Correlation of sputum inflammatory markers with severity and blood inflammatory markers in bronchiectasis
Highlight box
Key findings
• Baseline sputum interleukin-1beta (IL-1β) level had significant moderate positive correlation with baseline blood high sensitivity C-reactive protein level, FACED score and E-FACED score. And baseline sputum tumor necrosis factor-alpha (TNF-α) level was found to have significant moderate positive correlation with baseline FACED score.
What is known and what is new?
• Sputum biomarkers have been investigated in their association with severity and prognosis of bronchiectasis.
• This study is the first report of association and correlation between sputum IL-1β, interleukin-8, and TNF-α levels at stable state and clinical, spirometry, blood parameters, as well as prognostic scores.
What is the implication, and what should change now?
• The findings from this study suggest the clinical value of the severity of airway inflammation as measured by sputum cytokines in stable state bronchiectasis.
Introduction
Bronchiectasis is characterized by chronic airway inflammation, abnormal mucus clearance and bacterial colonization. The end result is progressive airway destruction and distortion (1-3). This destructive process perpetuates in a vicious cycle even when the initial insult has subsided, which is commonly due to an infective process like tuberculosis (4-6).
In recent years, accumulated evidence suggested a central role of airway inflammation and immune dysregulation in the evolution of bronchiectasis (7). The most well reported airway inflammation in bronchiectasis is neutrophilic inflammation. It is characterized by abundance of neutrophils in sputum, bronchoalveolar lavage fluid and bronchial biopsy from patients with bronchiectasis (8). Various pro-inflammatory cytokines were shown to be responsible in the recruitment and trafficking of neutrophils to the bronchiectatic airways. These cytokines include interleukin-1beta (IL-1β) (9), interleukin-8 (IL-8) (10,11), tumor necrosis factor-alpha (TNF-α) (12), and leukotriene (LT) B4 (13). There was also in vitro model suggesting that sputum from patients with bronchiectasis could stimulate IL-6 production from normal human bronchial epithelial cells, mediated via TNF-α (14). Studies suggested the involvement of Th17 immunity in bronchiectasis, in which Th17-polarized CD4 T cells can respond to bacteria (especially Pseudomonas aeruginosa) in bronchiectatic airways by elaboration of IL-17, leading to downstream IL-8 release from airway epithelial cells, neutrophil chemotaxis, mucus hypersecretion and formation of ectopic lymphoid follicles (3). This IL-17 driven pathway can further aggravate the vicious cycle in bronchiectasis. Studies demonstrated that airway neutrophilic inflammation as indicated by sputum neutrophil count at stable state was associated with worse lung function as measured forced expiratory volume in one second (FEV1), and disease severity as measured by bronchiectasis severity score (BSI) (15,16). Sputum elastase was also shown to be significantly correlated with 24-hour sputum volume, extent of bronchiectasis, FEV1 as percentage predicted, as well as sputum leukocyte count in stable bronchiectasis (5). Among patients who had Pseudomonas aeruginosa colonization, they had greater sputum neutrophilia and volume, with lower FEV1 and FEV1/forced vital capacity (FVC) ratio (15,16).
While different sputum cytokines and inflammatory markers can be measured in sputum sample, their clinical relevance is yet to be determined. A pediatric study suggested that symptom scores correlated with FEV1 and sputum IL-8 levels. There was also a significant correlation between high resolution computed tomography (HRCT) severity scores and symptoms, FEV1, sputum IL-8 and TNF-α levels (17).
There was one study suggesting that sputum cell counts, IL-8 and IL-13 correlated with distinct clinical and functional measurements of disease severity. In that study, they showed that sputum IL-8 levels were higher in subjects with frequent exacerbations. It also correlated with sputum neutrophil level, the extent of bronchiectasis in HRCT and post-bronchodilator FEV1. On the other hand, sputum IL-13 level was shown to be correlated with sputum eosinophil level and bronchodilator reversibility (18). However, the value of sputum IL-1β and TNF-α were not assessed in previous studies. They also did not assess the correlation of the sputum inflammatory cytokines level with FACED (FEV1, age, chronic colonisation by Pseudomonas aeruginosa, radiological extension and dyspnoea)/E-FACED (FACED plus exacerbations) score, as well as blood inflammatory markers.
