Image quality and detection efficacy of zero echo time magnetic resonance imaging on Lung-RADS 2 pulmonary ground-glass nodules in comparison to thin-slice fat-saturated T2-weighted imaging
Highlight box
Key findings
• The detection rate for pure ground-glass nodules (pGGNs) by thin-slice fat-saturated T2-weighted imaging (80.0%) outperformed zero echo time (ZTE) (51.8%).
• ZTE visibility was associated with pathology from 0% for atypical adenomatous hyperplasia to 94.1% for invasive adenocarcinoma.
What is known and what is new?
• Magnetic resonance imaging (MRI) is a promising tool in lung nodule screening compared to computed tomography; however, the ability of MRI to detect the invasiveness of pGGN has seldom been investigated yet.
• Our study demonstrates the potential of MRI as an additional tool in assessing pGGN with a lower false positive rate.
What is the implication, and what should change now?
• Magnetic resonance could serve as a potential alternative for examinations in eligible pGGN patients who require long-term follow-up.
Introduction
With the prevalence of computed tomography (CT) screening, pulmonary pure ground-glass nodules (pGGNs) are increasingly being detected. The Lung Imaging Reporting and Data System (Lung-RADS) is a standardized classification system for lung cancer CT screening reports (1). pGGNs mostly belong to Lung-RADS 2 category. Despite its low malignancy risk, inaccurate classification may cause long-term follow-ups and anxiety for patients (1,2).
Local pGGNs encompass a range of benign and malignant entities, including focal inflammatory lesions (FILs), precursor glandular lesions [atypical adenomatous hyperplasia (AAH), adenocarcinoma in situ (AIS)], minimally invasive adenocarcinoma (MIA), and invasive adenocarcinoma (IAC) (3). Precursor glandular lesions are characterized by nearly 100% 5-year disease-free survival following surgery (4). However, MIA with poorly differentiated invasive patterns, such as micropapillary or solid predominant patterns, may recur or develop metachronous adenocarcinoma, requiring stricter monitoring and additional post-operation measures (5). IAC exhibits a relatively less favorable prognosis (6). Therefore, accurate prediction of pathological subtypes (e.g., invasive and non-invasive lesions) is essential for optimal patient management.
Size and CT values have been reported as predictive factors for the invasiveness of pure ground-glass opacity (pGGO), while some studies have indicated that CT density cannot effectively differentiate between invasive and preinvasive histology (7,8). Furthermore, dual-layer spectral detector CT (9) and CT-based radiomics (10,11) have been utilized to predict the invasiveness of pGGO. However, most previous studies have only included precursor lesions and invasive lesions, while FILs that are often clinically confusing have rarely been incorporated. In contrast, pulmonary magnetic resonance imaging (MRI) has emerged as a promising alternative to CT in the assessment of lung diseases, particularly for patients with lung nodules (12). Ultrashort echo time (UTE) MRI has shown promising results in lung nodule screening compared to low-dose and standard CT imaging (13,14); it has been a favorable and effective option for lung imaging, especially for those who are sensitive to radiation exposure (15). Zero echo time (ZTE) is another high-resolution pulmonary structural imaging with better image quality and shorter echo time than UTE (16). Additionally, T2-weighted imaging shows good performance on lung nodule screening (17).
To the best of our knowledge, there are few studies on the capability of ZTE for pGGN detection in comparison with thin-slice T2WI, not to mention research on the invasiveness of pGGN. Therefore, this study aimed to address this gap in knowledge of pulmonary MRI by comparing the detection rates of ZTE and thin-slice T2WI-FS for Lung-RADS 2 pGGNs and evaluating their ability to predict invasiveness on 3.0 T MRI. We present this article in accordance with the STARD reporting checklist (available at https://jtd.amegroups.com/article/view/10.21037/jtd-24-414/rc).
Methods
Patient population
This prospective study was conducted in accordance with the Declaration of Helsinki (as revised in 2013). The study was approved by the institutional review board of The First Affiliated Hospital of Guangzhou Medical University (No. 2022-63), and informed consent was taken from all the patients. The participant enrollment process is depicted in Figure 1. Between January and October 2022, 3,845 adult patients (aged over 18 years) visited the pulmonary outpatient clinic of The First Affiliated Hospital of Guangzhou Medical University due to the detection of pGGNs during routine health checkups. A total of 254 consecutive patients consented to participate in this study and had preoperative lung MRI after being thoroughly informed about the purpose and procedures of the study. Exclusion criteria were: (I) participants whose most recent CT scan was performed more than 1 week prior to their scheduled video-assisted thoracoscopic surgery (VATS). This ensured that the CT data were up-to-date and accurately reflected the state of the pGGNs; (II) contraindications to magnetic resonance (MR) imaging; (III) poor quality MR or CT images that hampered observation. Finally, 83 patients were included in the study, and none had participated in any previously published research.
