Blood urea nitrogen-to-albumin ratio independently predicts 30-day mortality in acute respiratory failure patients: a retrospective cohort study
Highlight box
Key findings
• The study finds out that the correlation between baseline blood urea nitrogen-to-albumin ratio (BAR) and 30-day all-cause mortality in patients with acute respiratory failure (ARF) was non-linear, with a significant inflection point (11.76 mg/g). The Kaplan-Meier curve demonstrated that ARF patients had higher 30-day all-cause mortality rates when they had higher BAR levels (>11.76 mg/g).
What is known and what is new?
• Previous studies have explored the use of prognostic biomarkers based on a combination of blood urea nitrogen (BUN) and albumin levels in patient with respiratory disease.
• We are the first to investigate the combined use of BUN and albumin as a prognostic indicator for ARF patients across a diverse range of etiologies within a large-scale dataset.
What is the implication, and what should change now?
• A high BAR was linked to a higher risk of mortality in ARF patients, indicating that BAR could serve as a straightforward and possibly useful prognostic biomarker for ARF. Additional studies are warranted to evaluate this biomarker panel and to further elucidate the underlying biological mechanisms involved.
Introduction
Acute respiratory failure (ARF) is a common and prevalent life-threatening medical condition (1). More than 50% of severely ill patients admitted to the intensive care unit (ICU) suffer from ARF due to respiratory disease or pulmonary vascular disease (2). There are several other etiologies of ARF, including sepsis, congestive heart failure, and central nervous system depression (3). The in-hospital mortality rate of ARF patients who require invasive mechanical ventilation is 33–37% (4). The precise prediction of ARF severity may facilitate the early identification of patients approaching critical condition, enabling timely interventions that could potentially reduce the duration of their ICU stay and enhance their probability of survival with reduced morbidity (5). Therefore, biomarkers that can accurately and rapidly predict ARF mortality are needed.
However, predictive biomarkers for therapeutic decisions are critically deficient. Interleukin-1 (IL-1), IL-6, IL-8, tumor necrosis factor alpha (TNF-α), and Galectin-3 have all been linked to the prognosis of ARF patients (6,7); however, these biomarkers are not included in routine laboratory tests and results cannot be obtained immediately. Previous studies have shown that blood urea nitrogen (BUN) and albumin, two routinely used laboratory indicators, can predict the severity of disease-related mortality (8,9). BUN is a significant indicator of dehydration status and is typically used to evaluate renal function and hypovolemia. It is an important parameter for several types of severity assessment scores in community acquired pneumonia, such as SMARTCOP (10), REA-ICU (11), and CURB-65 (12). Elevated BUN levels are associated with poor clinical results in patients with community-acquired pneumonia (CAP) and heart failure (13,14). Albumin is an acute-phase reactant that is usually used to assess malnutrition (15) and plays many physiological roles, such as regulating osmotic pressure (16), acid-base balance (17) and vascular permeability (18). In addition, it is an antioxidant involved in the process of destroying oxygen radicals produced during oxidative stress (18).
Recent researches have shown that BUN-to-albumin ratio (BAR) can be used as a predictor of in-hospital mortality in corona virus disease 2019 (COVID-19) patients in the emergency department and aspiration pneumonia patients (19,20). Additionally, BAR is found to be a more accurate predictor than BUN or albumin levels alone. However, there is no research that employs the BAR to forecast ARF patient mortality and its applicability to a broader spectrum of ARF etiologies remains underexplored. Therefore, this study aimed to explore how BAR may be used to predict in-hospital and 30-day mortality in patients with ARF. We present this article in accordance with the STROBE reporting checklist (available at https://jtd.amegroups.com/article/view/10.21037/jtd-24-298/rc).
Methods
Study design
The Medical Information Mart for Intensive Care IV (MIMIC-IV) databases, built by the Massachusetts Institute of Technology and provided by Beth Israel Deaconess Medical Center (BIDMC), are large-scale, retrospective resources for critical care research. Patient data were anonymized in accordance with the Health Insurance Portability and Accountability Act (HIPAA) Safe Harbor Provision. This study utilized data retrieved from the MIMIC database (https://physionet.org/content/mimiciv/2.2/). After finishing the web-based training courses and the Protecting Human Research Participants examination (No. 36208651), we obtained permission to extract data from MIMIC-IV database. Because the study had a retrospective design, the need for patient consent was waived. The study was approved by the Medical Research Ethics Committee of Changde Hospital, Xiangya School of Medicine, Central South University (The First People’s Hospital of Changde City) (No. 2022-048-1). The study was conducted in accordance with the Declaration of Helsinki (as revised in 2013).