There has been limited clinical data on how various sputum cytokines and inflammatory markers correlate with the disease severity and other blood inflammatory markers. As such, we aim to investigate the association between sputum IL-1β, IL-8 and TNF-α level at clinical stable and the severity of bronchiectasis, as well as the level of blood inflammatory markers. We present this article in accordance with the STROBE reporting checklist (available at https://jtd.amegroups.com/article/view/10.21037/jtd-24-573/rc).
Methods
This was a prospective clinical study conducted in Hong Kong. Patients with bronchiectasis that have follow up in the adult Respiratory Specialty Clinic at Queen Mary Hospital, Hong Kong were recruited from 1st October to 31st December 2021. Subjects above the age of 18 years with confirmed diagnosis of bronchiectasis based on (HRCT were included). Patients with co-existing systemic inflammatory diseases were excluded.
Fresh sputum sample was collected from the patients at clinical stable state and stored at −70 °C within 15 min of collection until ultracentrifugation (100,000 g for 30 min at 4 °C) to obtain the sol phase used for enzyme-linked immunosorbent assay of cytokines including IL-1β and IL-8, TNF-α levels using commercially available kits (19). Samples were added to a 96-well plate (R&D Systems, Minneapolis, MN, USA) coated with monoclonal antibody against one of the cytokines and incubated for 2 hours at room temperature. The samples were then removed and washed three times with buffer, and an enzyme-linked antibody specific for a particular cytokine is added to each well and incubated at room temperature for 2 hours. After a final wash to remove all unbound antibody, a substrate solution was added to each well and incubated for 20 min before the reaction is terminated by adding a “stop solution” (1 M sulfuric acid). The optical density was determined by using a plate reader at 450 nm that reflects the concentration of the cytokines. Experiments were conducted in triplicates.
The co-primary outcomes were the correlation of sputum IL-1β, IL-8 and TNF-α levels with FACED score, E-FACED score; modified Medical Research Council (mMRC) dyspnoea scale, number of exacerbations in the subsequent 1 year; spirometry values (FEV1 in litre and as percentage predicted); blood neutrophil count, lymphocyte count, neutrophil to lymphocyte ratio (NLR), C-reactive protein (CRP) and high sensitivity C-reactive protein (hs-CRP) level at stable state. The study was conducted in accordance with the Declaration of Helsinki (as revised in 2013). The study was approved by the Institutional Review Board of the University of Hong Kong and Hospital Authority Hong Kong West Cluster (No. UW19-624). Informed consents were obtained.
The sample size was calculated with reference to a previously published study on the correlation with inflammatory markers in induced sputum and clinical characteristics in children with bronchiectasis (17). In that study, symptom scores correlated with sputum IL-8 levels (r=0.67, P<0.05). A sample size of 17 would have α of 0.05, β of 0.15 and sample correlation of 0.67.
Statistical analysis
Numeric data based on demographic features and sputum cytokines results were presented as mean ± standard deviation (SD) for the values that were normally distributed. The values were presented as median and interquartile range (IQR) if they were not normally distributed. The categorical variables were presented as frequencies and percentages. The relationship between sputum IL-1β, IL-8, TNF-α levels and mMRC dyspnoea scale, FACED score, E-FACED score, number of exacerbations in the follow-up period of 1 year, spirometry values, blood inflammatory markers levels were assessed using the Pearson’s correlation coefficient metrics. Statistical significance was determined at the level of P≤0.05. All statistical analyses were performed using the 26th version of SPSS statistical package.
Results
A total of 44 Chinese patients with bronchiectasis managed in Queen Mary Hospital were included.
Baseline characteristics
The mean age was 70.2±8.6 years. There were more females (68.2%) and never-smokers (86.4%). The mean body mass index (BMI) was 22.3±4.2 kg/m2. The mean FEV1 was 1.73±0.65 L (90%±24%). Multilobar involvement, defined as those with disease in more than 2 lobes, were seen in 38.6% of the patients. The median mMRC dyspnea scale was 1 (IQR, 1–2) with 4 (9.1%) of the patients having mMRC dyspnea scale ≥2, which will be scored 1 in FACED and E-FACED score. The median FACED and E-FACED score were 3 (IQR, 2–4) and 3 (IQR, 2–4) respectively. The median sputum IL-1β and IL-8, TNF-α levels at baseline were 2.10 (IQR, 0.81–4.44) ng/mL, 13.48 (IQR, 6.43–22.0) ng/mL and 6.86 (IQR, 1.35–19.35) pg/mL, respectively. The results are summarized in Table 1.
