygen utilization. In addition, damaged mitochondria produce alarmins in the extracellular space, including mitochondrial DNA and formyl peptides, which can stimulate neutrophils and lead to increased tissue damage (49,50). Infection plays a crucial role in ECMO patients. Gram-negative bacteria have become the dominant species of nosocomial infection due to the extensive use of glycopeptides and vancomycin (43). Consistent with this, a total of 73.9% of the Gram-negative bacteria were involved in ECMO-related infections in our study. Acinetobacter baumannii and Klebsiella pneumoniae increased dramatically, accounting for 62.5% of VAP and 57.1% of BSI, respectively. The clinical management of patients supported by ECMO should be improved even further.
Early identification of ECMO-related infection is critically important for patient management. Close attention should be given to immune monitoring in patients receiving ECMO support.
Several limitations in our study should be noted when interpreting the results. First, the present study was carried out in a single center, which precludes the extrapolation of the results to the general population of ECMO patients. Prospective, multicenter studies are needed to evaluate the epidemiology of infectious complications in ECMO patients. Second, the sample size was small and may not have been sufficient to produce statistical power. Third, we studied a mixed population with different infectious complications (VAP and BSI), and a detailed analysis of every type of infection was not performed. Fourth, we observed that a decrease in lymphocytes is associated with an increased risk for infection, but there are no data on lymphocyte type or specific lymphocyte subgroup functions. Assessment of immune status requires more comprehensive indicators. Finally, we cannot exclude the possibility that the results of our multivariable logistic regression analysis may be biased by confounding factors that were not considered in this study.
Conclusions
A high incidence of ECMO-related infection was observed in critically ill patients supported with ECMO, which contributed to worse outcomes. A decrease in lymphocyte count was significantly associated with an increased risk of ECMO-related infection.
Acknowledgments
Funding: This work was supported, in part, by
Footnote
Reporting Checklist: The authors have completed the STROBE reporting checklist. Available at https://jtd.amegroups.com/article/view/10.21037/jtd-23-1912/rc
Data Sharing Statement: Available at https://jtd.amegroups.com/article/view/10.21037/jtd-23-1912/dss
Peer Review File: Available at https://jtd.amegroups.com/article/view/10.21037/jtd-23-1912/prf
Conflicts of Interest: All authors have completed the ICMJE uniform disclosure form (available at https://jtd.amegroups.com/article/view/10.21037/jtd-23-1912/coif). The authors have no conflicts of interest to declare.
Ethical Statement: The authors are accountable for all aspects of the work in ensuring that questions related to the accuracy or integrity of any part of the work are appropriately investigated and resolved. The study was conducted in accordance with the Declaration of Helsinki (as revised in 2013). All the data in this study were anonymized and provided with permission from the CDIC database. Approval from Zhongda Hospital, Southeast University, Nanjing, China, was obtained for this study (No. 2022ZDSYLL177-P01). Patient informed consent was waived due to the retrospective study design.
Open Access Statement: This is an Open Access article distributed in accordance with the Creative Commons Attribution-NonCommercial-NoDerivs 4.0 International License (CC BY-NC-ND 4.0), which permits the non-commercial replication and distribution of the article with the strict proviso that no changes or edits are made and the original work is properly cited (including links to both the formal publication through the relevant DOI and the license). See: https://creativecommons.org/licenses/by-nc-nd/4.0/.