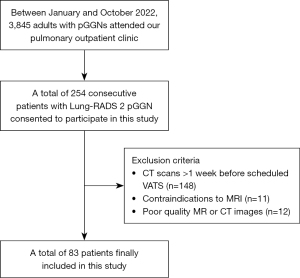
CT examinations
Inspiratory and breath-hold CT examinations were conducted using either a 64-detector row or a 128-detector row CT scanner (Brilliance, Philips Healthcare, Amsterdam, The Netherlands; SOMATOM Definition AS, Siemens Healthcare, Erlangen, Germany) with 120 kVp and 110–270 mAs. Images were reconstructed at 1 mm thickness covering the whole lungs. These thin-slice lung images were used to identify and measure the reference nodules.
MRI examinations
MRI scans were obtained using a 3.0 T scanner (750W, GE Healthcare, Milwaukee, WI, USA). Patients were wrapped a respiratory bellows at the upper abdomen and positioned foot-first supine at the magnetic field centered along the line connecting the sternal angle and the xiphoid process. 3D respiratory-triggered ZTE was performed with a non-selective hard pulse excitation followed by 3D center-out radial sampling at a silent scanning mode, using a 32-channel body coil without contrast enhancement. we performed a shimming procedure before each sequence acquisition to ensure optimal image quality and minimize artifacts related to field inhomogeneity. Respiratory-triggering coronal and transverse T2WI-FS and transverse ZTE lung scans were carried out sequentially. Image data were prospectively collected only when the diaphragm position was within an acceptable range for about one-third of the end-expiration phase for ZTE and T2WI-FS. The parameters of ZTE and T2WI-FS are presented in Table 1.
Table 1
Parameters | ZTE | T2WI-FS |
---|---|---|
FOV | 420 mm × 420 mm | 360 mm × 360 mm |
Matrix | 240 × 256 | 320 × 256 |
Slice thickness | 1.5 mm | 2.0 mm |
Bandwidth | 31.25 kHz | 83.33 kHz |
Flip angle | 2° | Auto refocus |
Image acquisition | Respiratory gating | Respiratory gating |
TR | 300–600 ms | >3,000 ms |
TE | 0 | 30–50 ms |
Acquired orientation | Transverse | Transverse |
Scan time, mean (range) | 281 s (210–305 s) | 227 s (201–246 s) |
MR, magnetic resonance; ZTE, zero echo time; T2WI-FS, thin-slice fat-saturated T2-weighted imaging; FOV, field of view; TR, repetition time; TE, echo time.
Quantitative and qualitative assessment
All images were reviewed and evaluated using the Picture Archiving and Communication System (PACS, Version 5.5; Neusoft Medical, Shenyang, China) at our institution. To objectively assess the image quality of ZTE and T2WI-FS sequences, signal intensities (SIs) of both normal structures and lung lesions were measured. Following the methodology outlined by Bae et al. (18), one radiologist (with 4 years of experience) placed circular regions of interest (ROIs) in specific areas including lung parenchyma, tracheal lumen and wall, aorta, subscapularis muscle, peripheral pulmonary vessel, peripheral bronchus, and lung nodules. Measurements were conducted three times for each ROI and averaged. The signal-to-noise ratios (SNRs) and contrast-to-noise ratios (CNRs) were then calculated for these structures. SNR was defined as the mean SI of the structure divided by noise, while CNR was calculated as the difference in mean SIs between the structure and lung parenchyma, divided by noise. Noise was characterized using the standard deviation (SD) of SI in the tracheal lumen.
For the qualitative assessment, separate evaluations of ZTE and T2WI-FS images were performed by two radiologists (with 10 and 8 years of experience, respectively) in a randomized order. A five-point scale was utilized to assess various parameters (18). These included visualization of pulmonary vessels and bronchi, sharpness of the diaphragmatic contour, and the presence of noise and artifacts. Overall diagnostic acceptability was also rated based on the same methodology.
Pathological analysis
The interval between pathological examination and CT/MRI scans did not exceed 1 week. Specimens were fixed in 10% neutral-buffered formalin, paraffin-embedded, and sectioned from lesion centers for hematoxylin and eosin staining. Two independent, blinded pathologists with expertise in pulmonary pathology evaluated the sections. Discrepancies were resolved through consensus to ensure accurate pathological diagnosis.
