Complex chest wall reconstruction after failure: a literature review
Introduction
Primary osseous and soft-tissue chest wall tumors (osteo- and chondrosarcomas; desmoid tumors, schwannomas, etc.), metastatic lesions of the chest wall, locally advanced breast cancers, congenital defects, and chest wall osteoradionecrosis often require extensive full-thickness wide local excisions and complex reconstruction to re-provide stabilization and good biomechanical results, reducing skeletal instability and the risk of respiratory impairment and infectious complications (1). The surgical management of large neoplasms involving the skeletal chest wall, pleura or even the lungs includes three phases: (I) resection of the involved tissue with adequate surgical margins; (II) reconstruction of the skeletal chest wall; and (III) resurfacing the soft tissue defect (2).
Managing oncologic chest wall resection and reconstruction is challenging for all medical professionals involved, like thoracic and plastic surgeons, medical and radiation oncologists. It should always be approached through multidisciplinary team (MDT) discussion. Chest wall reconstruction could be performed using various techniques and tools including meshes, methyl-methacrylate (MMA) prosthesis, titanium implants and custom-made 3D printed anatomical ribs and implants based on the defect to cover, to minimize the loss in anatomical shape and physiological function of the chest wall.
It is important to promptly complete bony reconstruction of the chest wall in order to restore its integrity and stability. Failure to do so can lead to chest wall weakening, abnormal breathing, exacerbated respiratory problems, and circulatory disorders. There are several key considerations that should be taken into account for chest wall reconstruction. Firstly, chest wall repair materials must possess adequate rigidity to safeguard the chest and upper abdominal organs. Secondly, the integrity of respiratory function should be maintained after chest wall reconstruction. Thirdly, the reconstructed thorax should enable full range of motion and strength in the upper limbs and shoulder joints. Fourthly, chest wall reconstruction materials should exhibit high safety, implantability, non-carcinogenic properties, promote fibrous tissue growth, and resist infection. Lastly, it should not interfere with chest X-ray examinations and allow for convenient patient follow-up (3). Chest wall reconstructive surgery is affected by a high complication rate, especially when the defect is large, ranging from 16% to 69%, including 27% respiratory morbidity (4-6). Complete failure of complex chest wall reconstructions requiring reintervention may occur because of recurrent tumor, type of prosthesis adopted, defect extension (>3 ribs or >100 cm2, sternal resections), or local conditions (e.g., irradiation or infection) (1). Each situation requires a personalized and tailored approach, with no existing guidelines for correct management. Literature only includes case reports or series illustrating case-by-case approaches. This review aims to collect and summarize experiences related to failed chest wall reconstruction procedures, identify common causes of failure, and highlight surgical and oncological principles that can aid in complex chest wall reconstruction post-failure. We present this article in accordance with the Narrative Review reporting checklist (available at https://jtd.amegroups.com/article/view/10.21037/jtd-23-1431/rc).
Methods
A literature review was performed through PubMed, Scopus, ScienceDirect, and Google Scholar to identify studies published after 1970 to August 2023 evaluating the surgical management of complex chest wall reconstruction after failure. The retrieval terms were “reconstruction AND chest wall”, “chest wall failure”, “complex reconstruction AND chest wall”, and “prosthesis AND chest wall”. Once the abstracts of potentially relevant studies were scrutinized, each study was independently evaluated by three co-authors (G.M., D.B., G.M.) for inclusion or exclusion from this analysis. The search strategy has been summarized in Table 1.
Table 1
Items | Specification |
---|---|
Date of search | May–August 2023 |
Databases and other sources searched | PubMed, Google Scholar, ScienceDirect and Scopus |
Search terms used | “reconstruction AND chest wall”, “chest wall failure”, “complex reconstruction AND chest wall”, and “prosthesis AND chest wall” |
Timeframe | 1970 to August 2023 |
Inclusion and exclusion criteria | Inclusion: full manuscripts published in English including all study designs (reviews, case reports, original articles) |
Exclusion: abstracts, preprints, and letters to editors | |
Selection process | Three co-authors (G.M., D.B., G.M.) independently read all the publication titles to identify only the most relevant; following a reading of the abstracts of these publications only the most relevant full texts were included in this review. Disagreement on included articles was settled by consensus among these investigators |
Failure because of recurrent tumor
Local recurrence after primary surgical treatment mainly depends on histology and on the achievement of adequate surgical margins. Tumor-free margins are critical because 86% of non-radical resections result in recurrence (7). In the case of metastases or benign lesions, 2 cm of margins may be sufficient, while at least 4 cm of margins should be achieved in the case of primary chest wall malignant neoplasms (sarcoma) (7). Desmoid tumors, although considered benign soft-tissue tumors of the chest wall, are locally invasive and have a high rate of recurrence. Abbas et al. (8) reported a local relapse of 89% with positive margins versus 18% with negative margins; therefore, a clear margin at least of 5 cm should be warranted. King et al. (9) found out that the 5-year survival rate of patients with malignant chest wall neoplasms with a 4 cm resection was significantly higher when compared with those with a 2 cm resection margin (56% vs. 29%). McMillan et al. (10) in their study on 192 patients who underwent resection for chest wall soft tissue sarcoma, reported a 23% of local or distant recurrence, with 5- and 10-year survival rates of 73% and 61% respectively.