References
- Becher PM, Schrage B, Sinning CR, et al. Venoarterial Extracorporeal Membrane Oxygenation for Cardiopulmonary Support. Circulation 2018;138:2298-300. [Crossref] [PubMed]
- Combes A, Hajage D, Capellier G, et al. Extracorporeal Membrane Oxygenation for Severe Acute Respiratory Distress Syndrome. N Engl J Med 2018;378:1965-75. [Crossref] [PubMed]
- Barbaro RP, MacLaren G, Boonstra PS, et al. Extracorporeal membrane oxygenation for COVID-19: evolving outcomes from the international Extracorporeal Life Support Organization Registry. Lancet 2021;398:1230-8. [Crossref] [PubMed]
- Sauer CM, Yuh DD, Bonde P. Extracorporeal membrane oxygenation use has increased by 433% in adults in the United States from 2006 to 2011. ASAIO J 2015;61:31-6. [Crossref] [PubMed]
- Karagiannidis C, Brodie D, Strassmann S, et al. Extracorporeal membrane oxygenation: evolving epidemiology and mortality. Intensive Care Med 2016;42:889-96. [Crossref] [PubMed]
- Shah M, Patnaik S, Patel B, et al. Trends in mechanical circulatory support use and hospital mortality among patients with acute myocardial infarction and non-infarction related cardiogenic shock in the United States. Clin Res Cardiol 2018;107:287-303. [Crossref] [PubMed]
- Ling RR, Ramanathan K, Poon WH, et al. Venoarterial extracorporeal membrane oxygenation as mechanical circulatory support in adult septic shock: a systematic review and meta-analysis with individual participant data meta-regression analysis. Crit Care 2021;25:246. [Crossref] [PubMed]
- Ostadal P, Rokyta R, Karasek J, et al. Extracorporeal Membrane Oxygenation in the Therapy of Cardiogenic Shock: Results of the ECMO-CS Randomized Clinical Trial. Circulation 2023;147:454-64. [Crossref] [PubMed]
- Juthani BK, Macfarlan J, Wu J, et al. Incidence of nosocomial infections in adult patients undergoing extracorporeal membrane oxygenation. Heart Lung 2018;47:626-30. [Crossref] [PubMed]
- Abrams D, Grasselli G, Schmidt M, et al. ECLS-associated infections in adults: what we know and what we don't yet know. Intensive Care Med 2020;46:182-91. [Crossref] [PubMed]
- Meyer DM, Jessen ME, Eberhart RC. Neonatal extracorporeal membrane oxygenation complicated by sepsis. Extracorporeal Life Support Organization. Ann Thorac Surg 1995;59:975-80. [Crossref] [PubMed]
- Schmidt M, Bréchot N, Hariri S, et al. Nosocomial infections in adult cardiogenic shock patients supported by venoarterial extracorporeal membrane oxygenation. Clin Infect Dis 2012;55:1633-41. [Crossref] [PubMed]
- Bouglé A, Bombled C, Margetis D, et al. Ventilator-associated pneumonia in patients assisted by veno-arterial extracorporeal membrane oxygenation support: Epidemiology and risk factors of treatment failure. PLoS One 2018;13:e0194976. [Crossref] [PubMed]
- Xu W, Fu Y, Yao Y, et al. Nosocomial Infections in Nonsurgical Patients Undergoing Extracorporeal Membrane Oxygenation: A Retrospective Analysis in a Chinese Hospital. Infect Drug Resist 2022;15:4117-26. [Crossref] [PubMed]
- Grasselli G, Scaravilli V, Di Bella S, et al. Nosocomial Infections During Extracorporeal Membrane Oxygenation: Incidence, Etiology, and Impact on Patients' Outcome. Crit Care Med 2017;45:1726-33. [Crossref] [PubMed]
- Cilloniz C, Peroni HJ, Gabarrús A, et al. Lymphopenia Is Associated With Poor Outcomes of Patients With Community-Acquired Pneumonia and Sepsis. Open Forum Infect Dis 2021;8:ofab169. [Crossref] [PubMed]