Data collection and analysis
CT images were transferred to commercial artificial intelligence (AI) software (Dr. Wise system, 1.22.07.31, Deep-wise Corporation, Hangzhou, China) for automatic detection and segmentation. The location, number, longest diameter, CT values (whole tumor measurement) and Lung-RADS scores of pGGNs were automatically generated by the AI software (19) and then assessed and confirmed on PACS with lung window settings [level, −600 Hounsfield unit (HU); width, 1,600 HU] by the fourth radiologist with 12 years of thoracic imaging experience as a reference standard for nodule detection.
The MRI images were independently reviewed on the same PACS by the same two radiologists who were blinded to the pathology results (with 10 years and 8 years of thoracic imaging experience, respectively) in a random order. Side-by-side comparison of MRI with CT scans to determine detectability of pulmonary pathologies. In cases of disagreement, a senior radiologist (with over 30 years of experience) served as an adjudicator.
Statistical analysis
The Wilcoxon signed-rank test was used to compare the differences in the SNR and CNR between ZTE and T2WI-FS for normal structures and lung nodules as well as subjective image quality scores. The weighted kappa coefficient was used to determine the inter-rater agreement for the qualitative assessment with the following established thresholds: slight (≤0.20); fair (0.21–0.40); moderate (0.41–0.60); substantial (0.61–0.80); almost perfect (0.81–1.00). The nodule diameters and CT values among different groups were compared using the Kruskal-Wallis test with a post-hoc test. The visibility of pGGNs among various pathological subgroups across different MRI sequences was examined for between-group differences using McNemar’s test. We performed hierarchical regression analysis to evaluate the moderating effects of various imaging features on the invasiveness of pGGNs. In Model 1, we entered variable CT values into the base model. In Model 2, we added diameter. In Model 3, ZTE visibility was added. A receiver operating characteristic (ROC) curve analysis and area under the curve (AUC) comparison were carried out to assess the diagnostic accuracy of pGGN. Statistical analyses were conducted using the Statistical Package for the Social Sciences (SPSS) version 25.0 (Chicago, IL, USA) and MedCalc version 18.2.1 (Ostend, Belgium). A P value less than 0.05 indicated statistical significance.
Results
Participants
A total of 83 subjects (62 women and 21 men) aged 49.8±11.8 years (range, 22–73 years) were included in this study. All pGGNs were surgically removed via thoracoscopic resection after CT and MRI scans; subsequently, they were pathologically identified and analyzed by two thoracic pathologists with results shown in Table 2.
Table 2
Characteristics | Values |
---|---|
Patient (n=83) | |
Age (years) | 49.8±11.8 [22–73] |
Gender (female/male) | 62/21 |
Number of nodules per patient | |
1 | 63 (75.9) |
2 | 14 (16.9) |
3 | 4 (4.8) |
4 | 2 (2.4) |
Nodule (n=110) | |
Diameter (mm) | 9.7±4.8 [4–26] |
Number of nodules per size category | |
<6 mm | 16 (14.6) |
6–10 mm | 58 (52.7) |
>10 mm | 36 (32.7) |
Number of nodules per location | |
RUL | 38 (34.5) |
RML | 14 (12.8) |
RLL | 20 (18.2) |
LUL | 23 (20.9) |
LLL | 15 (13.6) |
Number of nodules per surgical pathology | |
FIL | 10 (9.1) |
AAH | 4 (3.6) |
AIS | 19 (17.3) |
MIA | 60 (54.5) |
IAC | 17 (15.5) |
Values are presented as mean ± SD [range], number, or n (%). RUL, right upper lung; RML, right middle lung; RLL, right lower lung; LUL, left upper lung; LLL, left lower lung; FIL, focal inflammatory lesion; AAH, atypical adenomatous hyperplasia; AIS, adenocarcinoma in situ; MIA, minimally invasive adenocarcinoma; IAC, invasive adenocarcinoma; SD, standard deviation.
Quantitative and qualitative assessment
All SNRs and CNRs are shown in Figure 2. SNRs of the lung parenchyma, aorta, peripheral pulmonary vessel and peripheral bronchus (P<0.001) and CNRs of the peripheral pulmonary vessel (P<0.001) were higher on ZTE than on T2WI-FS, while SNRs of the tracheal wall and lung nodule and CNRs of peripheral bronchus and lung nodule (P<0.001) were significantly higher on T2WI-FS than on ZTE. There was no inter-modality difference for SNRs of subscapularis muscle (P=0.60).