Patients
The analysis included all patients (≥18 years old) in the database diagnosed with ARF using the International Classification of Disease, Ninth Revision (ICD-9) and ICD-10 codes. The detailed ICD-9 codes were “51881,” and the detailed ICD-10 codes were “J960”, “J9600”, “J9601, and “J9602”. Patients who met any of the following criteria were excluded: (I) repeated hospital or ICU admissions, (II) ICU stays of less than 24 h, and (III) missing variables.
Data extraction
The following information was extracted for each patient: age at admission, sex, ethnicity, body mass index (BMI), comorbidities (including atrial fibrillation, myocardial infarction, congestive heart failure, chronic pulmonary disease, diabetes, renal disease, severe liver disease, malignant cancer, and metastatic solid tumor), first 24-hour Sequential Organ Failure Assessment (SOFA) score, Acute Physiology Score III (APSIII), and Oxford Acute Severity of Illness Score (OASIS) after admission to the ICU, vital signs [including temperature, pondus hydrogenii (PH), oxygen saturation (SO2), partial pressure of oxygen (PO2), and partial pressure of carbon dioxide (PCO2)], laboratory tests, mechanical ventilation (including non-invasive ventilation, invasive ventilation, high flow, and oxygen), vasopressor use, and 30-day mortality.
Statistical analysis
Continuous variables were presented as the mean (SD) or median [interquartile range (IQR)], and categorical variables were expressed as percentages. In two groups, when the baseline characteristics were continuous variables, Student’s t-test (normal distribution) or Mann-Whitney test (non-normal distribution) was used to compare them, and when the baseline characteristics were categorical variables, the Chi-squared test was used. For multiple groups, continuous variables were compared using the analysis of variance (ANOVA) and Kruskal Wallis rank sum test.
A generalized additive model was used to evaluate whether there was a non-linear association between BAR and 30-day mortality. Segmented regression, also known as piecewise regression, was applied. This method fits each interval with a different line segment by identifying one or more breakpoints where the slope of the relationship changes, thereby providing a better fit to the data. To ascertain whether a threshold exists, the log-likelihood ratio test was employed to compare the one-line (non-segmented) model to the segmented regression model. To investigate the independent effects of BAR on in-hospital and 30-day mortality, multivariate Cox analysis was used with crude and full models. Age, ethnicity, sex, severe liver disease, white blood cell (WBC) count, creatine level, platelet count, malignant cancer, SOFA score, vasoactive use, and mechanical ventilation were included as adjusting variables. Kaplan-Meier (K-M) methods and log-rank tests were used to assess the differences in survival rates between each group of patients with varied BAR at admission. Receiver operating characteristic (ROC) analysis was performed to determine the 30-day mortality predictive power of the BUN, albumin, and BAR levels. Subgroup analysis was conducted to determine whether there were any differences among the subgroups, and their interactions were examined.
The statistical software packages R (http://www.R-project.org) and Empower Stats (http://www.empowerstats.com version R.4.3.2) were used for data analyses. Statistical significance was considered when P value was <0.05.
Results
Characteristics of patients
A total of 9,734 patients with ARF were included in the study cohort according to the inclusion and exclusion criteria, with 7,153 survivors and 2,581 non-survivors stratified by 30-day mortality (Table 1). A flowchart for selecting the study participants is presented in Figure 1. The BAR in the survivor group was significantly higher than that in the non-survivor group [10.79 (6.25–18.81) vs. 7.35 (4.48–13.62), P<0.001]. In addition, age, ethnicity, BMI, comorbidities including atrial fibrillation, myocardial infarction, chronic pulmonary disease, renal disease, malignant cancer, severe liver disease, and metastatic solid tumor; severity scores including APSIII, SOFA, and OASIS; vital signs including PH, SO2, and temperature; laboratory tests including albumin, BUN, WBC, platelets, lactate dehydrogenase (LDH), lactate, creatinine, and PO2; and treatment received (mechanical ventilation and vasopressor use) were significantly different between the two groups.