Surgical management of recurrent chest wall malignancies after primary resection and reconstruction is challenging and rarely reported in the literature, consisting only of anecdotic cases. Endara et al. (11) reported the peculiar case of a 31-year-old patient affected by synovial sarcoma who underwent tumor resection en bloc with the left ninth rib by thoracotomy and who presented after two years with a magnetic resonance imaging (MRI) evidence of tumor recurrence, with involvement of the left posterior chest wall with extension to the diaphragm, lung and erosion of the posterior ninth costal arch and left transverse process of the T9 vertebral body. After a multidisciplinary discussion, they decided to perform complete resection of the tumor mass with the cooperation of the thoracic surgeon for the resection of the tumor en bloc with the chest wall, the diaphragm and the lung and their reconstruction and the spine surgeon for T8–T9 hemi laminectomy, spinal cord decompression, block pedicle osteotomy T8–T9, ligation of the emerging nerve roots, arthrodesis and posterior instrumentation T6–T12. Although anecdotic, this complex chest wall demolition and reconstruction highlights the paramount importance of an MDT discussion at first and multidisciplinary surgical management at second (12). It is reported that the successful rate of re-excision after MDT discussion is 81.2%, higher than what is reported in the literature for not-discussed cases, highlighting the key role of MDT in the decision to perform re-excision after unplanned resection (13).
Wouters et al. (7) performed a retrospective review of patients with either primary (83 patients) or recurrent (44 patients) chest wall sarcomas treated with radical surgery. Surgical outcomes were comparable between the two groups; particularly a median number of 2.4 ribs were resected in the recurrence group, like those resected in the primary sarcoma group; postoperative morbidity and the need for reintervention were similar in the two groups, median hospital stay was 3 days longer for patients in the recurrence group. On the other hand, oncologic outcomes were different between the two groups, in fact only 25% of patients with resection for recurrent sarcoma remained tumor free, as compared with 51% of the patients who underwent a primary resection.
The need for a larger resection in case of recurrent tumors raises the problem regarding the reconstruction that usually is challenging for both bone and soft tissue and myo-cutaneous area being the best muscles and tissues usually already utilized during previous reconstructive surgery (Figures 1,2).
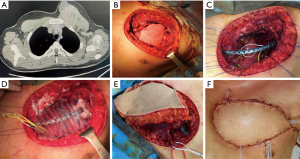
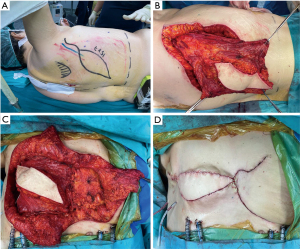
Failure of chest wall reconstruction because of recurrence of the tumor is non-negligible; because of the complexity of a reintervention and of the need to be oncological effective, the best approach is MDT discussion and adequate pre-surgical planning including all the surgical figures involved. Because of the technical difficulty of these reinterventions, these procedures should be carried out only in high-volume and selected centers.
Failure because of the type of prosthesis
Chest wall reconstruction of large defects has been linked with surgical morbidity and failure, including both minor (seromas, etc.) and major (respiratory impairment, infections, etc.) complications needing sometimes a reintervention (14). The choice of the right prosthetic material and surgical technique impacts the result the most. Few reports are available in literature about implant failure, and all data are anecdotic and derive from small case series. Recently, new biomaterials such as cryopreserved homograft, acellular collagen matrices, and titanium plates have been introduced in clinical practice. These new materials are reported to have advantages over conventional ones in terms of biocompatibility and easy incorporation into the host even in compromised local conditions. In fact, they can also be used in infected or heavily irradiated areas without the need for removal, should a suppurative complication develop. Table 2 summarizes the largest experiences reported in literature about the use of prosthetic materials for chest wall reconstruction and the percentage of their failure because of different reasons.
Table 2
Author | Year | No. patients | Type of prosthesis | % failure | Type of failure (%) | Management of failure (%) | |||||
---|---|---|---|---|---|---|---|---|---|---|---|
Rupture | Displacement | Infection | Recurrence | Reintervention | Conservative | ||||||
Osteosynthesis | |||||||||||
Berthet et al. (15) | 2015 | 54: 29 (tumor); 25 (trauma) | Stratos or the Matrix Fixation | 44.4 | 37.0 | 7.4 | − | − | NA | NA | |
De Palma et al. (16) | 2016 | 27: 11 (tumor); 16 (trauma) | Synthes® Sternal Fixation/MatrixRIB | 11.1 | 7.4 | 3.7 | − | − | 7.4 | 3.7 | |
Clermidy et al. (17) | 2022 | 68: 68 (tumor) | Thorib® titanium bars or Trionyx® sternal plate | 14.7 | 5.9 | − | 8.8 | − | 1.4 | 4.5 | |
Wong et al. (18) | 2022 | 22: 19 (tumor); 3 (trauma) |
MatrixRIB/prosthetic patch (Gore® Dualmesh® or Permacol™) | 22.7 | 4.5 | 4.5 | 18.2 | 9.0 | 22.7 | − | |
Synthetic and biological materials | |||||||||||
Bergovec et al. (19) | 2022 | 26: 26 (tumor) | Polypropylene, porcine dermal collagen mesh +/− titanium rib plates | 15.4 | 15.4 | − | − | − | NA | NA | |
Petrella et al. (20) | 2018 | 166: 166 (tumor) | polypropylene double mesh + MMA | 6 | − | − | 4.8 | 1.2 | 6 | − | |
Weyant et al. (6) | 2006 | 209: 209 (tumor) | MMA and PTFE prostheses | 4.3 | 0.5 | − | 3.8 | 4.3 | − | ||
Rocco (21) | 2011 | 86: 86 (tumor) | PTFE, MMA and biomaterials | 10.5 | − | − | 8.2 | 3.2 | 10.5 | − |
MMA, methyl-methacrylate; PTFE, polytetrafluoroethylene prostheses; NA, not available.