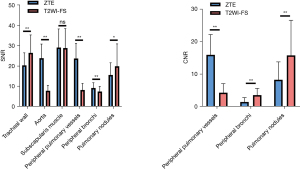
The subjective image quality of the pulmonary vessels and bronchus was considerably higher (P<0.001) on ZTE by both independent radiologists (Figure 3). However, the scores of noise, artifacts, and overall acceptability were higher on T2WI-FS than on ZTE (all P<0.001). There were no significantly different scores for diaphragmatic sharpness (P=0.31).
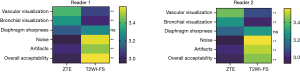
Inter-reader agreements on peripheral vessels (K=0.67 in ZTE, K=0.72 in T2WI-FS), bronchus (K=0.63 in ZTE, K=0.60 in T2WI-FS), diaphragmatic sharpness (K=0.72 in ZTE, K=0.75 in T2WI-FS), image noise (K=0.62 in ZTE, K=0.76 in T2WI-FS), artifacts (K=0.65 in ZTE.K=0.70 in T2WI-FS), and overall acceptability (K=0.62 in ZTE, K=0.64 in T2WI-FS) were substantial in both sequences.
Nodule characteristics
The characteristics of nodules in the five groups are shown in Table 3 and Figures 4-7. The diameters of FIL, AAH, AIS, MIA, and IAC were 5.55±2.51, 4.50±1.08, 5.92±1.57, 8.70±2.91 and 14.82±4.53 mm, respectively. IAC showed a significantly larger diameter than MIA, AIS, AAH, and FIL (all P<0.001), and MIA had a significantly larger diameter than AIS, AAH, and FIL (P=0.001, 0.007, 0.003). No statistical differences were observed in comparisons between the other groups.
Table 3
Variables | Pathological entities | P value | ||||
---|---|---|---|---|---|---|
FIL (n=10) | AAH (n=4) | AIS (n=19) | MIA (n=60) | IAC (n=17) | ||
CT value (HU) | −712.55±45.59 | −615.60±146.77 | −642.35±90.65 | −615.27±82.42 | −581.60±96.90 | 0.004 |
Diameter (mm) | 5.55±2.51 | 4.50±1.08 | 5.92±1.57 | 8.70±2.91 | 14.82±4.53 | <0.001 |
Data are presented as mean ± SD. FIL, focal inflammatory lesion; AAH, atypical adenomatous hyperplasia; AIS, adenocarcinoma in situ; MIA, minimally invasive adenocarcinoma; IAC, invasive adenocarcinoma; CT, computed tomography; HU, Hounsfield unit; SD, standard deviation.
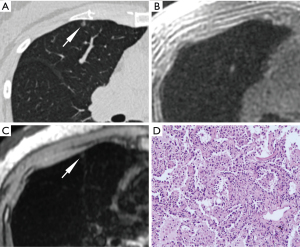
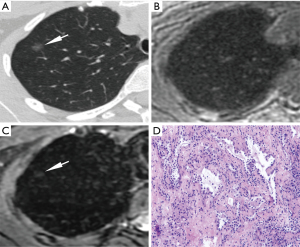
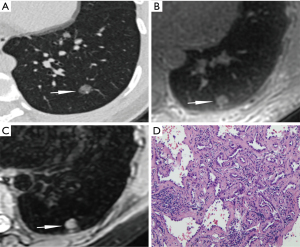
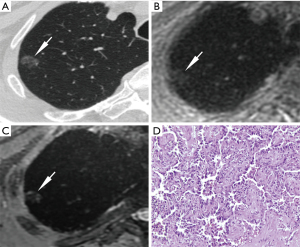
The CT values of FIL, AAH, AIS, MIA, and IAC were respectively −712.55±45.59, −615.60±146.77, −642.35±90.65, −615.27±82.42 and −581.60±96.90 in the unit of HU. AAH, MIA, and IAC showed significantly higher CT values than FIL (P=0.04, P=0.001, P<0.001, respectively), and IAC had significantly higher CT values than AIS (P=0.03). No statistical differences were observed in comparisons between the other groups.