Table 1
Characteristics | Survivor (n=7,153) | Non-survivor (n=2,581) | P |
---|---|---|---|
Age (years) | 64.77±16.82 | 70.51±14.98 | <0.001 |
Gender | 0.40 | ||
Male | 3,936 (55.03) | 1,445 (55.99) | |
Female | 3,217 (44.97) | 1,136 (44.01) | |
Ethnicity | <0.001 | ||
White | 4,807 (67.20) | 1,687 (65.36) | |
Black/African American | 810 (11.32) | 209 (8.10) | |
Asian | 194 (2.71) | 76 (2.94) | |
Unknown | 1,342 (18.76) | 609 (23.60) | |
BMI* (kg/m2) | 28.01 (24.06–33.23) | 27.14 (23.43–32.37) | <0.001 |
Comorbidity | |||
Atrial fibrillation | 2,166 (30.28) | 977 (37.85) | <0.001 |
Myocardial infarct | 1,262 (17.64) | 563 (21.81) | <0.001 |
Congestive heart failure | 2,707 (37.84) | 941 (36.46) | 0.21 |
Chronic pulmonary disease | 2,393 (33.45) | 782 (30.30) | 0.003 |
Renal disease | 1,744 (24.38) | 693 (26.85) | 0.01 |
Diabetes | 1,739 (24.31) | 599 (23.21) | 0.26 |
Malignant cancer | 988 (13.81) | 584 (22.63) | <0.001 |
Severe liver disease | 461 (6.44) | 329 (12.75) | <0.001 |
Metastatic solid tumor | 377 (5.27) | 362 (14.03) | <0.001 |
Charlson comorbidity | 5.93 (4.00–8.00) | 7.23 (5.00–9.00) | <0.001 |
Severity scores | |||
APSIII* | 48.00 (36.00–64.00) | 70.00 (51.00–91.00) | <0.001 |
SOFA* | 6.00 (4.00–9.00) | 9.00 (6.00–13.00) | <0.001 |
OASIS | 36.91±8.96 | 42.35±9.19 | <0.001 |
Vital signs | |||
PH* | 7.32 (7.24–7.38) | 7.28 (7.18–7.37) | <0.001 |
SO2 (%) | 82.79±17.95 | 79.54±19.97 | <0.001 |
Temperature (℃) | 36.84±0.92 | 36.58±1.09 | <0.001 |
PO2min* (mmHg) | 61.00 (40.00–89.00) | 55.00 (38.00–81.00) | <0.001 |
PCO2max* (mmHg) | 47.00 (40.00–57.00) | 47.00 (39.00–58.00) | 0.23 |
Albumin (g/dL) | 3.23±0.70 | 2.99±0.74 | <0.001 |
BUN* (mg/dL) | 22.00 (14.00–36.00) | 28.00 (18.00–47.00) | <0.001 |
WBC* (109 cells/L) | 11.60 (8.20–16.10) | 12.40 (8.40–17.70) | <0.001 |
Platelets* (109 cells/L) | 212.00 (150.00–282.00) | 192.00 (119.00–276.75) | <0.001 |
Glucose* (mg/dL) | 135.00 (109.00–178.00) | 140.00 (109.00–189.00) | 0.25 |
LDH* (U/mL) | 300.00 (225.00–448.00) | 373.50 (259.00–641.75) | <0.001 |
Lactate* (mmol/L) | 2.00 (1.30–3.30) | 2.80 (1.70–5.45) | <0.001 |
Creatinine* (mg/dL) | 1.10 (0.80–1.70) | 1.30 (0.90–2.10) | <0.001 |
BAR* (mg/g) | 7.35 (4.48–13.62) | 10.79 (6.25–18.81) | <0.001 |
Mechanical ventilation | <0.001 | ||
Non-invasive ventilation | 219 (3.15) | 37 (1.49) | |
Invasive ventilation | 4,316 (62.11) | 1,766 (71.21) | |
High flow | 876 (12.61) | 294 (11.85) | |
Oxygen | 1,538 (22.13) | 383 (15.44) | |
Vasopressor use | 313 (4.38) | 223 (8.64) | <0.001 |
Continuous variables conforming to normal distribution are expressed as mean ± standard deviation, whereas those not adhering to normal distribution are presented as median (interquartile range). Categorical variables are expressed as n (percentage). Skewed variables are marked with an asterisk. BMI, body mass index; ASPSIII, acute physiology score III; SOFA, sequential organ failure assessment; OASIS, Oxford Acute Severity of Illness Score; PH, pondus hydrogenii; SO2, oxygen saturation; PO2, partial pressure of oxygen; PCO2, partial pressure of carbon dioxide; BUN, blood urea nitrogen; WBC, white blood cell; LDH, lactate dehydrogenase; BAR, blood urea nitrogen-to-albumin ratio.
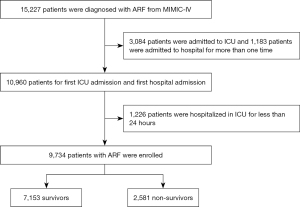
Association between BAR and clinical outcome in ARF patients
Table S1 displays the results of univariate Cox regression analysis for 30-day mortality. Age, comorbidities including malignant cancer and severe liver disease, albumin, BUN, creatinine, WBC, SOFA, platelets, vasopressor use, and BAR were significantly associated with 30-day mortality in patients with ARF.
We divided all patients into several groups based on the tertile of BAR and the threshold determined from the segmented regression model (results of threshold analysis are provided in Table S2). Table 2 displays the clinical outcomes of the subjects with different BAR values. Patients with high BAR had longer in-hospital and ICU stays and higher in-hospital and 30-day mortality rates.