Table 1
Patient characteristics | Values |
---|---|
Age (years) | 70.2±8.6 |
Female | 30 (68.2) |
Never-smokers | 38 (86.4) |
Body mass index (kg/m2) | 22.3±4.2 |
FEV1 (L) | 1.73±0.65 |
FEV1 (% predicted) | 90±24 |
FVC (L) | 2.44±0.84 |
FVC (% predicted) | 95±19 |
Extent of involvement ≥3 lobes | 17 (38.6) |
Baseline mMRC dyspnoea scale | 1 [1–2] |
Number of patients with mMRC dyspnoea scale ≥2 | 4 (9.1) |
Pseudomonas aeruginosa colonization | 16 (36.4) |
Number of exacerbations in the past 1 year | 7 (15.9) |
Number of hospitalized exacerbations in the past 1 year | 2 (4.5) |
Exacerbations in the follow period of 1 year | 14 (31.8) |
Hospitalized exacerbations in the follow period of 1 year | 8 (18.2) |
Baseline blood leucocyte count (× cells/µL) | 6.96±2.43 |
Baseline blood neutrophil count (× cells/µL) | 4.41±2.31 |
Baseline blood lymphocyte (× cells/µL) | 1.83±0.54 |
Baseline neutrophil to lymphocyte ratio | 2.27 [1.57–3.12] |
Baseline hs-CRP level (mg/dL) | 0.57±0.15 |
Baseline FACED score | 3 [2–4] |
Baseline E-FACED score | 3 [2–4] |
Baseline sputum IL-1β level (ng/mL) | 2.10 [0.81–4.44] |
Baseline sputum IL-8 level (ng/mL) | 13.48 [6.43–22.0] |
Baseline sputum TNF-α level (pg/mL) | 6.86 [1.35–19.35] |
Date are presented as mean ± standard deviation or n (%) or median [interquartile range]. FEV1, forced expiratory volume in one second; FVC, forced vital capacity; mMRC, modified Medical Research Council; hs-CRP, high sensitivity C-reactive protein; FACED, FEV1, age, chronic colonisation by Pseudomonas aeruginosa, radiological extension and dyspnoea; E-FACED, FACED plus exacerbations; IL-1β, interleukin-1beta; IL-8, interleukin-8; TNF-α, tumor necrosis factor-alpha.
Sputum IL-1β
Baseline sputum IL-1β level was found to have significant positive correlation with baseline blood hs-CRP level, number of all bronchiectasis exacerbation and hospitalized bronchiectasis exacerbation in the follow-up of 1 year, baseline mMRC dyspnoea scale, FACED score, E-FACED score, baseline blood neutrophil count and NLR with Pearson correlation coefficient (r) of 0.529 (P=0.001). 0.400 (P=0.01), 0.427 (P=0.008), 0.426 (P=0.004), 0.446 (P=0.004), 0.399 (P=0.01), 0.318 (P=0.04) and 0.461 (P=0.002). It had negative correlation with baseline FEV1 in litre and percentage predicted, as well as baseline blood lymphocyte count with r of −0.348 (P=0.03), −0.441 (P=0.005), −0.307 (P=0.04), respectively. The results are summarized in Table 2 and Figures 1,2.