MRI detection rate for pGGN
Among 110 pGGNs, the detection rates of ZTE and thin-sliced T2WI-FS were respectively 51.8% (57/110) and 80.0% (88/110). A significant difference in visibility was observed across the pathological entities on both ZTE MRI (P<0.001 for the overall comparison) and T2WI-FS (P=0.001 for the overall comparison). The visibility for AAH, AIS, MIA and IAC showed an increasing detectability ranging from low to high in alignment with the invasiveness. T2WI-FS demonstrated a universally higher detection rate across all pathologies compared to ZTE, with notable effectiveness in identifying MIA (90.0%) and IAC (94.1%). Even in cases of AAH, where ZTE showed no visibility, T2WI-FS was able to detect 50.0% of these nodules (Table 4).
Table 4
Variables | ZTE, n (%) | T2WI-FS, n (%) | |||||||
---|---|---|---|---|---|---|---|---|---|
Invisible | Visible | Total | P value | Invisible | Visible | Total | P value | ||
FIL | 8 (80.0) | 2 (20.0) | 10 | <0.001 | 5 (50.0) | 5 (50.0) | 10 | 0.001 | |
AAH | 4 (100.0) | 0 (0.0) | 4 | 2 (50.0) | 2 (50.0) | 4 | |||
AIS | 15 (78.9) | 4 (21.1) | 19 | 8 (42.1) | 11 (57.9) | 19 | |||
MIA | 25 (41.7) | 35 (58.3) | 60 | 6 (10.0) | 54 (90.0) | 60 | |||
IAC | 1 (5.9) | 16 (94.1) | 17 | 1 (5.9) | 16 (94.1) | 17 | |||
Total | 53 (48.2) | 57 (51.8) | 110 | 22 (20.0) | 88 (80.0) | 110 |
MRI, magnetic resonance imaging; ZTE, zero echo time; T2WI-FS, thin-slice fat-saturated T2-weighted imaging; FIL, focal inflammatory lesion; AAH, atypical adenomatous hyperplasia; AIS, adenocarcinoma in situ; MIA, minimally invasive adenocarcinoma; IAC, invasive adenocarcinoma.
According to nodule size, the detection rates of ZTE and thin-sliced T2WI-FS were respectively 12.5% and 37.5% for pGGNs <6 mm, 44.8% and 81.0% for 6–10 mm, and 80.6% and 97.2% for >10 mm (Figure 8). Inter-reader agreement on the detection rate for pGGNs was substantial for both ZTE (K=0.71, P<0.001) and T2WI-FS (K=0.69, P<0.001).
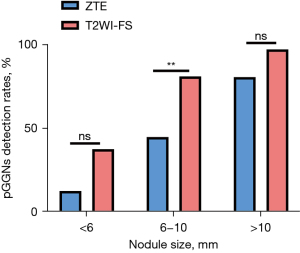
Hierarchical regression analysis
The result of the hierarchical regression analysis of the imaging features predicting invasiveness of pGGNs is shown in Table 5. In the first model, the CT value significantly explained 7.1% of the variance in ICA + MIA pathology (R2=0.071, F=8.212, P=0.005). In the second model, the inclusion of nodule diameter significantly increased the explanatory power to 26.4% (R2=0.264, F=19.221, P<0.001). In the third model, the addition of ZTE visibility further improved the model, explaining 29.7% of the variance with significant changes in R2 of 3.3% (F=14.925, P=0.02).
Table 5
Variables | Model 1 | Model 2 | Model 3 | ||||||||
---|---|---|---|---|---|---|---|---|---|---|---|
B | P | β | B | P | β | B | P | β | |||
Constant | 1.539 | <0.001** | – | 0.881 | 0.003** | – | 0.682 | 0.02* | – | ||
CT value | 0.001 | 0.005** | 0.266 | 0.001 | 0.02* | 0.19 | 0.001 | 0.13 | 0.131 | ||
Diameter | 0.043 | <0.001** | 0.447 | 0.034 | <0.001** | 0.354 | |||||
ZTE visibility | 0.197 | 0.02* | 0.215 | ||||||||
R2 | 0.071 | 0.264 | 0.297 | ||||||||
F value | 8.212, P=0.005 | 19.221, P<0.001 | 14.925, P<0.001 | ||||||||
ΔR2 | 0.071 | 0.194 | 0.033 | ||||||||
ΔF value | 8.212, P=0.005 | 28.164, P<0.001 | 4.924, P=0.02 |
*, P<0.05; **, P<0.01. pGGNs, pulmonary pure ground-glass nodules; B, unstandardized coefficients; β, standardized coefficients; CT, computed tomography; ZTE, zero echo time.