Table 2
Exposure | Time in hospital (days) | Time in ICU (days) | In-hospital mortality | 30-day mortality | |||||||
---|---|---|---|---|---|---|---|---|---|---|---|
Median (IQR) | P value | Median (IQR) | P value | n (%) | P value | n (%) | P value | ||||
BAR tertile | 0.001 | <0.001 | <0.001 | <0.001 | |||||||
Low | 9.82 (5.35–17.71) | 4.30 (2.26–9.05) | 360 (19.81) | 259 (14.25) | |||||||
Middle | 10.78 (5.90–18.80) | 4.64 (2.44–9.08) | 529 (29.08) | 356 (19.57) | |||||||
High | 11.45 (5.99–19.80) | 4.98 (2.69–9.77) | 693 (38.08) | 500 (27.47) | |||||||
BAR categorical | 0.02 | <0.001 | <0.001 | <0.001 | |||||||
≤11.76 mg/g | 10.28 (5.70–18.21) | 4.49 (2.31–9.07) | 857 (23.99) | 594 (16.63) | |||||||
>11.76 mg/g | 11.42 (5.92–19.77) | 4.97 (2.69–9.76) | 725 (38.48) | 521 (27.65) |
ARF, acute respiratory failure; BAR, blood urea nitrogen-to-albumin ratio; ICU, intensive care unit; IQR, interquartile range.
We demonstrated the relationship between baseline BAR and the risk of 30-day mortality using smooth curve fitting (Figure 2). Taking BAR as a continuous variable, the association between baseline BAR and the risk of 30-day mortality was non-linear with a significant inflection point (11.76); after adjusting for age, sex, ethnicity, SOFA score, and other variables, the tendency still existed.
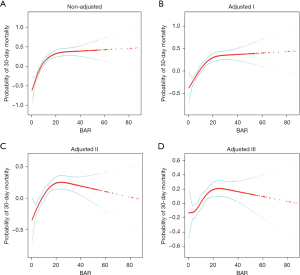
This finding was further evaluated using multivariable Cox regression analysis, which is displayed in Table 3. The unadjusted hazard ratio (HR) [95% confidence interval (CI)] of BAR as a continuous variable was 1.02 (95% CI: 1.01–1.02). After model I was adjusted for age, sex, and ethnicity, the hazard ratio was 1.02 (95% CI: 1.01–1.02). After model II was adjusted for age, sex, ethnicity, comorbidities including malignant cancer and severe liver disease, creatine, WBC, platelets, SOFA score, mechanical ventilation, and vasoactive use, the hazard ratio was 1.01 (95% CI: 1.01–1.02). When BAR was assessed as tertiles, the unadjusted hazard ratio of the middle group (1.39; 95% CI: 1.22–1.59) and the high group (1.79; 95% CI: 1.57–2.03) was higher than that of patients in the low BAR group. After model I, the hazard ratio of the middle group (1.24; 95% CI: 1.08–1.42) and the high group (1.60; 95% CI: 1.40–1.82) was higher than that of the low BAR group. After model II, the hazard ratio of the middle group (1.03; 95% CI: 0.89–1.18) and the high group (1.28; 95% CI: 1.10–1.49) was higher than that of the low BAR group. When BAR was categorized to two groups according to threshold determined from segmented regression model, patients in the high BAR group (>11.76 mg/g) had significantly higher risk of 30-day mortality than those in the low BAR group (≤11.76 mg/g), with the unadjusted model (HR 1.54, 95% CI: 1.39–1.70), adjusted model I (HR 1.45, 95% CI: 1.31–1.60) and adjusted model II (HR 1.29, 95% CI: 1.15–1.45).
Table 3
Exposure | Non-adjusted | Adjust I | Adjust II | |||||
---|---|---|---|---|---|---|---|---|
HR (95% CI) | P value | HR (95% CI) | P value | HR (95% CI) | P value | |||
BUN | 1.01 (1.01, 1.01) | <0.001 | 1.01 (1.00, 1.01) | <0.0001 | 1.00 (1.00, 1.01) | <0.001 | ||
Albumin | 0.77 (0.72, 0.82) | <0.001 | 0.76 (0.71, 0.81) | <0.0001 | 0.87 (0.81, 0.94) | <0.001 | ||
BAR per 1sd | 1.02 (1.01, 1.02) | <0.001 | 1.02 (1.01, 1.02) | <0.0001 | 1.01 (1.01, 1.02) | <0.001 | ||
BAR tertile | ||||||||
Low (ref) | 1.0 | 1.0 | 1.0 | |||||
Middle | 1.39 (1.22, 1.59) | <0.001 | 1.24 (1.08, 1.42) | 0.0024 | 1.03 (0.89, 1.18) | 0.71 | ||
High | 1.79 (1.57, 2.03) | <0.001 | 1.60 (1.40, 1.82) | <0.0001 | 1.28 (1.10, 1.49) | 0.002 | ||
BAR categorical | ||||||||
≤11.76 mg/g (ref) | 1.0 | 1.0 | 1.0 | |||||
>11.76 mg/g | 1.54 (1.39, 1.70) | <0.001 | 1.45 (1.31, 1.60) | <0.0001 | 1.29 (1.15, 1.45) | <0.001 |
Adjusted I for age, gender and ethnicity. Adjusted II for age, gender and ethnicity, malignant cancer, severe liver disease, creatine, WBC, platelets, SOFA, mechanical ventilation and vasoactive use. BAR, blood urea nitrogen-to-albumin ratio; HR, hazard ratio; CI, confidence interval; BUN, blood urea nitrogen; ref, reference; WBC, white blood cell; SOFA, sequential organ failure assessment.