Table 2
Clinical and laboratory parameters | Pearson correlation coefficient | P value |
---|---|---|
FEV1 | −0.348† | 0.03* |
FEV1 (% predicted) | −0.441‡ | 0.005* |
Baseline mMRC dyspnoea scale | 0.426‡ | 0.004* |
Number of exacerbations in the follow period of 1 year | 0.400‡ | 0.01* |
Number of hospitalized exacerbations in the follow period of 1 year | 0.427‡ | 0.008* |
Baseline blood leucocyte count | 0.271 | 0.08 |
Baseline blood neutrophil count | 0.318† | 0.04* |
Baseline blood lymphocyte | −0.307† | 0.04* |
Baseline neutrophil to lymphocyte ratio | 0.461‡ | 0.002* |
Baseline hs-CRP level | 0.529‡ | 0.001* |
Baseline FACED score | 0.446‡ | 0.004* |
Baseline E-FACED score | 0.399† | 0.01* |
*, statistically significant; †, weak correlation; ‡, moderate correlation. IL-1β, interleukin-1beta; FEV1, forced expiratory volume in one second; mMRC, modified Medical Research Council; hs-CRP, high sensitivity C-reactive protein; FACED, FEV1, age, chronic colonisation by Pseudomonas aeruginosa, radiological extension and dyspnoea; E-FACED, FACED plus exacerbations.
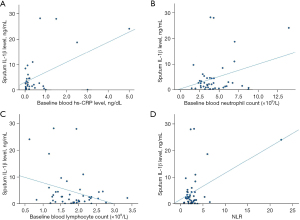
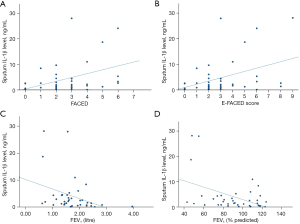
Sputum IL-8
Baseline sputum IL-8 level was found to have significant moderate positive correlation with baseline FACED and E-FACED score with r of 0.574 (P<0.001) and 0.539 (P<0.001) respectively. It has weak positive correlation with baseline mMRC dyspnoea scale with r of 0.309 (P=0.04) and moderate negative correlation with baseline FEV1 in percentage predicted with r of −0.440 (P=0.005). The results are summarized in Table 3 and Figure 3.
Table 3
Clinical and laboratory parameters | Pearson correlation coefficient | P value |
---|---|---|
FEV1 | −0.215 | 0.19 |
FEV1 (% predicted) | −0.440‡ | 0.005* |
Baseline mMRC dyspnoea scale | 0.309† | 0.04* |
Number of exacerbations in the follow period of 1 year | 0.232 | 0.17 |
Number of hospitalized exacerbations in the follow period of 1 year | 0.233 | 0.17 |
Baseline blood leucocyte count | 0.145 | 0.35 |
Baseline blood neutrophil count | 0.148 | 0.34 |
Baseline blood lymphocyte | −0.102 | 0.51 |
Baseline neutrophil to lymphocyte ratio | 0.215 | 0.16 |
Baseline hs-CRP level | 0.219 | 0.21 |
Baseline FACED score | 0.574‡ | <0.001* |
Baseline E-FACED score | 0.539‡ | <0.001* |
*, statistically significant; †, weak correlation; ‡, moderate correlation. IL-8, interleukin-8; FEV1, forced expiratory volume in one second; mMRC, modified Medical Research Council; hs-CRP, high sensitivity C-reactive protein; FACED, FEV1, age, chronic colonisation by Pseudomonas aeruginosa, radiological extension and dyspnoea; E-FACED, FACED plus exacerbations.
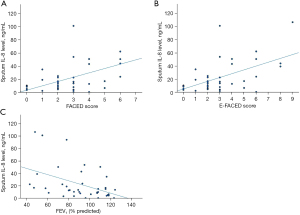
Sputum TNF-α
Baseline sputum TNF-α level was found to have significant moderate positive correlation with baseline FACED score, with baseline E-FACED and mMRC dyspnoea scale with r of 0.520 (P<0.001), 0.477 (P=0.002) and r of 0.335 (P=0.03) respectively. It has negative correlation with baseline FEV1 percentage predicted with r of −0.445 (P=0.005). The results are summarized in Table 4 and Figure 4.