Osteosynthesis systems
For a long time, titanium has been chosen as the best prosthetic material for large defects, given its strength, corrosion resistance and biocompatibility and several titanium plating systems have been commercialized (Figure 3). Aside from these advantages, these prostheses must be tailored to each patient and manipulation itself may lead to microfractures and full fractures which may lead to reoperation (Figure 4) (22). The successful implantation of a prosthetic rib requires thorough preoperative planning to prevent complications. The imaging should be carefully studied to estimate the size of the post-resection defect (22). The size of the defect must be evaluated first to determine the optimal prosthesis and the most suitable reconstruction. The length of the plate determines the maximum rib-rib defect that can be bridged. The positioning of the ribs should be assessed secondly. Most of the failures occur in anterior chest wall implants, probably because of the larger chest movement during respiration and repeated stress. Another complication is prosthesis screw loosening, which can happen because of the mismatch between the screw length and the measured rib thickness. The system’s parallel gauge measures the thickest aspect of the rib. The surgeon must ensure that the drilling, plates, and screws are placed over this thickest aspect of the rib to correspond to the measured screw length and prevent screw protrusion and loosening (22).
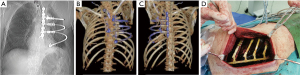
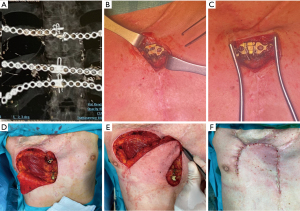
Frequently, implant failures are asymptomatic [in 87% of cases (15)] and they are diagnosed especially during follow-up for oncologic reasons, while a radiologic follow-up is not so systematically performed in patients submitted to osteosynthesis for traumas, and this could explain the potential underestimation of this topic and the paucity of its description in literature. Berthet et al. (15) analyzed a series of 54 patients enrolled from 2009 to 2013 who underwent chest wall reconstruction through titanium implants, for either traumatic or oncologic reasons and they found 44.4% of implant failures, all occurred within 14 months after surgery. Interestingly, Berthet reported that neither the initial pathologic condition nor the type of implant and the extent of the defect did impact the risk of failure, whereas the anterior location of the defect and the presence of three or more implants did. De Palma et al. (16), in their study of 27 consecutive patients treated with titanium implants for either benign or malignant chest wall diseases, observed failure in 11.1% of cases. Particularly, plate rupture was described in two cases (one patient treated for anterior flail chest and one patient for rib chondrosarcoma) and dislodgment of the pin of a plate in another one (after sternectomy for chondrosarcoma). In the first case, removal of the plates was performed without replacement because a complete and stable bone callus was detected; in the second case, the one of the rib chondrosarcomas, the ruptured titanium plate caused no pain at all, thus they decided for a radiological follow-up; the third case, was treated with surgery for reposition the pin. Clermidy et al. (17) in their recent experience on a series of 87 patients treated with titanium implants only for chest wall tumors, described implant failure in four patients (6%); reintervention was needed only in one case almost 4 years after the initial surgery, while the three remaining cases were managed conservatively with radiological follow-up because of the age of the patient and the asymptomatic fracture.
Titanium has been widely used in recent years as a new material for reconstruction because both of its biocompatibility and strength and they were designed to provide support to the underlying bone during the healing process, instead of replacing it. Failure of reconstruction tends to occur in anterior chest wall implants probably because of the larger chest movement during respiration and repeated stress. Surgical removal is often required following implant fracture because of discomfort and pain, and risk of implant migration injury, but conservative management and radiological follow-up have also been proposed as a successful choice, in case of the patients’ good clinical conditions.
Synthetic materials
Synthetic meshes, both flexible (e.g., polypropylene, polytetrafluoroethylene, polyglactin) and rigid (e.g., MMA), are available for chest wall reconstructions. Flexible meshes distribute tension along the edges of medium-sized defects, while rigid prostheses provide stability and rigidity for larger defects. Petrella et al. (20) performed a retrospective review of 166 patients who underwent chest wall resection for sarcoma and reconstruction by a rigid prosthesis (polypropylene double mesh + methyl methacrylate); among them, 6% of patients required prosthesis removal because of infection (4.8%) or neoplastic recurrence (1.2%). In case of infection, they first attempted vacuum-assisted closure to minimize the micro-bacterial load before surgical explant, then the prosthesis was removed. In all cases the meshes were removed at least 6 to 8 weeks after the first implant, so that a thick fibrous layer rigid enough to furnish chest wall stability was formed, not requiring a new rigid prosthesis but at least a soft one; while in only one case of an extended re-do chest wall resection for local recurrence, a new rigid prosthesis was required.
Weyant et al. (6) performed a retrospective analysis on 209 patients who underwent chest wall resection and reconstruction with either rigid Marlex mesh (Bard, Cranston, RI, USA) MMA and non-rigid polytetrafluoroethylene prostheses (PTFE). Nine patients (4.3%) experienced prosthesis failure due to wound infections (3.8%) and respiratory failure (0.5%). One patient, in fact, who had a PTFE prosthesis, developed chest wall instability, and required early removal and replacement with an MMA prosthesis in the postoperative period.
A recent review of the literature focused on the outcomes in chest wall reconstruction using MMA prosthesis including 562 patients reported a complication rate of 13.7%, with infection confirming the most common (5.6%). Interestingly, prosthesis dislocation was reported in 1.2% of the patients (23).
