Prognostic value of baseline clinicopathological characteristics in first-line chemotherapy ± immunotherapy for extensive-stage small cell lung cancer: a retrospective cohort study
Highlight box
Key findings
• This study showed the survival benefit of receiving a chemoimmunotherapy regimen as a first-line treatment in a real-world scenario. It also showed the prognostic significance and potential utility of specific baseline clinicopathological characteristics in guiding first-line treatment strategies.
What is known, and what is new?
• Currently, there are inconsistencies in the findings of various studies on the prognostic factors for small cell lung cancer (SCLC), and there remains a shortage of reliable indicators to predict the therapeutic efficacy of treatment regimens.
• This study identified six independent prognostic factors related to the first-line treatment of extensive-stage SCLC (ES-SCLC) and two potential predictive factors associated with the effectiveness of the two first-line treatment modalities.
What is the implication, and what should change now?
• Some baseline clinicopathological characteristics, such as low-density lipoprotein levels, may be of significant value in predicting the prognosis of ES-SCLC patients and the efficacy of first-line treatment regimens. Further prospective studies need to be conducted to validate these findings.
Introduction
Lung cancer is the leading cause of cancer-related death worldwide (1). Small cell lung cancer (SCLC) accounts for about 15% of all lung cancers and is a highly aggressive neuroendocrine malignancy characterized by rapid growth and early metastasis (2). About 80–85% of patients are diagnosed with advanced or extensive-stage small cell lung cancer (ES-SCLC) (3). Over the past four decades, platinum-based chemotherapy has been the cornerstone of the treatment management of ES-SCLC, with etoposide plus platinum being the most commonly used regimen. Most patients with ES-SCLC initially respond to this regimen (4). However, local recurrence or distant metastasis is inevitable, and there is currently a lack of effective subsequent treatment options, which results in a poor prognosis for these patients (5). In recent years, the addition of immune checkpoint inhibitors (ICIs) to the etoposide-platinum chemotherapy regimen has gradually improved the prognosis of patients with this disease, with an approximate improvement in overall survival (OS) of 2–3 months compared to that of chemotherapy alone (6,7). Despite this clinical benefit, it is considered modest, and there is still a need for reliable indicators to predict clinical outcomes or to guide decision making for the initial treatment modality (8).
Many baseline clinicopathological indicators, including demographic characteristics, laboratory variables, and tumor biological properties, are still being explored as markers for predicting treatment response and prognosis in ES-SCLC. Researchers have proposed that clinical indicators such as hyponatremia (9), procalcitonin (PCT) (10), the presence of non-small cell lung cancer (NSCLC) (11) and liver metastasis (12) may be associated with the prognosis of SCLC patients. However, the specific relationships of these indicators with disease progression and prognosis still require further in-depth research and validation.
In this study, we conducted a comprehensive assessment of various clinicopathological baseline characteristics, including clinical information, serum laboratory indicators, and pathological immunohistochemical markers, to determine if they could serve as prognostic indicators for ES-SCLC and to provide new insights for the selection of first-line treatment strategies. We present this article in accordance with the STROBE reporting checklist (available at https://jtd.amegroups.com/article/view/10.21037/jtd-24-929/rc).
Methods
Study population
The study was conducted in accordance with the Declaration of Helsinki (as revised in 2013). The study was approved by Ethics Committee of The Affiliated Lihuili Hospital of Ningbo University (No. KY2022SL458-02) and individual consent for this retrospective analysis was waived. Consecutive patients newly diagnosed with SCLC from April 2017 to April 2023 at The Affiliated Lihuili Hospital of Ningbo University were included in the review. To be eligible for inclusion in this study, the patients had to meet the following inclusion criteria: (I) have sufficient imaging evidence to assess ES-SCLC before treatment according to the Veterans Affairs Lung Study Group (VALSG) staging system; (II) have an Eastern Cooperative Oncology Group (ECOG) performance status of grade 0–1; (III) have received etoposide-platinum as the first-line chemotherapy regimen prior to the authorization of ICI medications, or a combination of etoposide-platinum and ICIs (such as durvalumab, atezolizumab, or srullimab) as the first-line immunochemotherapy; and (IV) have detailed baseline clinicopathological characteristics and imaging assessment after first-line treatment data available. Patients were excluded from the study if they met any of the following exclusion criteria: (I) had a past or current history of another malignant tumor; (II) had developed a disease affecting their short-term survival during treatment and follow up; (III) had complex SCLC with other pathological types; and/or (IV) had discontinued treatment due to the adverse effects of the first-line medications; however, those who had their medication doses reduced were not excluded. Tumor response was evaluated according to the Response Evaluation Criteria in Solid Tumors (RECIST, version 1.1). Progression-free survival (PFS) was defined as the time from diagnosis to the earliest occurrence of disease progression or death from any cause. All patients were followed up through medical record retrieval to obtain survival data, with the last follow-up date being November 1, 2023.