Table 4
Clinical and laboratory parameters | Pearson correlation coefficient | P value |
---|---|---|
FEV1 | −0.312 | 0.053 |
FEV1 (% predicted) | −0.445‡ | 0.005* |
Baseline mMRC dyspnoea scale | 0.335† | 0.03* |
Number of exacerbations in the follow period of 1 year | 0.056 | 0.74 |
Number of hospitalized exacerbations in the follow period of 1 year | 0.005 | 0.98 |
Baseline blood leucocyte count | −0.051 | 0.74 |
Baseline blood neutrophil count | −0.040 | 0.80 |
Baseline blood lymphocyte | −0134 | 0.39 |
Baseline neutrophil to lymphocyte ratio | 0.020 | 0.90 |
Baseline hs-CRP level | 0.073 | 0.68 |
Baseline FACED score | 0.520‡ | <0.001* |
Baseline E-FACED score | 0.477‡ | 0.002* |
*, statistically significant; †, weak correlation; ‡, moderate correlation. TNF-α, tumor necrosis factor-alpha; FEV1, forced expiratory volume in one second; mMRC, modified Medical Research Council; hs-CRP, high sensitivity C-reactive protein; FACED, FEV1, age, chronic colonisation by Pseudomonas aeruginosa, radiological extension and dyspnoea; E-FACED, FACED plus exacerbations.
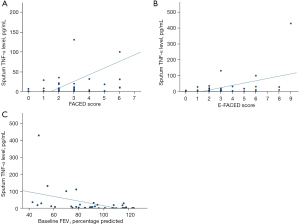
Sputum IL-1β, IL-8 and TNF-α levels
Sputum IL-1β, IL-8 and TNF-α levels were found to be strongly and significantly correlated with each other. The r for IL-1β and IL-8 was 0.715 (P<0.001), 0.748 (P<0.001) for IL-1β and TNF-α, 0.654 (P<0.001) for IL-1β and TNF-α.
Discussion
To our best knowledge, it is the first report of the association and correlation between sputum inflammatory markers with IL-1β, IL-8 and TNF-α level at clinical stable and severity of bronchiectasis and the level of blood inflammatory markers. The higher the sputum inflammatory markers are, the more severe the disease, as evidenced by higher mMRC dyspnea scale, lower FEV1, higher FACED score and E-FACED score; as well as higher blood inflammatory markers including CRP, hs-CRP and NLR. The findings from this study suggest the possible clinical value of measuring these sputum cytokines in stable state bronchiectasis to reflect the severity of airway inflammation with clinical correlations.
As a disease with chronic neutrophilic inflammation, the role of sputum neutrophil count and neutrophil elastase level had been studied and shown to be associated with more severe disease. However, other sputum cytokines including IL-1β, IL-8 and TNF-α are less well studied. This could partly be related to the cost and availability of these tests. But the role of sputum inflammatory markers shall not be underestimated as they are directly reflecting the degree of airway inflammation, while blood inflammatory markers could be affected by non-respiratory tract conditions including infection and inflammatory disorders. Our result suggested that these sputum cytokines to be good inflammatory markers to correlate well with various markers of disease severity, including blood inflammatory markers, spirometric values, number of bronchiectasis exacerbation and FACED/E-FACED score. The long-term prognostic value of these sputum cytokines, especially the variability at stable state, as well as the values and variability at acute exacerbation of bronchiectasis are worth exploring.
In the recent years, there have been growing interest in the research on phenotyping of airway diseases, especially with the use of blood and sputum inflammatory markers (20-22). The correlation with the blood and sputum inflammatory markers have also been extensively studied (23,24). However, similar studies are lacking in bronchiectasis. While there is limited evidence in some sputum cytokines such as sputum IL-8 and IL-13 and clinical/functional measurements of disease severity, the results were not comprehensive enough. Compared with other airway diseases, bronchiectasis is well known to be associated with chronic productive cough with significant amount of sputum. This makes the use of sputum cytokines to monitor disease severity and progress feasible. In other airway diseases such as asthma, induced sputum may be needed in order to measure sputum inflammatory markers level, which has the potential risks of bronchospasm. It also needs to be done by specially trained physiotherapists or nurses. This adds up to extra costs of measuring sputum cytokines values in asthma and chronic obstructive pulmonary disease (COPD). As a disease known to produce significant amount of sputum in the majority of patients, sputum cytokines can much easily be measured and the value of their level worth further research in larger scale study with prolonged follow-up period. The levels of sputum cytokines level may also help in phenotyping of bronchiectasis, which is one of the areas that is being studied more extensively in recent years (25-27). Our study suggested that sputum IL-1β, IL-8 and TNF-α to be reliable markers that correlate with the clinical features in bronchiectasis that carry prognostic implications. Our finding also provides insights on how the use of various sputum cytokines correlates in the clinical features with prognostic implications in bronchiectasis. Apart from the three cytokines we examined, there are other sputum inflammatory markers that can be measured, and it is worthwhile to know about their role in bronchiectasis in future.