Diagnostic performance of image parameters
The diameter had the highest diagnostic performance on differentiating invasive pGGNs from non-invasive ones [AUC =0.874, 95% confidence interval (CI): 0.800–0.947], followed by ZTE-MRI (AUC =0.740, 95% CI: 0.641–0.840), while the CT values (AUC =0.682, 95% CI: 0.564–0.792) and T2WI-FS (AUC =0.678, 95% CI: 0.564–0.800) exhibited relatively lower AUCs. The diagnostic performance of diameter was significantly better than the other three indicators (P=0.005, 0.006, <0.001), while the differences among the other indicators were not statistically significant (Figure 9).
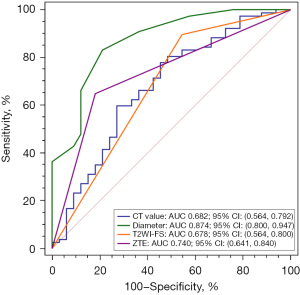
Discussion
The inherent properties of lung tissue, such as low proton density and short T2 relaxation time, have posed significant challenges for effective MR imaging. Advancements in MRI technology have led to the development of short T2-sensitive techniques like ZTE, which captures signals from rapidly decaying tissues by employing extremely short echo times, minimizing signal loss and providing greater detail and contrast in lung tissue imaging. While ZTE and traditional T2WI have shown promise in lung nodule detection (13,14,17), comparative analyses of these sequences and their efficacy in assessing the invasiveness of pGGNs remain underexplored. The present study first compared the values of ZTE and thin-slice T2WI-FS in detecting and predicting the invasiveness of Lung-RADS 2 pGGNs on 3.0 T MRI. Our findings revealed that ZTE exhibited a greater potential in predicting the histological invasiveness of pGGNs, while T2WI-FS demonstrated a higher detection rate for pGGNs.
Our analysis encompassed both subjective and objective assessments of image quality across the two sequences. The study demonstrated substantial inter-rater agreement in the subjective evaluation of pulmonary tissues using either ZTE-MRI or T2WI-FS, indicating both sequences exhibit good reproducibility and reliability for lung MR imaging. Objectively, ZTE displayed significantly higher SNR and CNR in lung parenchyma and pulmonary vessels, underscoring its enhanced capability in delineating these structures. Conversely, T2WI-FS revealed superior SNR and CNR for lung nodules, highlighting its effectiveness in nodule characterization. This indicates that different sequences possess unique advantages, necessitating their integrated use in imaging protocols. Although UTE is recommended for lung MRI applications due to its excellent assessment of lung parenchyma (12,20), T2WI-FS is indispensable for detecting and monitoring lung nodules in clinical practice.
The current study delineated invasive from preinvasive pGGNs using nodule size and CT density, achieving AUC of 0.874 and 0.682, respectively, underscoring the diagnostic utility of these parameters. An increase in the size of pGGNs correlates with a heightened risk of malignancy, a finding consistent with prior studies (21,22). While our results regarding CT values in differentiating pGGNs’ pathological entities resonate with several studies (21,23), they diverge from others (8), suggesting a complex landscape of diagnostic markers. Of note, most prior CT studies relied on manual measurements, whereas automatic measure was carried out in our study. This methodological refinement aimed to minimize subjective variability, enhancing the objectivity and accuracy of our results.
A novel aspect of our study was the notable association of ZTE visibility with the histological invasiveness of pGGNs. ZTE visibility offered slightly better diagnostic performance in distinguishing between non-invasive and invasive pGGNs (AUC =0.740) compared to CT density (AUC =0.682) and T2WI-FS (AUC =0.678). However, its diagnostic performance did not exceed the predictive power of nodule diameter, which achieved the highest AUC value of 0.874. This reaffirms the diameter of pGGNs as a critical determinant in evaluating their potential invasiveness. The association of ZTE visibility with histological invasiveness highlights its potential utility as a complementary imaging technique. Larger and denser nodules are more likely to be detected on ZTE, which suggests a higher probability of malignancy. Nevertheless, the absence of a lesion on ZTE might not completely exclude the possibility of malignancy, as smaller or less dense nodules may not be visible despite their invasive nature.
We conducted a hierarchical regression analysis to further clarify the relationship between nodule size, density, and ZTE visibility. Our findings suggest that ZTE-MRI provides additional insights into nodule invasiveness, even when nodule diameter and CT values are considered. This layered approach to regression analysis reinforces the complementary strengths of CT and MRI in the comprehensive evaluation of pGGNs.