Managing the complications of chest wall reconstruction with synthetic materials is more challenging, since they are more prone to others to develop infections and, when it happens, the prosthesis has to be removed without possibility of a conservative treatment (Figure 5). It is important to avoid the use of synthetic materials in primary chest wall infections.
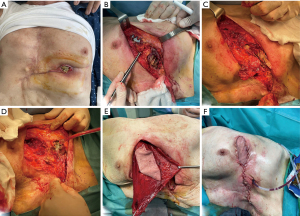
Biological meshes
Biological meshes have advantages such as easy manipulation, uniform stretching, and easy suturing. They provide a scaffold for connective tissue growth, especially biologic meshes that allow regeneration and revascularization. Disadvantages include less protection for intrathoracic organs compared to rigid prostheses, cosmetic results, and higher costs.
Shah et al. (24) presented a case of a patient who underwent triple-vessel coronary artery bypass grafting and then developed a sternal infection and the rupture of the right ventricle which required an open chest and the subsequent placement of a Strattice Reconstructive Tissue Matrix (LifeCell Corporation; Branchburg, NJ, USA) over the heart and underneath the rib cage to minimize contact of the heart with the sternal edges. Seven years later, the patient presented with a severe recurrent coronary disease requiring a reoperation. After having excised the old sternal scar, the mesh was exposed, intact and completely incorporated into the surrounding tissues. Only adhesions between the mesh and right heart were found, but they could be easily managed, and a repeat coronary artery bypass grafting was completed. No need for a new reconstruction was needed, but the divided mesh was left in place.
Differently from synthetic ones, biological materials have the advantage of being biocompatible and easily incorporated into the surrounding tissues. Biological mesh represents a valuable option in chest wall reconstruction, especially in infected or high-risk-of-infection surgical sites.
Failure because of infection
Local morbidity after chest wall reconstruction varies between 4.6% and 23%, yielding removal of the prosthetic material in 6.4% of the cases (21). Rigid reconstruction of the chest wall is affected by infections in 0 to 22% of cases (25,26) and in 10% after sternum osteosynthesis (27). Redo-surgery after failure of previous reconstruction for infection or heavy irradiation may be challenging. It is important to avoid the use of synthetic materials in primary chest wall infections, but in secondary infections after previous reconstruction and in the presence of an infected prosthesis, the removal of the infected hardware is crucial. However, it may lead to severe consequences such as chronic pain, lung function impairment, and chest-wall deformity in the case of large defects. Berthet et al. (28) reviewed four cases of secondary deep chest wall infections highlighting the available management options for these situations. The surgical procedure involves removing all synthetic material implanted during initial reconstruction, except for titanium implants. The procedure includes radical debridement of the thoracic wall, complete excision of tissue necrosis, meticulous pulse-jet lavage, and decortication in some cases which may lead to a larger parietal defect than the primary one (29).
Haslam et al. (30) presented a case of a 66-year-old woman with a radiation-induced chest ulcer after breast cancer treatment. Conservative treatment failed, leading to chest wall resection and reconstruction with biologic Strattice porcine tissue matrix (LifeCell Corporation, One Millenium Way, Branchburg, NJ, USA) and a BioBridge prosthesis (Acute Innovations, Hillsboro, OR, USA). Six months later, a fragment of the BioBridge plate was found in the wound, and the prostheses were fractured into seven fragments that were completely explanted. Wound closure was achieved with myocutaneous latissimus dorsi (LD) flap without additional skeletal reconstruction. Rocco et al. (31) described a series of 86 patients who underwent chest wall resection and reconstruction for tumor and in nine cases (10.5%) patients underwent reintervention because local wound complications. In 4 of 7 patients with local infectious wound complications (4.6% of 86 patients), the prosthesis placed at the primary operation had to be removed. Moreover, all patients with local sepsis were subjected to vacuum assisted closure (VAC) treatment as a “bridge” for chest wall reconstruction, since it facilitates myocutaneous transfer preparation and minimizes the area that needs to be covered.
Finally, recent evidence suggests that, in case of surgical case infection, the resorbable features of the biological patches do not require their removal even if infected (32,33).
Infections and radiation-induced chest ulcer after previous surgical treatment may be challenging situations. The surgical procedure should involve debridement of the thoracic wall, excision of tissue necrosis, pulse-jet lavage, and removal of the prosthesis. After this, VAC-therapy could be considered as a bridge for chest wall re-reconstruction, minimizing the area that needs to be covered.