Collection of baseline clinicopathological characteristics
This is a retrospective cohort study. All the data were obtained from the electronic medical record system of The Affiliated Lihuili Hospital of Ningbo University. The basic clinical information of the patients included their demographic characteristics, sites of metastasis, first-line treatment regimen, and PFS. We collected the pre-treatment serological indicators and immunohistochemical staining indicators from diagnostic samples. Data on the following serum laboratory indicators were collected: (I) whole blood cell counts, including neutrophil, lymphocyte, monocyte, and platelet (PLT) counts; (II) lipid indexes, including triglycerides (TGs), total cholesterol (TC), high-density lipoprotein (HDL), low-density lipoprotein (LDL), apolipoprotein A1 (ApoA1), and apolipoprotein B (ApoB); (III) coagulation parameters, with fibrinogen (FIB) as the present study biomarker; (IV) biochemical indices, including serum lactate dehydrogenase (LDH) and sodium; (V) tumor markers, including carcinoembryonic antigen (CEA), cancer antigen 19-9 (CA19-9), squamous epithelial associated antigen (SEAA), cytokeratin, and neuron-specific enolase (NSE). The collected data set also included the principal immunohistochemical markers for diagnosis, such as synuclein (SYN) (Antibody Clone SYN C9D11, Celnovte, Henan, China), chromogranin A (CgA) (Antibody Clone CgA LK2H10, ZSGB-Bio, Beijing, China), cluster of differentiation 56 (CD56) (Antibody Clone CD56 UMAB83, ZSGB-Bio), thyroid transcription factor 1 (TTF-1) (Antibody Clone TTF1 SPT24, ZSGB-Bio), and Ki-67 (Antibody Clone Ki67 UMAB107, ZSGB-Bio).
Statistical analysis
The primary endpoint of this study was PFS. Differences between the two treatment groups in the categorical variables and continuous variables were compared using Fisher’s exact test and the Wilcoxon test, respectively. The correlation between two continuous variables [e.g., body mass index (BMI) and LDL] was calculated using the Pearson correlation coefficient. Optimal cut-off values for all the continuous variables, such as Ki-67, FIB, and LDL, were determined using X-tile, except for the blood sodium level, which was classified as hyponatremia or normal. Univariate analyses were conducted to assess the association between PFS and potential prognostic factors using the Cox proportional hazards regression model. Multivariate analyses were then conducted to identify the independent predictors of outcomes (using a stepwise method, with P<0.1 as the cut-off value). Potential predictive markers for treatment efficacy were identified using a Cox regression model that included an interaction term between the treatment modality and the marker. The P value of the interaction term was used to assess whether the marker affected the treatment outcomes. Survival curves were generated using the Kaplan-Meier method. A P value of <0.05 was considered statistically significant. Samples with missing entries were excluded, and the analysis was conducted on the remaining data. All the statistical tests were two-sided. The data analyses were performed with SAS software, version 9.4 and R software (version 4.0.3, https://www.r-project.org/).
Results
Baseline clinicopathological characteristics
In total, 121 ES-SCLC patients who met the selection criteria were enrolled in the study and separated into the chemotherapy (n=62) and ICI plus chemotherapy (n=59) cohorts. The detailed baseline clinicopathological characteristics of the cohorts are summarized in Table 1 and table available at: https://cdn.amegroups.cn/static/public/10.21037jtd-24-929-1.xlsx. The mean or median count of each continuous variable was calculated for the cohort comparison. As Table 1 shows, the chemotherapy cohort had significantly higher levels of FIB (5.09 vs. 4.47 g/L) and SEAA (1.61 vs. 1.12 ng/mL), while the ICI + chemotherapy cohort had significantly higher lymphocyte counts (1.51×109/L vs. 1.38×109/L), serum sodium levels (138.86 vs. 136.56 mmol/L), and NSE levels (55.90 vs. 39.91 ng/mL). The other clinicopathological factors did not differ significantly between the two treatment groups.