Several limitations of our study still need to be considered. Firstly, a relatively small sample size at a single hospital may limit the generalization of our findings. Secondly, we did not compare with conventional T1-weighted images (T1WI), for UTE with superior performance on lung imaging to standard post-contrast T1WI (15). Thirdly, an inherent and unavoidable limitation presented in our study population was exclusively including patients undergoing surgery to have histological results as reference. This selection criterion may introduce bias and cannot represent the broader spectrum of patients with pGGNs, potentially affecting the applicability of our results to non-surgical cases. Last but not least, although radiologists were blinded to pathology results, MR images were directly compared with CT images, which was necessary as noise on ZTE could sometimes be confused with ground-glass opacities. Future research should focus on improving the SNR of ZTE to reduce such confusion and evaluate MRI’s ability to detect and assess pGGN when radiologists are blinded to CT images. However, this study is significant in demonstrating that MRI can serve as a viable follow-up tool for pGGN confirmed by CT, providing a non-ionizing alternative for long-term monitoring and potentially reducing radiation exposure from repeated CT scans.
Conclusions
Our study highlights the complementary strengths of thin-slice T2WI-FS and ZTE sequences in the imaging of pulmonary ground glass nodules. T2WI-FS excels in detection rate, making it a valuable tool for identifying pGGNs, while ZTE provides superior insight into the histological invasiveness of these nodules. In clinical practice, the combined use of T2WI and ZTE is recommended to leverage their respective advantages, offering a comprehensive, non-radiative approach for evaluating pGGNs.
Acknowledgments
Funding: This work was supported by
Footnote
Reporting Checklist: The authors have completed the STARD reporting checklist. Available at https://jtd.amegroups.com/article/view/10.21037/jtd-24-414/rc
Data Sharing Statement: Available at https://jtd.amegroups.com/article/view/10.21037/jtd-24-414/dss
Peer Review File: Available at https://jtd.amegroups.com/article/view/10.21037/jtd-24-414/prf
Conflicts of Interest: All authors have completed the ICMJE uniform disclosure form (available at https://jtd.amegroups.com/article/view/10.21037/jtd-24-414/coif). W.V.L. is an employee of GE Healthcare, Beijing, China. The other authors have no conflicts of interest to declare.
Ethical Statement: The authors are accountable for all aspects of the work in ensuring that questions related to the accuracy or integrity of any part of the work are appropriately investigated and resolved. This study was conducted in accordance with the Declaration of Helsinki (as revised in 2013). The study was approved by the institutional ethics board of The First Affiliated Hospital of Guangzhou Medical University (No. 2022-63), and informed consent was taken from all the patients.
Open Access Statement: This is an Open Access article distributed in accordance with the Creative Commons Attribution-NonCommercial-NoDerivs 4.0 International License (CC BY-NC-ND 4.0), which permits the non-commercial replication and distribution of the article with the strict proviso that no changes or edits are made and the original work is properly cited (including links to both the formal publication through the relevant DOI and the license). See: https://creativecommons.org/licenses/by-nc-nd/4.0/.
References
- Chelala L, Hossain R, Kazerooni EA, et al. Lung-RADS Version 1.1: Challenges and a Look Ahead, From the AJR Special Series on Radiology Reporting and Data Systems. AJR Am J Roentgenol 2021;216:1411-22. [Crossref] [PubMed]
- ACoR. Lung-Screening Reporting and Data System (LungRADS) Version 1.1. 2019. Available online: https://www.acr.org/-/media/ACR/Files/RADS/Lung-RADS/LungRADSAssessmentCategoriesv1-1.pdf?la=en
- Chang YC, Chen PT, Hsieh MS, et al. Discrimination of invasive lung adenocarcinoma from Lung-RADS category 2 nonsolid nodules through visual assessment: a retrospective study. Eur Radiol 2024;34:3453-61. [Crossref] [PubMed]
- Yotsukura M, Asamura H, Motoi N, et al. Long-Term Prognosis of Patients With Resected Adenocarcinoma In Situ and Minimally Invasive Adenocarcinoma of the Lung. J Thorac Oncol 2021;16:1312-20. [Crossref] [PubMed]