- Ménétrier-Caux C, Ray-Coquard I, Blay JY, et al. Lymphopenia in Cancer Patients and its Effects on Response to Immunotherapy: an opportunity for combination with Cytokines? J Immunother Cancer 2019;7:85. [Crossref] [PubMed]
- Qin L, Jing X, Qiu Z, et al. Aging of immune system: Immune signature from peripheral blood lymphocyte subsets in 1068 healthy adults. Aging (Albany NY) 2016;8:848-59. [Crossref] [PubMed]
- von Elm E, Altman DG, Egger M, et al. The Strengthening the Reporting of Observational Studies in Epidemiology (STROBE) statement: guidelines for reporting observational studies. J Clin Epidemiol 2008;61:344-9. [Crossref] [PubMed]
- Pneumonia (Ventilator-associated [VAP] and non-ventilator- associated Pneumonia [PNEU]) Event. 2022. Available online: https://www.cdc.gov/nhsn/pdfs/pscmanual/6pscvapcurrent.pdf
- Kalil AC, Metersky ML, Klompas M, et al. Management of Adults With Hospital-acquired and Ventilator-associated Pneumonia: 2016 Clinical Practice Guidelines by the Infectious Diseases Society of America and the American Thoracic Society. Clin Infect Dis 2016;63:e61-e111. [Crossref] [PubMed]
- American Thoracic Society, Infectious Diseases Society of America. Guidelines for the management of adults with hospital-acquired, ventilator-associated, and healthcare-associated pneumonia. Am J Respir Crit Care Med 2005;171:388-416. [Crossref] [PubMed]
- Tabah A, Buetti N, Staiquly Q, et al. Epidemiology and outcomes of hospital-acquired bloodstream infections in intensive care unit patients: the EUROBACT-2 international cohort study. Intensive Care Med 2023;49:178-90. [Crossref] [PubMed]
- Clarke K, Hall CL, Wiley Z, et al. Catheter-Associated Urinary Tract Infections in Adults: Diagnosis, Treatment, and Prevention. J Hosp Med 2020;15:552-6. [Crossref] [PubMed]
- Hooton TM, Bradley SF, Cardenas DD, et al. Diagnosis, prevention, and treatment of catheter-associated urinary tract infection in adults: 2009 International Clinical Practice Guidelines from the Infectious Diseases Society of America. Clin Infect Dis 2010;50:625-63. [Crossref] [PubMed]
- Magiorakos AP, Srinivasan A, Carey RB, et al. Multidrug-resistant, extensively drug-resistant and pandrug-resistant bacteria: an international expert proposal for interim standard definitions for acquired resistance. Clin Microbiol Infect 2012;18:268-81. [Crossref] [PubMed]
- Schmidt M, Schellongowski P, Patroniti N, et al. Six-Month Outcome of Immunocompromised Patients with Severe Acute Respiratory Distress Syndrome Rescued by Extracorporeal Membrane Oxygenation. An International Multicenter Retrospective Study. Am J Respir Crit Care Med 2018;197:1297-307. [Crossref] [PubMed]
- Lambden S, Laterre PF, Levy MM, et al. The SOFA score-development, utility and challenges of accurate assessment in clinical trials. Crit Care 2019;23:374. [Crossref] [PubMed]
- Knaus WA, Draper EA, Wagner DP, et al. APACHE II: a severity of disease classification system. Crit Care Med 1985;13:818-29. [Crossref] [PubMed]
- Allou N, Lo Pinto H, Persichini R, et al. Cannula-Related Infection in Patients Supported by Peripheral ECMO: Clinical and Microbiological Characteristics. ASAIO J 2019;65:180-6. [Crossref] [PubMed]
- Xie J, Yang Y, Huang Y, et al. The Current Epidemiological Landscape of Ventilator-associated Pneumonia in the Intensive Care Unit: A Multicenter Prospective Observational Study in China. Clin Infect Dis 2018;67:S153-61. [Crossref] [PubMed]