Table 1
Variables | All patients (n=121) | Chemotherapy cohort (n=62) | ICI plus chemotherapy cohort (n=59) | P value |
---|---|---|---|---|
Age (years), n (%) | 0.56 (Fisher test) | |||
<65 | 55 (45.45) | 30 (48.39) | 25 (42.37) | |
≥65 | 66 (54.55) | 32 (51.61) | 34 (57.63) | |
Gender, n (%) | 0.36 (Fisher test) | |||
Female | 4 (3.31) | 1 (1.61) | 3 (5.08) | |
Male | 117 (96.69) | 61 (98.39) | 56 (94.92) | |
Smoking, n (%) | >0.99 (Fisher test) | |||
No | 24 (19.83) | 12 (19.35) | 12 (20.34) | |
Yes | 97 (80.17) | 50 (80.65) | 47 (79.66) | |
SYN, n (%) | 0.32 (Fisher test) | |||
Negative | 9 (7.44) | 3 (4.84) | 6 (10.17) | |
Positive | 111 (91.74) | 58 (93.55) | 53 (89.83) | |
Missing | 1 (0.83) | 1 (1.61) | 0 (0.00) | |
CgA, n (%) | >0.99 (Fisher test) | |||
Negative | 55 (45.45) | 28 (45.16) | 27 (45.76) | |
Positive | 65 (53.72) | 33 (53.23) | 32 (54.24) | |
Missing | 1 (0.83) | 1 (1.61) | 0 (0.00) | |
CD56, n (%) | 0.36 (Fisher test) | |||
Negative | 12 (9.92) | 8 (12.90) | 4 (6.78) | |
Positive | 105 (86.78) | 52 (83.87) | 53 (89.83) | |
Missing | 4 (3.31) | 2 (3.23) | 2 (3.39) | |
TTF-1, n (%) | 0.35 (Fisher test) | |||
Negative | 22 (18.18) | 9 (14.52) | 13 (22.03) | |
Positive | 98 (80.99) | 52 (83.87) | 46 (77.97) | |
Missing | 1 (0.83) | 1 (1.61) | 0 (0.00) | |
Ki-67 (%) | 0.77 (Wilcox test) | |||
Mean (SD) | 74.44 (14.33) | 74.48 (15.69) | 74.41 (13.03) | |
Median [IQR] | 80.00 [70.00, 80.00] | 80.00 [70.00, 90.00] | 80.00 [70.00, 80.00] | |
Missing, n (%) | 6 (4.96) | 6 (9.68) | 0 (0.00) | |
FIB (g/L) | 0.04 (Wilcox test) | |||
Mean (SD) | 4.79 (1.62) | 5.09 (1.70) | 4.47 (1.49) | |
Median [IQR] | 4.35 [3.66, 5.86] | 4.73 [3.89, 6.01] | 4.08 [3.57, 5.29] | |
Missing, n (%) | 1 (0.83) | 1 (1.61) | 0 (0.00) | |
Neutrophil (×109/L) | 0.67 (Wilcox test) | |||
Mean (SD) | 4.53 (2.01) | 4.54 (2.11) | 4.52 (1.91) | |
Median [IQR] | 4.10 [3.30, 4.80] | 4.10 [3.30, 4.80] | 4.20 [3.35, 4.90] | |
Missing, n (%) | 1 (0.83) | 1 (1.61) | 0 (0.00) | |
Lymphocyte (×109/L) | 0.02 (Wilcox test) | |||
Mean (SD) | 1.44 (0.52) | 1.38 (0.59) | 1.51 (0.45) | |
Median [IQR] | 1.30 [1.10, 1.70] | 1.20 [1.00, 1.60] | 1.40 [1.20, 1.80] | |
Missing, n (%) | 1 (0.83) | 1 (1.61) | 0 (0.00) | |
Monocyte (×109/L) | 0.99 (Wilcox test) | |||
Mean (SD) | 0.73 (1.18) | 0.87 (1.63) | 0.58 (0.24) | |
Median [IQR] | 0.50 [0.40, 0.70] | 0.50 [0.40, 0.70] | 0.50 [0.40, 0.70] | |
Missing, n (%) | 1 (0.83) | 1 (1.61) | 0 (0.00) | |
PLT (×109/L) | 0.06 (Wilcox test) | |||
Mean (SD) | 227.07 (75.48) | 217.62 (76.24) | 236.83 (74.07) | |