The ROC curve for predicting 30-day mortality of ARF patients is provided in Table S3 and Figure S1. The area under the receiver operating characteristic curve (AUC) for the BAR was 0.704 (95% CI: 0.692–0.714) with 61.8% sensitivity and 69.2% specificity. As for BUN, its AUC value was found to be 0.647 (95% CI: 0.634–0.659) with 59.4% sensitivity and 61.9% specificity. For Albumin, its AUC was found to be 0.641 (95% CI: 0.629–0.653) with 58.2% sensitivity and 62.9% specificity.
The Kaplan-Meier curve demonstrated that ARF patients had higher 30-day all-cause mortality rates when they had higher BAR levels (>11.76 mg/g). The log-rank Chi-square was 74.051, with a highly significant P value <0.001. Furthermore, a significant difference was found when the BAR was divided into tertiles (low, middle, and high groups), yielding a log-rank Chi-square of 82.513 and a P value <0.001, as shown in Figure 3.
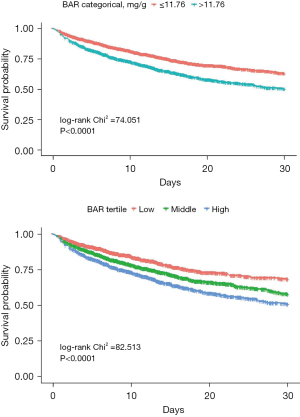
Subgroup analysis and interaction
Subgroup analyses for the relationship between BAR and 30-day all-cause mortality were performed according to age, sex, ethnicity, and comorbidities including malignant cancer, severe liver disease, renal disease, chronic pulmonary disease, congestive heart failure, vasopressor use, and mechanical ventilation. The association between BAR and 30-day mortality in patients with ARF was not observed in the severe liver disease group [odds ratio (OR) 1.01, 95% CI: 0.99–1.02, P=0.27] and vasopressor use group (OR 1.00, 95% CI: 0.98–1.02, P=0.91). In addition, no significant interactions were found for age, sex, ethnicity, comorbidities, mechanical ventilation, or vasopressor use (Table 4).
Table 4
Subgroups | No. of patients | HR (95% CI) | P value | P value for interaction |
---|---|---|---|---|
Age (years) | 0.89 | |||
<65 | 2,595 | 1.01 (1.00, 1.02) | 0.04 | |
≥65 | 2,861 | 1.02 (1.01, 1.03) | <0.001 | |
Gender | 0.83 | |||
Male | 3,133 | 1.02 (1.01, 1.03) | <0.001 | |
Female | 2,323 | 1.02 (1.01, 1.03) | <0.001 | |
Ethnicity | 0.78 | |||
White | 3,516 | 1.01 (1.01, 1.02) | <0.001 | |
Black/African American | 551 | 1.02 (1.00, 1.04) | 0.03 | |
Asian | 158 | 1.03 (1.00, 1.06) | 0.07 | |
Unknown | 1,231 | 1.01 (1.00, 1.02) | 0.02 | |
Congestive heart failure | 0.40 | |||
No | 3,506 | 1.02 (1.01, 1.02) | <0.001 | |
Yes | 1,950 | 1.01 (1.00, 1.02) | 0.003 | |
Renal disease | 0.12 | |||
No | 4,083 | 1.01 (1.01, 1.02) | <0.001 | |
Yes | 1,373 | 1.01 (1.00, 1.02) | 0.003 | |
Severe liver disease | 0.57 | |||
No | 4,813 | 1.02 (1.01, 1.02) | <0.001 | |
Yes | 643 | 1.01 (0.99, 1.02) | 0.27 | |
Malignant cancer | 0.19 | |||
No | 4,596 | 1.02 (1.01, 1.02) | <0.001 | |
Yes | 860 | 1.01 (1.00, 1.02) | 0.007 | |
Chronic pulmonary disease | 0.48 | |||
No | 3,870 | 1.01 (1.01, 1.02) | <0.001 | |
Yes | 1,586 | 1.02 (1.01, 1.03) | <0.001 | |
Mechanical ventilation | 0.09 | |||
No | 1,801 | 1.03 (1.02, 1.04) | <0.001 | |
Yes | 3,655 | 1.01 (1.00, 1.02) | 0.01 | |
Vasopressor use | 0.06 | |||
No | 5,104 | 1.02 (1.01, 1.02) | <0.001 | |
Yes | 352 | 1.00 (0.98, 1.02) | 0.91 |
BAR, blood urea nitrogen-to-albumin ratio; WBC, white blood cell; SOFA, sequential organ failure assessment; HR, hazard risk; CI, confidence interval.