- Xie J, Li S, Xue M, et al. Early- and Late-Onset Bloodstream Infections in the Intensive Care Unit: A Retrospective 5-Year Study of Patients at a University Hospital in China. J Infect Dis 2020;221:S184-92. [Crossref] [PubMed]
- van der Poll T, Shankar-Hari M, Wiersinga WJ. The immunology of sepsis. Immunity 2021;54:2450-64. [Crossref] [PubMed]
- Yan L, Chen Y, Han Y, et al. Role of CD8(+) T cell exhaustion in the progression and prognosis of acute respiratory distress syndrome induced by sepsis: a prospective observational study. BMC Emerg Med 2022;22:182. [Crossref] [PubMed]
- Guinault D, Nicolau-Travers ML, Silva S, et al. Expression of Exhaustion Markers on CD8+ T-Cell Patterns Predict Outcomes in Septic Patients Admitted to the ICU. Crit Care Med 2021;49:1513-23. [Crossref] [PubMed]
- Frerou A, Lesouhaitier M, Gregoire M, et al. Venoarterial extracorporeal membrane oxygenation induces early immune alterations. Crit Care 2021;25:9. [Crossref] [PubMed]
- Cho HJ, Kayumov M, Kim D, et al. Acute Immune Response in Venoarterial and Venovenous Extracorporeal Membrane Oxygenation Models of Rats. ASAIO J 2021;67:546-53. [Crossref] [PubMed]
- Ceccato A, Panagiotarakou M, Ranzani OT, et al. Lymphocytopenia as a Predictor of Mortality in Patients with ICU-Acquired Pneumonia. J Clin Med 2019;8:843. [Crossref] [PubMed]
- Campbell B, Budreau D, Williams-Perez S, et al. Admission Lymphopenia Predicts Infectious Complications and Mortality in Traumatic Brain Injury Victims. Shock 2022;57:189-98. [Crossref] [PubMed]
- Warny M, Helby J, Nordestgaard BG, et al. Lymphopenia and risk of infection and infection-related death in 98,344 individuals from a prospective Danish population-based study. PLoS Med 2018;15:e1002685. [Crossref] [PubMed]
- Adrie C, Lugosi M, Sonneville R, et al. Persistent lymphopenia is a risk factor for ICU-acquired infections and for death in ICU patients with sustained hypotension at admission. Ann Intensive Care 2017;7:30. [Crossref] [PubMed]
- Pei F, Song W, Wang L, et al. Lymphocyte trajectories are associated with prognosis in critically ill patients: A convenient way to monitor immune status. Front Med (Lausanne) 2022;9:953103. [Crossref] [PubMed]
- Biffi S, Di Bella S, Scaravilli V, et al. Infections during extracorporeal membrane oxygenation: epidemiology, risk factors, pathogenesis and prevention. Int J Antimicrob Agents 2017;50:9-16. [Crossref] [PubMed]
- Haneke F, Schildhauer TA, Schlebes AD, et al. Infections and Extracorporeal Membrane Oxygenation: Incidence, Therapy, and Outcome. ASAIO J 2016;62:80-6. [Crossref] [PubMed]
- Fux T, Holm M, Corbascio M, et al. Venoarterial extracorporeal membrane oxygenation for postcardiotomy shock: Risk factors for mortality. J Thorac Cardiovasc Surg 2018;156:1894-1902.e3. [Crossref] [PubMed]
- Bizzarro MJ, Conrad SA, Kaufman DA, et al. Infections acquired during extracorporeal membrane oxygenation in neonates, children, and adults. Pediatr Crit Care Med 2011;12:277-81. [Crossref] [PubMed]
- Angus DC, van der Poll T. Severe sepsis and septic shock. N Engl J Med 2013;369:840-51. [Crossref] [PubMed]
- Opal SM, van der Poll T. Endothelial barrier dysfunction in septic shock. J Intern Med 2015;277:277-93. [Crossref] [PubMed]
- Zhang Q, Raoof M, Chen Y, et al. Circulating mitochondrial DAMPs cause inflammatory responses to injury. Nature 2010;464:104-7. [Crossref] [PubMed]
- Mantzarlis K, Tsolaki V, Zakynthinos E. Role of Oxidative Stress and Mitochondrial Dysfunction in Sepsis and Potential Therapies. Oxid Med Cell Longev 2017;2017:5985209. [Crossref] [PubMed]