Median [IQR] | 219.50 [178.75, 265.00] | 204.00 [169.00, 256.00] | 236.00 [193.00, 268.50] | |
Missing, n (%) | 1 (0.83) | 1 (1.61) | 0 (0.00) | |
Blood_sodium (mmol/L) | 0.04 (Wilcox test) | |||
Mean (SD) | 137.69 (4.42) | 136.56 (5.21) | 138.86 (3.03) | |
Median [IQR] | 138.15 [136.60, 140.03] | 137.80 [136.10, 139.20] | 138.50 [136.80, 141.55] | |
Missing, n (%) | 1 (0.83) | 1 (1.61) | 0 (0.00) | |
TG (mmol/L) | 0.85 (Wilcox test) | |||
Mean (SD) | 1.24 (0.52) | 1.24 (0.53) | 1.23 (0.51) | |
Median [IQR] | 1.08 [0.84, 1.59] | 1.04 [0.84, 1.67] | 1.19 [0.86, 1.51] | |
Missing, n (%) | 1 (0.83) | 1 (1.61) | 0 (0.00) | |
TC (mmol/L) | 0.56 (Wilcox test) | |||
Mean (SD) | 4.19 (0.88) | 4.24 (0.98) | 4.15 (0.79) | |
Median [IQR] | 4.14 [3.70, 4.70] | 4.22 [3.71, 4.75] | 4.02 [3.69, 4.59] | |
Missing, n (%) | 1 (0.83) | 1 (1.61) | 0 (0.00) | |
HDL (mmol/L) | 0.74 (Wilcox test) | |||
Mean (SD) | 1.1 (0.26) | 1.11 (0.27) | 1.09 (0.24) | |
Median [IQR] | 1.08 [0.91, 1.26] | 1.09 [0.91, 1.23] | 1.06 [0.91, 1.27] | |
Missing, n (%) | 1 (0.83) | 1 (1.61) | 0 (0.00) | |
LDL (mmol/L) | 0.48 (Wilcox test) | |||
Mean (SD) | 2.47 (0.63) | 2.43 (0.64) | 2.52 (0.62) | |
Median [IQR] | 2.44 [2.06, 2.88] | 2.43 [1.99, 2.76] | 2.49 [2.17, 2.90] | |
Missing, n (%) | 1 (0.83) | 1 (1.61) | 0 (0.00) | |
ApoA1 (g/L) | 0.45 (Wilcox test) | |||
Mean (SD) | 1.15 (0.24) | 1.14 (0.28) | 1.17 (0.20) | |
Median [IQR] | 1.15 [1.00, 1.27] | 1.13 [0.99, 1.27] | 1.16 [1.04, 1.26] | |
Missing, n (%) | 19 (15.70) | 9 (14.52) | 10 (16.95) | |
ApoB (g/L) | 0.92 (Wilcox test) | |||
Mean (SD) | 0.8 (0.19) | 0.81 (0.20) | 0.8 (0.18) | |
Median [IQR] | 0.80 [0.68, 0.91] | 0.81 [0.67, 0.90] | 0.79 [0.68, 0.91] | |
Missing, n (%) | 19 (15.70) | 9 (14.52) | 10 (16.95) | |
LDH (U/L) | 0.58 (Wilcox test) | |||
Mean (SD) | 380.52 (322.89) | 331.48 (227.12) | 430.49 (393.59) | |
Median [IQR] | 250.00 [203.00, 387.50] | 250.50 [202.50, 337.25] | 250.00 [204.00, 479.00] | |
Missing, n (%) | 14 (11.57) | 8 (12.90) | 6 (10.17) | |
CEA (μg/L) | 0.49 (Wilcox test) | |||
Mean (SD) | 31.58 (93.77) | 40.26 (122.13) | 22.31 (47.17) | |
Median [IQR] | 3.60 [2.18, 20.22] | 4.60 [2.10, 25.40] | 3.40 [2.32, 12.42] | |
Missing, n (%) | 1 (0.83) | 0 (0.00) | 1 (1.69) | |
CA19-9 (U/mL) | 0.97 (Wilcox test) | |||
Mean (SD) | 123.96 (464.39) | 77.5 (200.28) | 170.42 (625.20) | |
Median [IQR] | 14.25 [9.15, 31.33] | 13.30 [8.98, 37.65] | 15.95 [9.50, 21.65] | |
Missing, n (%) | 13 (10.74) | 8 (12.90) | 5 (8.47) | |
SEAA (μg/L) | 0.03 (Wilcox test) | |||