Discussion
The findings of this study indicate a significant positive association between BAR and 30-day mortality in patients with ARF across various etiologies, underscoring its potential as a universal biomarker in critically ill patients. This correlation persisted after adjusting for confounding factors using multivariate Cox regression analysis. Compared with patients with low BAR, those with high BAR showed a significantly higher risk of 30-day mortality.
Common indicators used to evaluate renal function include estimation of glomerular filtration rate, blood creatinine, and BUN (21). In critically ill patients, who are in a state of high protein catabolism (22), the increase in BUN is more pronounced than that in serum creatinine, suggesting that BUN has additional predictive value over serum creatinine (23). High BUN, indicating unfavorable neurohormonal activation and impaired cardiorenal function, is generally considered an ominous sign in various diseases (24,25). Patients with sepsis (26,27) and Escherichia coli bacteremia (28) have a higher risk of death when they have higher BUN levels. In addition, it performed well as one of the parameters in the BAP65 (29) and CURB65 (30) models, which are frequently used to predict the probability of CAP mortality.
The significance of albumin in clinical practice is often limited to that of an oncotic agent and a presumptive sign of undernutrition. However, serum albumin demonstrates more clinical significance by binding with bilirubin, bile acids, fatty acids, tryptophan, calcium and medicines (31). Additionally, it accounts for approximately three-quarters of the antioxidant capacity of plasma, making it the most significant extracellular antioxidant in terms of quantity (32). Spontaneous increases or decreases in serum albumin levels, together with an associated shrinkage of rising total body water, are useful indicators of recovery or worsening health in critical illness, respectively. Elderly people with hypoalbuminemia have a higher chance of developing pneumonia outside hospitals (33) and there are more CAP patients who need intensive care (34).
Recent researches have shown that BAR, rather than serum albumin or BUN levels alone, can predict mortality and illness severity more precisely in individuals with CAP and aspiration pneumonia (20,35). Furthermore, studies revealed that BAR was related to survival outcomes in hospital-acquired pneumonia (36), elderly patients who visited the emergency department (37), moderate-to-severe COVID-19 pneumonia (38), acute pulmonary embolism (39), and chronic heart failure (40), among various other diseases. However, no study has focused on the predictive role of the BAR in ARF. Consistent with previous studies, in this study we found that elevated BAR was independently linked to higher in-hospital and 30-day mortality rates in patients with ARF. However, the cut-off point was different. In our study we found that BAR >11.76 mg/g was an independent predictor of mortality in ARF patients while Dundar ZD and colleagues revealed that elderly patients in the emergency department had a higher risk of in-hospital mortality when BAR >6.25 mg/g (37). This might be due to the difference of oxidative stress state and the severity of the disease between them.
Nevertheless, there are some limitations in this study. First, as a retrospective cohort study, it was impossible to account for every confounder. Although we accounted for known confounders, some unmeasured factors may still have an impact on our findings. Second, we only included BUN and albumin records of patients at their first admission, so that the prognostic impact of dynamic changes in BAR was still unclear. Third, because this was a single-center study, caution should be exercised when extrapolating the findings to other populations and areas since racial differences may have an impact on the result.
Conclusions
In conclusion, after controlling for the potential confounding variables, a high BAR was found to be linked to a higher risk of in-hospital and 30-day mortality. In patients with ARF, BAR is a straightforward and possibly useful prognostic biomarker.
Acknowledgments
Funding: This work was supported by
Footnote
Reporting Checklist: The authors have completed the STROBE reporting checklist. Available at https://jtd.amegroups.com/article/view/10.21037/jtd-24-298/rc
Peer Review File: Available at https://jtd.amegroups.com/article/view/10.21037/jtd-24-298/prf
Conflicts of Interest: All authors have completed the ICMJE uniform disclosure form (available at https://jtd.amegroups.com/article/view/10.21037/jtd-24-298/coif). The authors have no conflicts of interest to declare.
Ethical Statement:
Open Access Statement: This is an Open Access article distributed in accordance with the Creative Commons Attribution-NonCommercial-NoDerivs 4.0 International License (CC BY-NC-ND 4.0), which permits the non-commercial replication and distribution of the article with the strict proviso that no changes or edits are made and the original work is properly cited (including links to both the formal publication through the relevant DOI and the license). See: https://creativecommons.org/licenses/by-nc-nd/4.0/.