Post-sternotomy complications
Complications from sternotomy wounds are a major issue in heart surgery, ranging from 0.5% to 10% and when sternal instability/dehiscence occurs, VAC therapy followed by Robicsek closure represents the most used approach (34,35). Robicsek failure and deep sternal wound infections require wire removal and sternal debridement, which result in massive bone loss. In some cases, reconstructive surgery can be difficult due to the downsides of common synthetic materials. These downsides include excessive rigidity, the risk of erosion of adjacent structures, the risk of infection, insufficient strength, rupture, migration, and the inability to incorporate into host tissue. Synthetic materials should be avoided in cases of active infection, such as sternal wound infections. Tailored techniques should be used for each individual case, resulting in only a few anecdotal cases reported in the literature. Dell’Amore et al. (36) have recently described two different surgical approaches for treating a massive sternal loss after a failed Robicsek repair (37). In the first case, a 71-year-old man with post-sternotomy mediastinitis due to Pseudomonas aeruginosa and the complete sternal defect was treated. During a re-operation, the cage was removed, and complete dehiscence of the sternum and bone loss was identified. After 15 days of VAC therapy, they performed a resection of the residual edges of the sternum, cutting 1–2 cm into healthy tissue. Due to extensive bone loss and prior infection, a cryopreserved tibial bone allograft was used to reconstruct the anterior chest wall. To stabilize the chest wall, four transversal titanium plates were implanted (Synthes®, Solothurn, Switzerland) and bilateral release of the pectoral muscle flaps was performed following reconstruction. The second case was that of a 59-year-old male patient with dilated cardiomyopathy who underwent orthotopic heart transplantation. He was reoperated for bleeding after 4 days and two weeks later, the Robicsek technique was used to treat sternal dehiscence. During the hospitalization, a wound infection with sternal instability was discovered and Klebsiella pneumoniae was isolated. VAC therapy was applied for 14 days, followed by mobilization and cutting of the sternal bone edges until visible bleeding from the bone marrow. The sternal bone had multiple fractures and a thin, fragile rim of tissue that did not guarantee stability, making it impossible to reconstruct with metal steel wires or a titanium plate alone. To cover the anterior chest wall defect, they used a titanium mesh (RPS.THORAX - MDF Medica S.r.l., Italy) placed above the sternal residue and fixed bilaterally to the rib heads with four steel wires. In a previous case report, Dell’Amore et al. (38) described a 68-year-old male who underwent coronary artery bypass grafting. Due to a postoperative Staphylococcus aureus infection, the patient needed surgical revision and sternal closure using the Robicsek procedure. However, after two months, the patient experienced complete sternal dehiscence and an attempt at sternal fixation using Ethibond sutures (Ethicon Inc., Somerville, NJ, USA) was unsuccessful. The patient underwent a third surgical revision, but complete fractures of the sternal bone prevented traditional sternal fixation techniques. To reconstruct the sternum, they decided to use a sternal allograft. Bone allograft is increasingly used for chest wall and sternum reconstruction with excellent outcomes (Figure 6). These allografts offer comparable benefits to bone autografts, including infection risk, compatibility, and host tissue integration, but without the need for extra incisions or tissue harvesting. Additionally, they are readily available from tissue banks (39-41).
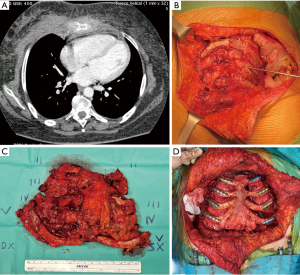
Finally, Limoges experience’s in treating refractory deep sternal wound infection after multiple debridement surgeries and VAC therapy by using an antibiotic loaded ceramic sternum deserves to be mentioned. They have reported a total of four infected patients treated by replacing destroyed sternum with gentamicin alone or in combination with vancomycin loaded in a porous ceramic sternum. Only one patient developed an infection of the device due to the presence of previously unknown gentamicin-resistant bacteria in the wound of patient at the time of implantation. The prosthesis was removed 19 months after its implantation without complications. Removal surgery was easy as the device was used as a dissection plan and the shape allowed to cut the bridges between it and the ribs. At the time of removal, underlying tissues were healed and strong enough to stabilize the chest wall and no complication occurred after this removal (42).
Sternotomy wounds are a major issue in heart surgery and, depending on the extension of the primary defect, its infections and the experience of the surgeon, adequate management includes removal of previous wires or prosthesis and the use of meshes or bone allografts to cover the defect, being them highly biocompatible, with low risk of infections and providing a good host tissue integration.
Role of soft tissues in complex chest wall reconstructions
Osteosynthesis systems are typically used together with myocutaneous flaps and/or meshes to cover complex chest wall defects and isolate the pleural space. Myocutaneous flaps can promote early incision healing and prevent infection, making it possible for patients to undergo adjuvant radiotherapy, chemotherapy, or other treatments more quickly. A variety of vascularized tissue flaps are used to aid in healing anastomoses or closures considered to be at risk for dehiscence and to help eradicate and sterilize infected spaces. The most commonly used flaps in these situations are intrathoracic transposition of intercostal, serratus anterior, LD, or pectoralis major muscle (43). The greater omentum is another option that can be transposed to any area on the anterior and lateral chest wall, providing a reliable blood supply and allowing for coverage of very large areas. It is particularly useful in the most complex cases involving high-risk closures and infected spaces, and it provides many advantages (44). In nearly all cases, an omental pedicle based on the right gastroepiploic artery can reach any location in the chest. The omentum’s bulk and pliability make it particularly well-suited to completely filling irregular spaces and to closely adhering to at-risk anastomoses that may be in relatively inaccessible locations. Additionally, the omentum delivers vascular endothelial growth factor, a potent angiogenic factor, which brings oxygenated blood to sites of ischemia (45). However, in cases of massive chest wall defects (defined as larger than two-thirds of the anterior chest wall), classic reconstructive options like the omentum or LD may not provide sufficient soft tissue coverage. In such cases, a large flap may be obtained from the abdomen to reconstruct the chest wall. In recent experiences, the anterolateral thigh (ALT) flap has been proven to be the workhorse flap to reconstruct large defects in the head and neck, lower limb, and thoracic wall. The anteromedial thigh (AMT) flap has also been used in conjunction with the ALT to reconstruct large chest wall defects (46) (Figure 1).