Mean (SD) | 1.37 (1.47) | 1.61 (1.94) | 1.12 (0.66) | |
Median [IQR] | 1.08 [0.76, 1.48] | 1.11 [0.94, 1.65] | 0.97 [0.63, 1.37] | |
Missing, n (%) | 6 (4.96) | 3 (4.84) | 3 (5.08) | |
Cytokeratin (μg/L) | 0.54 (Wilcox test) | |||
Mean (SD) | 4.96 (4.86) | 4.35 (3.37) | 5.61 (6.02) | |
Median [IQR] | 3.62 [2.75, 5.42] | 3.67 [2.66, 5.05] | 3.56 [2.88, 5.61] | |
Missing, n (%) | 6 (4.96) | 3 (4.84) | 3 (5.08) | |
NSE (ng/mL) | 0.01 (Wilcox test) | |||
Mean (SD) | 47.55 (59.96) | 39.91 (59.84) | 55.90 (59.52) | |
Median [IQR] | 22.45 [12.48, 53.63] | 18.49 [10.43, 41.47] | 28.04 [15.99, 59.53] | |
Missing, n (%) | 8 (6.61) | 3 (4.84) | 5 (8.47) | |
BMI (kg/m2) | 0.14 (Wilcox test) | |||
Mean (SD) | 23.08 (2.93) | 23.54 (3.19) | 22.65 (2.62) | |
Median [IQR] | 22.94 [21.12, 25.38] | 23.08 [21.54, 25.73] | 22.63 [20.91, 24.32] | |
Missing, n (%) | 9 (7.44) | 8 (12.90) | 1 (1.69) | |
Brain_M, n (%) | 0.32 (Fisher test) | |||
Yes | 19 (15.70) | 12 (19.35) | 7 (11.86) | |
No | 102 (84.30) | 50 (80.65) | 52 (88.14) | |
Bone_M, n (%) | 0.33 (Fisher test) | |||
Yes | 36 (29.75) | 21 (33.87) | 15 (25.42) | |
No | 85 (70.25) | 41 (66.13) | 44 (74.58) | |
Liver_M, n (%) | 0.45 (Fisher test) | |||
Yes | 39 (32.23) | 22 (35.48) | 17 (28.81) | |
No | 82 (67.77) | 40 (64.52) | 42 (71.19) | |
Other_M, n (%) | 0.31 (Fisher test) | |||
Yes | 18 (14.88) | 7 (11.29) | 11 (18.64) | |
No | 103 (85.12) | 55 (88.71) | 48 (81.36) |
ES-SCLC, extensive-stage small cell lung cancer; ICI, immune checkpoint inhibitor; SYN, synuclein; CgA, chromogranin A; CD56, cluster of differentiation 56; TTF-1, thyroid transcription factor 1; SD, standard deviation; IQR, interquartile range; FIB, fibrinogen; PLT, platelet; TG, triglyceride; TC, total cholesterol; HDL, high-density lipoprotein; LDL, low-density lipoprotein; ApoA1, apolipoprotein A1; ApoB, apolipoprotein B; LDH, lactate dehydrogenase; CEA, carcinoembryonic antigen; CA19-9, cancer antigen 19-9; SEAA, squamous epithelial associated antigen; NSE, neuron-specific enolase; BMI, body mass index; M, metastasis.
The average follow-up time for all patients is 5.9 months. As of the cutoff for the last follow-up, all patients in the chemotherapy cohorts had experienced PFS outcome events, and within the ICI plus chemotherapy cohorts, 52 out of 59 patients had achieved PFS outcome events. The median PFS in the chemotherapy cohorts was 4.3 months [95% confidence interval (CI): 3.3, 4.9], whereas the median PFS in the ICI plus chemotherapy cohorts was 6.1 months (95% CI: 4.6, 7.6).