References
- Stefan MS, Shieh MS, Pekow PS, et al. Epidemiology and outcomes of acute respiratory failure in the United States, 2001 to 2009: a national survey. J Hosp Med 2013;8:76-82. [Crossref] [PubMed]
- Vincent JL, Akça S, De Mendonça A, et al. The epidemiology of acute respiratory failure in critically ill patients Chest 2002;121:1602-9. [Crossref] [PubMed]
- Ferreyro BL, Munshi L. Causes of acute respiratory failure in the immunocompromised host. Curr Opin Crit Care 2019;25:21-8. [Crossref] [PubMed]
- Carson SS, Cox CE, Holmes GM, et al. The changing epidemiology of mechanical ventilation: a population-based study. J Intensive Care Med 2006;21:173-82. [Crossref] [PubMed]
- Dziadzko MA, Novotny PJ, Sloan J, et al. Multicenter derivation and validation of an early warning score for acute respiratory failure or death in the hospital. Crit Care 2018;22:286. [Crossref] [PubMed]
- Portacci A, Diaferia F, Santomasi C, et al. Galectin-3 as prognostic biomarker in patients with COVID-19 acute respiratory failure. Respir Med 2021;187:106556. [Crossref] [PubMed]
- Santa Cruz A, Mendes-Frias A, Oliveira AI, et al. Interleukin-6 Is a Biomarker for the Development of Fatal Severe Acute Respiratory Syndrome Coronavirus 2 Pneumonia. Front Immunol 2021;12:613422. [Crossref] [PubMed]
- Cirik MO, Yenibertiz D. What are the prognostic factors affecting 30-day mortality in geriatric patients with respiratory failure in the Intensive Care Unit? Pak J Med Sci 2021;37:15-20. [PubMed]
- Pan SW, Kao HK, Yu WK, et al. Synergistic impact of low serum albumin on intensive care unit admission and high blood urea nitrogen during intensive care unit stay on post-intensive care unit mortality in critically ill elderly patients requiring mechanical ventilation. Geriatr Gerontol Int 2013;13:107-15. [Crossref] [PubMed]
- Chen J. Calculated decisions: SMART-COP score for pneumonia severity. Emerg Med Pract 2021;23:CD3-4. [PubMed]
- Labarère J, Schuetz P, Renaud B, et al. Validation of a clinical prediction model for early admission to the intensive care unit of patients with pneumonia. Acad Emerg Med 2012;19:993-1003. [Crossref] [PubMed]
- Carlos P, Gomes R, Coelho J, et al. CURB-65 and Long-Term Mortality of Community-Acquired Pneumonia: A Retrospective Study on Hospitalized Patients. Cureus 2023;15:e36052. [Crossref] [PubMed]
- Solela G, Yimer YS. Clinical characteristics, treatment patterns, and outcomes of hospitalized patients with acute heart failure in central Ethiopia: a retrospective observational study. BMC Cardiovasc Disord 2024;24:241. [Crossref] [PubMed]
- Wei C, Wang X, He D, et al. Clinical profile analysis and nomogram for predicting in-hospital mortality among elderly severe community-acquired pneumonia patients: a retrospective cohort study. BMC Pulm Med 2024;24:38. [Crossref] [PubMed]
- Cabrerizo S, Cuadras D, Gomez-Busto F, et al. Serum albumin and health in older people: Review and meta analysis. Maturitas 2015;81:17-27. [Crossref] [PubMed]
- Hankins J. The role of albumin in fluid and electrolyte balance. J Infus Nurs 2006;29:260-5. [Crossref] [PubMed]
- Krbec M, Koudelková K, Duška F. On acid-base effects of albumin. Curr Med Res Opin 2021;37:1515-6. [Crossref] [PubMed]
- Belinskaia DA, Voronina PA, Goncharov NV. Integrative Role of Albumin: Evolutionary, Biochemical and Pathophysiological Aspects. J Evol Biochem Physiol 2021;57:1419-48. [Crossref] [PubMed]
- Küçükceran K, Ayrancı MK, Girişgin AS, et al. The role of the BUN/albumin ratio in predicting mortality in COVID-19 patients in the emergency department. Am J Emerg Med 2021;48:33-7. [Crossref] [PubMed]
- Ryu S, Oh SK, Cho SU, et al. Utility of the blood urea nitrogen to serum albumin ratio as a prognostic factor of mortality in aspiration pneumonia patients. Am J Emerg Med 2021;43:175-9. [Crossref] [PubMed]
- Levey AS, James MT. Acute Kidney Injury. Ann Intern Med 2017;167:ITC66-80. [Crossref] [PubMed]
- Sharma K, Mogensen KM, Robinson MK. Pathophysiology of Critical Illness and Role of Nutrition. Nutr Clin Pract 2019;34:12-22. [Crossref] [PubMed]