While these sputum inflammatory biomarkers are not yet widely used in clinical setting due to the availability issue, with further development of technology, they could emerge as potential biomarkers to monitor the clinical status of bronchiectasis. The measurement of these sputum biomarkers can reflect the degree of the airway inflammation in bronchiectasis, and serial measurement may allow the clinicians to know about the disease status. It may also allow clinicians to assess the response to treatment by monitoring the levels of the sputum inflammatory markers before and after started the treatment (28,29). Thinking one step further, the potential development of volatile organic compounds (VOCs) based disease monitoring might be possible, through the knowledge discovered in this study. Animal study suggested that 1-butanol and 1-pentanol were increased in TNF-α treated rats (30). Whether patients with elevated sputum TNF-α also had elevated 1-butanol, 1-pentanol and other VOCs would be an area that worth researchers to explore as it might bring about revolution in diagnostic measures by VOCs in bronchiectasis.
There are a few limitations in our study. Firstly, this study involved only a single centre. However, being a tertiary medical centre, the respiratory unit received referrals from all other health care facilities across the territory. Patients diagnosed with bronchiectasis were managed in a designated bronchiectasis clinic in our centre. Secondly, the levels of sputum cytokines were measured at different times. But all of them were measured when the patients were at clinical stable state, so as for the blood tests in these patients. Their level shall be representative enough. In this study, while we demonstrated the significant correlation between sputum IL-1β, IL-8 and TNF-α level at clinical stable with the severity of bronchiectasis and other the level of blood inflammatory markers, the correlation was at best to be moderate. Ideally, a significant and strong correlation shall allow the implementation of the biomarkers to be used in clinical setting. One of the possible reasons for only moderate but not strong correlation was observed could be related to the different inflammatory phenotypes in bronchiectasis. In recent years, eosinophilic phenotypes become increasingly being reported in bronchiectasis, which has different behavior and prognosis with neutrophilic bronchiectasis (25,31-33). As sputum IL-1β, IL-8, and TNF-α are markers related to neutrophilic inflammation, it is possible that the level of these cytokines was elevated in a greater degree in neutrophilic than eosinophilic bronchiectasis. However, due to the limited sample size in this study, we were not able to further analyze the correlation among different phenotypes. A dedicated large-scale study shall be conducted to assess this correlation. Furthermore, heterogeneity of systemic inflammatory proteins not adequately explained by differences in disease aetiology or severity was observed in prior study, which could explain for this phenomenon (33).
Conclusions
Sputum IL-1β, IL-8, and TNF-α levels were shown to have significant correlation with various clinical, laboratory and spirometry parameters in bronchiectasis, as well as more severe disease as measured by FACED and E-FACED scores.
Acknowledgments
The data of this manuscript were presented as poster in the 27th Congress of the Asian Pacific Society of Respirology (APSR 2023).
Funding: The study was supported by
Footnote
Reporting Checklist: The authors have completed the STROBE reporting checklist. Available at https://jtd.amegroups.com/article/view/10.21037/jtd-24-573/rc
Data Sharing Statement: Available at https://jtd.amegroups.com/article/view/10.21037/jtd-24-573/dss
Peer Review File: Available at https://jtd.amegroups.com/article/view/10.21037/jtd-24-573/prf
Conflicts of Interest: All authors have completed the ICMJE uniform disclosure form (available at https://jtd.amegroups.com/article/view/10.21037/jtd-24-573/coif). W.C.K. reports that the study was supported by the Hong Kong College of Physicians Young Investigator Research Grant, Hong Kong College of Physicians. The other authors have no conflicts of interest to declare.