Univariate and multivariate analysis of PFS in ES-SCLC
Using X-tile, we categorized the normally distributed continuous variables, including Ki-67, FIB, and LDL, into categorical variables based on optimal cut-off values, except for the serum sodium levels, which were categorized as hyponatremia and normal. Table 2 presents the factors with a P value <0.1 from the univariate Cox regression analysis of PFS in the ES-SCLC patients receiving first-line treatment. These parameters were then included in the multivariate Cox regression analysis. Meanwhile, Table S1 provides the results of the univariate analysis with a P value ≥0.1. In the univariate analysis, a total of 15 factors associated with PFS in all the ES-SCLC patients were identified, including the treatment modality, LDH level, NSE level, PLT count, lymphocyte count, age, serum sodium level, ApoA1 concentration, FIB level, NLR, ApoB concentration, LDL level, CgA, bone metastasis, and the sum of tissues with metastatic lesions (P<0.1 for all). Among these factors, the treatment modality emerged as the most significant prognostic factor, showing that immunochemotherapy significantly prolonged PFS compared to chemotherapy alone [P<0.001; hazard ratio (HR) =0.42; 95% CI: 0.28, 0.64; Figure 1]. A multivariate analysis was conducted to determine the correlation among different prognostic variables. We observed that a immunochemotherapy regimen (P<0.001; HR =0.40; 95% CI: 0.24, 0.68) and LDL level of >1.8 mmol/L (P=0.02; HR =0.41; 95% CI: 0.20, 0.85) were independent prognostic factors of favorable PFS, while a LDH level of >273 U/L (P=0.04; HR =1.78; 95% CI: 1.03, 3.07), NSE concentration of >102.6 ng/mL (P=0.009; HR =6.49; 95% CI: 1.60, 26.32), ApoA1 concentration of >0.9 g/L (P<0.001; HR =4.15; 95% CI: 1.98, 8.71), and ApoB concentration of >0.8 g/L (P=0.002; HR =2.24; 95% CI: 1.34, 3.75) were independent prognostic factors of poorer PFS in all ES-SCLC patients (P<0.05 for all). It was worth noting that in the chemoimmunotherapy cohort, an LDL level of >1.8 mmol/L significantly improved patient prognosis in terms of PFS (P=0.003; HR =4.59; 95% CI: 1.52, 13.90) (Figure S1), while in the chemotherapy cohort, an LDL level of ≤1.8 mmol/L showed a trend toward improved patient prognosis (Figure S2A). Notably, the recurrence rate within the first 3 months after chemotherapy was significantly lower in this group compared to those with LDL levels of >1.8 mmol/L (Figure S2B).
Table 2
Variables | Reference | Univariate analysis | Multivariate analysis | |||
---|---|---|---|---|---|---|
HR (95% CI) | P value | HR (95% CI) | P value | |||
Treatment | ICI + chemo vs. chemo | 0.42 (0.28, 0.64) | <0.001 | 0.40 (0.24, 0.68) | <0.001 | |
LDH273 | >273 vs. ≤273 U/L | 2 (1.32, 3.02) | 0.001 | 1.78 (1.03, 3.07) | 0.04 | |
NSE_C | >102.6 vs. ≤102.6 ng/mL | 2.26 (1.33, 3.84) | 0.003 | 6.49 (1.60, 26.32) | 0.009 | |
LDH | – | 1 (1, 1) | 0.003 | – | – | |
LDH_C | >245 vs. ≤245 U/L | 1.64 (1.1, 2.45) | 0.02 | – | – | |
PLT_C | >234×109/L vs. ≤234×109/L | 0.63 (0.43, 0.94) | 0.02 | – | – | |
Lymphocyte_C | >1.4×109/L vs. ≤1.4×109/L | 0.65 (0.44, 0.95) | 0.03 | – | – | |
sum_M | – | 1.32 (1.03, 1.68) | 0.03 | – | – | |
Bone_M | Yes vs. no | 1.57 (1.04, 2.37) | 0.03 | – | – | |
Age | ≥65 vs. <65 years | 0.67 (0.46, 0.97) | 0.04 | – | – | |
Blood_sodium_C | >135 vs. ≤135 mmol/L | 0.53 (0.3, 0.96) | 0.04 | – | – | |
ApoA1_C | >0.9 vs. ≤0.9 g/L | 1.85 (1.01, 3.4) | 0.048 | 4.15 (1.98, 8.71) | <0.001 | |
FIB_C | >2.99 vs. ≤2.99 g/L | 1.82 (0.99, 3.32) | 0.053 | – | – | |
NSE | – | 1 (1, 1.01) | 0.06 | – | – | |
NLR_C | >3.1 vs. ≤3.1 | 1.43 (0.98, 2.1) | 0.06 | – | – | |
ApoB _C | >0.8 vs. ≤0.8 g/L | 1.47 (0.98, 2.2) | 0.06 | 2.24 (1.34, 3.75) | 0.002 | |