- Haines RW, Zolfaghari P, Wan Y, et al. Elevated urea-to-creatinine ratio provides a biochemical signature of muscle catabolism and persistent critical illness after major trauma. Intensive Care Med 2019;45:1718-31. [Crossref] [PubMed]
- Horiuchi Y, Aoki J, Tanabe K, et al. A High Level of Blood Urea Nitrogen Is a Significant Predictor for In-hospital Mortality in Patients with Acute Myocardial Infarction. Int Heart J 2018;59:263-71. [Crossref] [PubMed]
- Chen L, Chen L, Zheng H, et al. The association of blood urea nitrogen levels upon emergency admission with mortality in acute exacerbation of chronic obstructive pulmonary disease. Chron Respir Dis 2021;18:14799731211060051. [Crossref] [PubMed]
- Peng Q, Zhang L, Ai Y, et al. Epidemiology of acute kidney injury in intensive care septic patients based on the KDIGO guidelines. Chin Med J (Engl) 2014;127:1820-6. [Crossref] [PubMed]
- Li X, Zheng R, Zhang T, et al. Association between blood urea nitrogen and 30-day mortality in patients with sepsis: a retrospective analysis. Ann Palliat Med 2021;10:11653-63. [Crossref] [PubMed]
- Zou XL, Feng DY, Wu WB, et al. Blood urea nitrogen to serum albumin ratio independently predicts 30-day mortality and severity in patients with Escherichia coli bacteraemia. Med Clin (Barc) 2021;157:219-25. [Crossref] [PubMed]
- Germini F, Veronese G, Marcucci M, et al. Validation of the BAP-65 score for prediction of in-hospital death or use of mechanical ventilation in patients presenting to the emergency department with an acute exacerbation of COPD: a retrospective multi-center study from the Italian Society of Emergency Medicine (SIMEU). Eur J Intern Med 2019;61:62-8. [Crossref] [PubMed]
- Chang CL, Sullivan GD, Karalus NC, et al. Predicting early mortality in acute exacerbation of chronic obstructive pulmonary disease using the CURB65 score. Respirology 2011;16:146-51. [Crossref] [PubMed]
- Soeters PB, Wolfe RR, Shenkin A. Hypoalbuminemia: Pathogenesis and Clinical Significance. JPEN J Parenter Enteral Nutr 2019;43:181-93. [Crossref] [PubMed]
- Bourdon E, Blache D. The importance of proteins in defense against oxidation. Antioxid Redox Signal 2001;3:293-311. [Crossref] [PubMed]
- Washio M, Kondo K, Fujisawa N, et al. Hypoalbuminemia, influenza vaccination and other factors related to the development of pneumonia acquired outside hospitals in southern Japan: A case-control study. Geriatr Gerontol Int 2016;16:223-9. [Crossref] [PubMed]
- Adnan M, Hashmat N, Latif M, et al. Hypoalbuminemia predicts intensive care need among adult inpatients with community acquired pneumonia: a cross sectional study. J Infect Dev Ctries 2018;12:636-41. [Crossref] [PubMed]
- Ugajin M, Yamaki K, Iwamura N, et al. Blood urea nitrogen to serum albumin ratio independently predicts mortality and severity of community-acquired pneumonia. Int J Gen Med 2012;5:583-9. [Crossref] [PubMed]
- Feng DY, Zhou YQ, Zou XL, et al. Elevated Blood Urea Nitrogen-to-Serum Albumin Ratio as a Factor That Negatively Affects the Mortality of Patients with Hospital-Acquired Pneumonia. Can J Infect Dis Med Microbiol 2019;2019:1547405. [Crossref] [PubMed]
- Dundar ZD, Kucukceran K, Ayranci MK. Blood urea nitrogen to albumin ratio is a predictor of in-hospital mortality in older emergency department patients. Am J Emerg Med 2021;46:349-54. [Crossref] [PubMed]
- Ata F, As AK, Engin M, et al. Can blood urea Nitrogen-to-Albumin ratio predict mortality in patients with moderate-to-severe COVID-19 pneumonia hospitalized in the intensive care unit? Rev Assoc Med Bras (1992) 2021;67:1421-6. [Crossref] [PubMed]
- Fang J, Xu B. Blood Urea Nitrogen to Serum Albumin Ratio Independently Predicts Mortality in Critically Ill Patients With Acute Pulmonary Embolism. Clin Appl Thromb Hemost 2021;27:10760296211010241. [Crossref] [PubMed]
- Lin Z, Zhao Y, Xiao L, et al. Blood urea nitrogen to serum albumin ratio as a new prognostic indicator in critical patients with chronic heart failure. ESC Heart Fail 2022;9:1360-9. [Crossref] [PubMed]