Future perspectives
The development of 3D custom-made prostheses has opened up a new realm of personalized approaches to chest wall reconstruction (47). However, any new technique must adhere to Okereke’s postulates (48) to ensure clear indications, contained costs, and demonstrable functional results. Currently, researchers are using mathematical models that consider the entire rib cage as a whole, along with new generation materials with improved mechanical behavior, to improve osseointegration and bone adaptation to the prosthesis (49-52). Future chest wall implants will not be just inert structures, but will have therapeutic capacity. Recent studies have shown the feasibility of developing stimuli-responsive nanosystems based on mesoporous silica nanoparticles or the adaptive-response bioceramics for antimicrobial purposes (53,54). Another approach would be the use of 3D-printed biological scaffolds that would be colonized by the patient’s cells once implanted. These scaffolds are capable of supporting the marrow structure and mimic the geometry of a vertebral body (55).
Conclusions
In conclusion, extensive surgery is often necessary for primary chest wall tumors, advanced breast cancers, congenital defects, and chest wall osteoradionecrosis. In the future, emerging intraoperative real-time imaging and 3D printing technology, as well as development in biomaterials, will allow chest wall reconstruction to become increasingly personalized. However, still today, these procedures have a high complication rate and may require reintervention due to recurrent tumor, prosthesis failure, defect extension, or local conditions. Each situation requires a personalized approach as no guidelines exist for their management. Collagen matrices and titanium plates are now used in clinical practice due to their biocompatibility and ability to be easily incorporated into compromised local areas, including infected or heavily irradiated ones, without needing removal in case of suppurative complications. Proper pre-surgical planning is crucial for complex cases such as infections and radiation-induced chest ulcers after previous surgical treatment. The surgical procedure should involve debridement of the thoracic wall, excision of tissue necrosis, pulse-jet lavage, and removal of the prosthesis. VAC-therapy could be used as a bridge for chest wall re-reconstruction. Sternotomy wounds are a major issue in heart surgery. Adequate management includes removal of previous wires or prosthesis and the use of meshes or bone allografts to cover the defect, being highly biocompatible with low risk of infections and providing good host tissue integration. These procedures should be carried out only in high-volume and selected centers due to the technical difficulty of these reinterventions.
Acknowledgments
Funding: None.
Footnote
Provenance and Peer Review: This article was commissioned by the Guest Editors (Erik de Loos, José Ribas de Campos, and Jean Daemen) for the series “Chest Wall Resections and Reconstructions” published in Journal of Thoracic Disease. The article has undergone external peer review.
Reporting Checklist: The authors have completed the Narrative Review reporting checklist. Available at https://jtd.amegroups.com/article/view/10.21037/jtd-23-1431/rc
Peer Review File: Available at https://jtd.amegroups.com/article/view/10.21037/jtd-23-1431/prf
Conflicts of Interest: All authors have completed the ICMJE uniform disclosure form (available at https://jtd.amegroups.com/article/view/10.21037/jtd-23-1431/coif). The series “Chest Wall Resections and Reconstructions” was commissioned by the editorial office without any funding or sponsorship. Giuseppe Marulli serves as an unpaid editorial board member of Journal of Thoracic Disease from February 2023 to January 2025. The authors have no other conflicts of interest to declare.
Ethical Statement: The authors are accountable for all aspects of the work, ensuring that questions related to the accuracy or integrity of any part of the work are appropriately investigated and resolved. All clinical procedures described in this study were performed in accordance with the ethical standards of the institutional and/or national research committee(s) and with the Helsinki Declaration (as revised in 2013). Written informed consent was obtained from the patients or patient’s guardians for the publication of this article and accompanying images.
Open Access Statement: This is an Open Access article distributed in accordance with the Creative Commons Attribution-NonCommercial-NoDerivs 4.0 International License (CC BY-NC-ND 4.0), which permits the non-commercial replication and distribution of the article with the strict proviso that no changes or edits are made and the original work is properly cited (including links to both the formal publication through the relevant DOI and the license). See: https://creativecommons.org/licenses/by-nc-nd/4.0/.