Ethical Statement: The authors are accountable for all aspects of the work in ensuring that questions related to the accuracy or integrity of any part of the work are appropriately investigated and resolved. The study was conducted in accordance with the Declaration of Helsinki (as revised in 2013). The study was approved by the Institutional Review Board of the University of Hong Kong and Hospital Authority Hong Kong West Cluster (No. UW19-624). Informed consents were obtained.
Open Access Statement: This is an Open Access article distributed in accordance with the Creative Commons Attribution-NonCommercial-NoDerivs 4.0 International License (CC BY-NC-ND 4.0), which permits the non-commercial replication and distribution of the article with the strict proviso that no changes or edits are made and the original work is properly cited (including links to both the formal publication through the relevant DOI and the license). See: https://creativecommons.org/licenses/by-nc-nd/4.0/.
References
- Giam YH, Shoemark A, Chalmers JD. Neutrophil dysfunction in bronchiectasis: an emerging role for immunometabolism. Eur Respir J 2021;58:2003157. [Crossref] [PubMed]
- King PT. The pathophysiology of bronchiectasis. Int J Chron Obstruct Pulmon Dis 2009;4:411-9. [Crossref] [PubMed]
- Bush A, Floto RA. Pathophysiology, causes and genetics of paediatric and adult bronchiectasis. Respirology 2019;24:1053-62. [Crossref] [PubMed]
- Wang H, Ji XB, Li CW, et al. Clinical characteristics and validation of bronchiectasis severity score systems for post-tuberculosis bronchiectasis. Clin Respir J 2018;12:2346-53. [Crossref] [PubMed]
- Vasconcelos A, Dias Rodrigues CF, Fonseca J, et al. Bronchiectasis secondary to pulmonary tuberculosis. Postgrad Med J 2021;97:335. [Crossref] [PubMed]
- Jordan TS, Spencer EM, Davies P. Tuberculosis, bronchiectasis and chronic airflow obstruction. Respirology 2010;15:623-8. [Crossref] [PubMed]
- Boyton RJ, Altmann DM. Bronchiectasis: Current Concepts in Pathogenesis, Immunology, and Microbiology. Annu Rev Pathol 2016;11:523-54. [Crossref] [PubMed]
- Fuschillo S, De Felice A, Balzano G. Mucosal inflammation in idiopathic bronchiectasis: cellular and molecular mechanisms. Eur Respir J 2008;31:396-406. [Crossref] [PubMed]
- Perea L, Bottier M, Cant E, et al. Airway IL-1β is related to disease severity and mucociliary function in bronchiectasis. Eur Respir J 2024; Epub ahead of print. [Crossref] [PubMed]
- Gaga M, Bentley AM, Humbert M, et al. Increases in CD4+ T lymphocytes, macrophages, neutrophils and interleukin 8 positive cells in the airways of patients with bronchiectasis. Thorax 1998;53:685-91. [Crossref] [PubMed]
- Richman-Eisenstat JB, Jorens PG, Hébert CA, et al. Interleukin-8: an important chemoattractant in sputum of patients with chronic inflammatory airway diseases. Am J Physiol 1993;264:L413-8. [PubMed]
- Martínez-García MA, Perpiñá-Tordera M, Román-Sánchez P, et al. The association between bronchiectasis, systemic inflammation, and tumor necrosis factor alpha. Arch Bronconeumol 2008;44:8-14. [Crossref] [PubMed]
- Tsang KW, Chan K, Ho P, et al. Sputum elastase in steady-state bronchiectasis. Chest 2000;117:420-6. [Crossref] [PubMed]
- Ho JC, Tipoe G, Zheng L, et al. In vitro study of regulation of IL-6 production in bronchiectasis. Respir Med 2004;98:334-41. [Crossref] [PubMed]
- Ho PL, Chan KN, Ip MS, et al. The effect of Pseudomonas aeruginosa infection on clinical parameters in steady-state bronchiectasis. Chest 1998;114:1594-8. [Crossref] [PubMed]