- Jia M, Yu S, Cao L, et al. Clinicopathologic Features and Genetic Alterations in Adenocarcinoma In Situ and Minimally Invasive Adenocarcinoma of the Lung: Long-Term Follow-Up Study of 121 Asian Patients. Ann Surg Oncol 2020;27:3052-63. [Crossref] [PubMed]
- Van Schil PE, Asamura H, Rusch VW, et al. Surgical implications of the new IASLC/ATS/ERS adenocarcinoma classification. Eur Respir J 2012;39:478-86. [Crossref] [PubMed]
- Lee HY, Choi YL, Lee KS, et al. Pure ground-glass opacity neoplastic lung nodules: histopathology, imaging, and management. AJR Am J Roentgenol 2014;202:W224-33. [Crossref] [PubMed]
- Fu F, Zhang Y, Wang S, et al. Computed tomography density is not associated with pathological tumor invasion for pure ground-glass nodules. J Thorac Cardiovasc Surg 2021;162:451-459.e3. [Crossref] [PubMed]
- Yu Y, Fu Y, Chen X, et al. Dual-layer spectral detector CT: predicting the invasiveness of pure ground-glass adenocarcinoma. Clin Radiol 2022;77:e458-65. [Crossref] [PubMed]
- Zhu YQ, Liu C, Mo Y, et al. Radiomics for differentiating minimally invasive adenocarcinoma from precursor lesions in pure ground-glass opacities on chest computed tomography. Br J Radiol 2022;95:20210768. [Crossref] [PubMed]
- Zhu M, Yang Z, Wang M, et al. A computerized tomography-based radiomic model for assessing the invasiveness of lung adenocarcinoma manifesting as ground-glass opacity nodules. Respir Res 2022;23:96. [Crossref] [PubMed]
- Hatabu H, Ohno Y, Gefter WB, et al. Expanding Applications of Pulmonary MRI in the Clinical Evaluation of Lung Disorders: Fleischner Society Position Paper. Radiology 2020;297:286-301. [Crossref] [PubMed]
- Ohno Y, Takenaka D, Yoshikawa T, et al. Efficacy of Ultrashort Echo Time Pulmonary MRI for Lung Nodule Detection and Lung-RADS Classification. Radiology 2022;302:697-706. [Crossref] [PubMed]
- Ohno Y, Koyama H, Yoshikawa T, et al. Standard-, Reduced-, and No-Dose Thin-Section Radiologic Examinations: Comparison of Capability for Nodule Detection and Nodule Type Assessment in Patients Suspected of Having Pulmonary Nodules. Radiology 2017;284:562-73. [Crossref] [PubMed]
- Renz DM, Herrmann KH, Kraemer M, et al. Ultrashort echo time MRI of the lung in children and adolescents: comparison with non-enhanced computed tomography and standard post-contrast T1w MRI sequences. Eur Radiol 2022;32:1833-42. [Crossref] [PubMed]
- Bae K, Jeon KN, Hwang MJ, et al. Comparison of lung imaging using three-dimensional ultrashort echo time and zero echo time sequences: preliminary study. Eur Radiol 2019;29:2253-62. [Crossref] [PubMed]
- Meier-Schroers M, Homsi R, Gieseke J, et al. Lung cancer screening with MRI: Evaluation of MRI for lung cancer screening by comparison of LDCT- and MRI-derived Lung-RADS categories in the first two screening rounds. Eur Radiol 2019;29:898-905. [Crossref] [PubMed]
- Bae K, Jeon KN, Hwang MJ, et al. Respiratory motion-resolved four-dimensional zero echo time (4D ZTE) lung MRI using retrospective soft gating: feasibility and image quality compared with 3D ZTE. Eur Radiol 2020;30:5130-8. [Crossref] [PubMed]
- Qi LL, Wu BT, Tang W, et al. Long-term follow-up of persistent pulmonary pure ground-glass nodules with deep learning-assisted nodule segmentation. Eur Radiol 2020;30:744-55. [Crossref] [PubMed]
- Ohno Y, Koyama H, Yoshikawa T, et al. Pulmonary high-resolution ultrashort TE MR imaging: Comparison with thin-section standard- and low-dose computed tomography for the assessment of pulmonary parenchyma diseases. J Magn Reson Imaging 2016;43:512-32. [Crossref] [PubMed]
- Chu ZG, Li WJ, Fu BJ, et al. CT Characteristics for Predicting Invasiveness in Pulmonary Pure Ground-Glass Nodules. AJR Am J Roentgenol 2020;215:351-8. [Crossref] [PubMed]
- Hsu WC, Huang PC, Pan KT, et al. Predictors of Invasive Adenocarcinomas among Pure Ground-Glass Nodules Less Than 2 cm in Diameter. Cancers (Basel) 2021;13:3945. [Crossref] [PubMed]
- Qi L, Xue K, Li C, et al. Analysis of CT morphologic features and attenuation for differentiating among transient lesions, atypical adenomatous hyperplasia, adenocarcinoma in situ, minimally invasive and invasive adenocarcinoma presenting as pure ground-glass nodules. Sci Rep 2019;9:14586. [Crossref] [PubMed]