References
- Gonfiotti A, Salvicchi A, Voltolini L. Chest-Wall Tumors and Surgical Techniques: State-of-the-Art and Our Institutional Experience. J Clin Med 2022;11:5516. [Crossref] [PubMed]
- Arya R, Chow WT, Rozen WM, et al. Microsurgical Reconstruction of Large Oncologic Chest Wall Defects for Locally Advanced Breast Cancer or Osteoradionecrosis: A Retrospective Review of 26 Cases over a 5-Year Period. J Reconstr Microsurg 2016;32:121-7. [Crossref] [PubMed]
- Zhang X, Cai Z, Liu B, et al. Finite element analysis and clinical study of chest wall reconstruction using carbon fiber artificial rib. Sci Rep 2024;14:97. [Crossref] [PubMed]
- Berthet JP, Wihlm JM, Canaud L, et al. The combination of polytetrafluoroethylene mesh and titanium rib implants: an innovative process for reconstructing large full thickness chest wall defects. Eur J Cardiothorac Surg 2012;42:444-53. [Crossref] [PubMed]
- Deschamps C, Tirnaksiz BM, Darbandi R, et al. Early and long-term results of prosthetic chest wall reconstruction. J Thorac Cardiovasc Surg 1999;117:588-91; discussion 591-2. [Crossref] [PubMed]
- Weyant MJ, Bains MS, Venkatraman E, et al. Results of chest wall resection and reconstruction with and without rigid prosthesis. Ann Thorac Surg 2006;81:279-85. [Crossref] [PubMed]
- Wouters MW, van Geel AN, Nieuwenhuis L, et al. Outcome after surgical resections of recurrent chest wall sarcomas. J Clin Oncol 2008;26:5113-8. [Crossref] [PubMed]
- Abbas AE, Deschamps C, Cassivi SD, et al. Chest-wall desmoid tumors: results of surgical intervention. Ann Thorac Surg 2004;78:1219-23. [Crossref] [PubMed]
- King RM, Pairolero PC, Trastek VF, et al. Primary chest wall tumors: factors affecting survival. Ann Thorac Surg 1986;41:597-601. [Crossref] [PubMed]
- McMillan RR, Sima CS, Moraco NH, et al. Recurrence patterns after resection of soft tissue sarcomas of the chest wall. Ann Thorac Surg 2013;96:1223-8. [Crossref] [PubMed]
- Endara SA, De la Torre JS, Terán FJ, et al. Multidisciplinary management of recurrent synovial sarcoma of the chest wall. N Am Spine Soc J 2023;15:100243. [Crossref] [PubMed]
- Vanstraelen S, Ali B, Bains MS, et al. The contribution of microvascular free flaps and pedicled flaps to successful chest wall surgery. J Thorac Cardiovasc Surg 2023;166:1262-1272.e2. [Crossref] [PubMed]
- Pang K, Guo X, Liu T, et al. The Role of a Multidisciplinary Team in the Diagnosis and Treatment of Bone and Soft Tissue Sarcomas: A Single-Center Experience. J Pers Med 2022;12:2079. [Crossref] [PubMed]
- Young JS, McAllister M, Marshall MB. Three-dimensional technologies in chest wall resection and reconstruction. J Surg Oncol 2023;127:336-42. [Crossref] [PubMed]
- Berthet JP, Gomez Caro A, Solovei L, et al. Titanium Implant Failure After Chest Wall Osteosynthesis. Ann Thorac Surg 2015;99:1945-52. [Crossref] [PubMed]
- De Palma A, Sollitto F, Loizzi D, et al. Chest wall stabilization and reconstruction: short and long-term results 5 years after the introduction of a new titanium plates system. J Thorac Dis 2016;8:490-8. [Crossref] [PubMed]
- Clermidy H, Fadel G, De Lemos A, et al. Long-term outcomes after chest wall resection and repair with titanium bars and sternal plates. Front Surg 2022;9:950177. [Crossref] [PubMed]
- Wong THY, Siu ICH, Lo KKN, et al. Ten-Year Experience of Chest Wall Reconstruction: Retrospective Review of a Titanium Plate MatrixRIB™ System. Front Surg 2022;9:947193. [Crossref] [PubMed]
- Bergovec M, Smolle M, Lindenmann J, et al. High complication rate with titanium plates for chest wall reconstruction following tumour resection. Eur J Cardiothorac Surg 2022;62:ezac534. [Crossref] [PubMed]
- Petrella F, Casiraghi M, Mariolo AV, et al. Rigid prosthesis removal following chest wall resection and reconstruction for cancer. Shanghai Chest 2018;2:64. [Crossref]
- Rocco G. Chest wall resection and reconstruction according to the principles of biomimesis. Semin Thorac Cardiovasc Surg 2011;23:307-13. [Crossref] [PubMed]
- Ng CS. Recent and Future Developments in Chest Wall Reconstruction. Semin Thorac Cardiovasc Surg 2015;27:234-9. [Crossref] [PubMed]
- Shah NR, Ayyala HS, Tran BNN, et al. Outcomes in Chest Wall Reconstruction Using Methyl Methacrylate Prostheses: A Review of the Literature and Case Series Utilizing a Novel Approach with Biologic Mesh. J Reconstr Microsurg 2019;35:575-86. [Crossref] [PubMed]
- Shah VN, Orlov O, Hoffman R, et al. Reoperation 7 years after sternal reconstruction with a porcine acellular dermal matrix. Eur J Cardiothorac Surg 2018;54:601-3. [Crossref] [PubMed]
- Gonfiotti A, Santini PF, Campanacci D, et al. Malignant primary chest-wall tumours: techniques of reconstruction and survival. Eur J Cardiothorac Surg 2010;38:39-45. [Crossref] [PubMed]
- Koppert LB, van Geel AN, Lans TE, et al. Sternal resection for sarcoma, recurrent breast cancer, and radiation-induced necrosis. Ann Thorac Surg 2010;90:1102-1108.e2. [Crossref] [PubMed]
- Gaudreau G, Costache V, Houde C, et al. Recurrent sternal infection following treatment with negative pressure wound therapy and titanium transverse plate fixation. Eur J Cardiothorac Surg 2010;37:888-92. [Crossref] [PubMed]