- Dente FL, Bilotta M, Bartoli ML, et al. Neutrophilic Bronchial Inflammation Correlates with Clinical and Functional Findings in Patients with Noncystic Fibrosis Bronchiectasis. Mediators Inflamm 2015;2015:642503. [Crossref] [PubMed]
- Guran T, Ersu R, Karadag B, et al. Association between inflammatory markers in induced sputum and clinical characteristics in children with non-cystic fibrosis bronchiectasis. Pediatr Pulmonol 2007;42:362-9. [Crossref] [PubMed]
- Tsikrika S, Dimakou K, Papaioannou AI, et al. The role of non-invasive modalities for assessing inflammation in patients with non-cystic fibrosis bronchiectasis. Cytokine 2017;99:281-6. [Crossref] [PubMed]
- Tsang KW, Ho PL, Lam WK, et al. Inhaled fluticasone reduces sputum inflammatory indices in severe bronchiectasis. Am J Respir Crit Care Med 1998;158:723-7. [Crossref] [PubMed]
- Proboszcz M, Mycroft K, Paplinska-Goryca M, et al. Relationship between Blood and Induced Sputum Eosinophils, Bronchial Hyperresponsiveness and Reversibility of Airway Obstruction in Mild-to-Moderate Chronic Obstructive Pulmonary Disease. COPD 2019;16:354-61. [Crossref] [PubMed]
- Gao J, Zhang M, Zhou L, et al. Correlation between fractional exhaled nitric oxide and sputum eosinophilia in exacerbations of COPD. Int J Chron Obstruct Pulmon Dis 2017;12:1287-93. [Crossref] [PubMed]
- Hastie AT, Martinez FJ, Curtis JL, et al. Association of sputum and blood eosinophil concentrations with clinical measures of COPD severity: an analysis of the SPIROMICS cohort. Lancet Respir Med 2017;5:956-67. [Crossref] [PubMed]
- Rank MA, Ochkur SI, Lewis JC, et al. Nasal and pharyngeal eosinophil peroxidase levels in adults with poorly controlled asthma correlate with sputum eosinophilia. Allergy 2016;71:567-70. [Crossref] [PubMed]
- Gao J, Wu F. Association between fractional exhaled nitric oxide, sputum induction and peripheral blood eosinophil in uncontrolled asthma. Allergy Asthma Clin Immunol 2018;14:21. [Crossref] [PubMed]
- Shoemark A, Shteinberg M, De Soyza A, et al. Characterization of Eosinophilic Bronchiectasis: A European Multicohort Study. Am J Respir Crit Care Med 2022;205:894-902. [Crossref] [PubMed]
- Gao L, Qin KR, Li T, et al. The clinical phenotype of bronchiectasis and its clinical guiding implications. Exp Biol Med (Maywood) 2021;246:275-80. [Crossref] [PubMed]
- Chang-Macchiu P, Traversi L, Polverino E. Bronchiectasis phenotypes. Curr Opin Pulm Med 2019;25:281-8. [Crossref] [PubMed]
- Terpstra LC, Altenburg J, Doodeman HJ, et al. The effect of azithromycin on sputum inflammatory markers in bronchiectasis. BMC Pulm Med 2023;23:151. [Crossref] [PubMed]
- Jayaram L, King PT, Hunt J, et al. Evaluation of high dose N- Acetylcysteine on airway inflammation and quality of life outcomes in adults with bronchiectasis: A randomised placebo-controlled pilot study. Pulm Pharmacol Ther 2024;84:102283. [Crossref] [PubMed]
- Albrecht FW, Maurer F, Müller-Wirtz LM, et al. Exhaled Volatile Organic Compounds during Inflammation Induced by TNF-α in Ventilated Rats. Metabolites 2020;10:245. [Crossref] [PubMed]
- Kwok WC, Tam TCC, Lam DCL, et al. Blood eosinophil percentage as a predictor of response to inhaled corticosteroid in bronchiectasis. Clin Respir J 2023;17:548-55. [Crossref] [PubMed]
- Martínez-García MÁ, Méndez R, Olveira C, et al. The U-Shaped Relationship Between Eosinophil Count and Bronchiectasis Severity: The Effect of Inhaled Corticosteroids. Chest 2023;164:606-13. [Crossref] [PubMed]
- Saleh AD, Chalmers JD, De Soyza A, et al. The heterogeneity of systemic inflammation in bronchiectasis. Respir Med 2017;127:33-9. [Crossref] [PubMed]