- Berthet JP, Solovei L, Tiffet O, et al. Chest-wall reconstruction in case of infection of the operative site: is there any interest in titanium rib osteosynthesis? Eur J Cardiothorac Surg 2013;44:866-74. [Crossref] [PubMed]
- Voss B, Bauernschmitt R, Will A, et al. Sternal reconstruction with titanium plates in complicated sternal dehiscence. Eur J Cardiothorac Surg 2008;34:139-45. [Crossref] [PubMed]
- Haslam M, Dentcheva E, Shafqat MS, et al. Structural Failure of the BioBridge Rib Prosthesis After Chest Wall Reconstruction. Ann Thorac Surg 2022;114:e233-5. [Crossref] [PubMed]
- Rocco G, Martucci N, La Rocca A, et al. Postoperative local morbidity and the use of vacuum-assisted closure after complex chest wall reconstructions with new and conventional materials. Ann Thorac Surg 2014;98:291-6. [Crossref] [PubMed]
- Hoganson DM, O'Doherty EM, Owens GE, et al. The retention of extracellular matrix proteins and angiogenic and mitogenic cytokines in a decellularized porcine dermis. Biomaterials 2010;31:6730-7. [Crossref] [PubMed]
- DiCocco JM, Fabian TC, Emmett KP, et al. Components separation for abdominal wall reconstruction: the Memphis modification. Surgery 2012;151:118-25. [Crossref] [PubMed]
- Hosseinrezaei H, Rafiei H, Amiri M. Incidence and risk factors of sternal wound infection at site of incision after open-heart surgery. J Wound Care 2012;21:408-11. [Crossref] [PubMed]
- Listewnik MJ, Sielicki P, Mokrzycki K, et al. The Use of Vacuum-Assisted Closure in Purulent Complications and Difficult-To-Heal Wounds in Cardiac Surgery. Adv Clin Exp Med 2015;24:643-50. [Crossref] [PubMed]
- Dell'Amore A, Campisi A, Giunta D, et al. Surgical options to treat massive sternal defect after failed Robicsek procedure. J Thorac Dis 2018;10:E410-5. [Crossref] [PubMed]
- Robicsek F, Daugherty HK, Cook JW. The prevention and treatment of sternum separation following open heart surgery. Coll Works Cardiopulm Dis 1977;21:61-3. [Crossref] [PubMed]
- Dell'Amore A, Dolci G, Cassanelli N, et al. A massive post-sternotomy sternal defect treated by allograft sternal transplantation. J Card Surg 2012;27:557-9. [Crossref] [PubMed]
- Marulli G, Hamad AM, Cogliati E, et al. Allograft sternochondral replacement after resection of large sternal chondrosarcoma. J Thorac Cardiovasc Surg 2010;139:e69-70. [Crossref] [PubMed]
- Aranda JL, Varela G, Benito P, et al. Donor cryopreserved rib allografts for chest wall reconstruction. Interact Cardiovasc Thorac Surg 2008;7:858-60. [Crossref] [PubMed]
- Kalab M, Molitor M, Kubesova B, et al. Use of allogenous bone graft and osteosynthetic stabilization in treatment of massive post-sternotomy defects. Eur J Cardiothorac Surg 2012;41:e182-4. [Crossref] [PubMed]
- Tricard J, Chermat A, El Balkhi S, et al. An antibiotic loaded ceramic sternum to treat destroyed infected sternum: 4 cases. J Thorac Dis 2020;12:209-16. [Crossref] [PubMed]
- Novoa N, Benito P, Jiménez MF, et al. Reconstruction of chest wall defects after resection of large neoplasms: ten-year experience. Interact Cardiovasc Thorac Surg 2005;4:250-5. [Crossref] [PubMed]
- Shrager JB, Wain JC, Wright CD, et al. Omentum is highly effective in the management of complex cardiothoracic surgical problems. J Thorac Cardiovasc Surg 2003;125:526-32. [Crossref] [PubMed]
- Zhang QX, Magovern CJ, Mack CA, et al. Vascular endothelial growth factor is the major angiogenic factor in omentum: mechanism of the omentum-mediated angiogenesis. J Surg Res 1997;67:147-54. [Crossref] [PubMed]
- Sim NHS, Ngaserin S, Feng JJ, et al. Reconstruction of a massive chest wall defect using a free anterolateral-lower medial thigh flaps: a case report. J Surg Case Rep 2023;2023:rjad264. [Crossref] [PubMed]
- Novoa NM, Aranda Alcaide JL, Gomez Hernández MT, et al. Chest wall—reconstruction: yesterday, today and the future. Shanghai Chest 2019;3:15. [Crossref]
- Okereke IC. Is 3-dimensional printing the right fit for your reconstruction? J Thorac Cardiovasc Surg 2018;155:e61-2. [Crossref] [PubMed]
- Zhang G, Chen X, Ohgi J, et al. Biomechanical simulation of thorax deformation using finite element approach. Biomed Eng Online 2016;15:18. [Crossref] [PubMed]
- Dimas LS, Bratzel GH, Eylon I, et al. Tough Composites Inspired by Mineralized Natural Materials: Computation, 3D printing, and Testing. Adv Funct Mater 2013;23:4629-38. [Crossref]
- Albrektsson T, Johansson C. Osteoinduction, osteoconduction and osseointegration. Eur Spine J 2001;10:S96-101. [Crossref] [PubMed]
- Pontzer H, Lieberman DE, Momin E, et al. Trabecular bone in the bird knee responds with high sensitivity to changes in load orientation. J Exp Biol 2006;209:57-65. [Crossref] [PubMed]
- Paris JL, Cabañas MV, Manzano M, et al. Polymer-Grafted Mesoporous Silica Nanoparticles as Ultrasound-Responsive Drug Carriers. ACS Nano 2015;9:11023-33. [Crossref] [PubMed]
- Mirzaali MJ, van Dongen ICP, Tümer N, et al. In-silico quest for bactericidal but non-cytotoxic nanopatterns. Nanotechnology 2018;29:43LT02. [Crossref] [PubMed]
- Daly AC, Cunniffe GM, Sathy BN, et al. 3D Bioprinting of Developmentally Inspired Templates for Whole Bone Organ Engineering. Adv Healthc Mater 2016;5:2353-62. [Crossref] [PubMed]