LDL_C | >1.8 vs. ≤1.8 mmol/L | 0.6 (0.34, 1.05) | 0.07 | 0.41 (0.20, 0.85) | 0.02 | |
CgA | Positive vs. negative | 0.71 (0.49, 1.04) | 0.08 | – | – |
The factors associated with PFS in ES-SCLC patients with a P value <0.1 in the univariate analysis were included in the multivariate analysis. A P value <0.05 was considered statistically significant. HR, hazard ratio; CI, confidence interval; ICI, immune checkpoint inhibitor; chemo, chemotherapy; LDH, lactate dehydrogenase; NSE, neuron-specific enolase; C, denotes the clinical features converted to categorical variables; PLT, platelet; sum_M, sum of tissues with metastatic lesions (e.g., brain, bone, liver, or other tissues); Bone_M, bone metastasis; ApoA1, apolipoprotein A1; FIB, fibrinogen; NLR, neutrophil-to-lymphocyte ratio; ApoB, apolipoprotein B; LDL, low-density lipoprotein; CgA, chromogranin A; PFS, progression-free survival; ES-SCLC, extensive-stage small cell lung cancer.
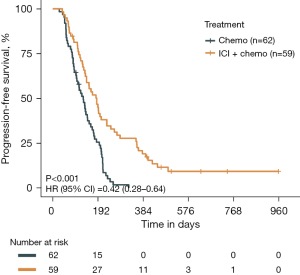
Furthermore, we found that LDL levels independently and significantly influenced PFS of patients receiving chemotherapy combined with ICI, but there was no significant association with BMI (Figure S3).
Potential predictive biomarkers of treatment efficacy in ES-SCLC
To identify the clinicopathological factors that might affect the efficacy of different treatments in patients, we constructed a Cox regression model incorporating interaction terms between treatment modalities and potential biomarkers (Table S2). The interaction effect analysis revealed significant interactions between the LDL levels and the presence of bone metastasis with treatment outcomes (LDL_C interaction P=0.007; bone metastasis interaction P=0.03). In patients with an LDL level of >1.8 mmol/L or those without bone metastasis, the efficacy of combined immunotherapy and chemotherapy was significantly superior to chemotherapy alone (both P<0.001). Conversely, in patients with an LDL level of ≤1.8 mmol/L or those with bone metastasis, there was no significant difference in the treatment efficacy (LDL_C ≤1.8 mmol/L P=0.12; with bone metastasis P=0.54) (Figure 2). These findings suggest that immunotherapy combined with chemotherapy is the preferred choice for patients with an LDL level of >1.8 mmol/L or those without bone metastasis, while for patients with an LDL level of ≤1.8 mmol/L or those with bone metastasis, either combination therapy or chemotherapy alone are viable options.
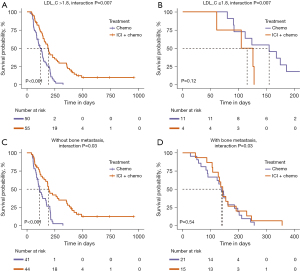
Discussion
In this study, we investigated two cohorts of ES-SCLC patients who received immunochemotherapy or chemotherapy alone as the first-line treatment to assess the correlation between various baseline clinicopathological characteristics and disease prognosis. We also attempted to identify the potential predictive factors of the efficacy of the two first-line treatment modalities.
Multiple factors, including sex, age, smoking history, tumor stage, performance status, weight loss, tumor burden, and increases in tumor biomarkers, have been shown to effect the survival outcomes of SCLC patients (13,14). However, discrepancies exist in the outcomes of various studies on prognostic factors for SCLC, and there remains a shortage of reliable indicators to predict the therapeutic efficacy of treatment regimens. In terms of the clinical information, hematological indicators, and pathological immunohistochemical indicators collected for this study cohort, we identified six independent prognostic factors related to the first-line treatment of ES-SCLC and two potential predictive factors associated with the effectiveness of the two first-line treatment modalities. Landmark studies, such as IMpower133 and CASPIAN, have established immunochemotherapy as the frontline treatment for ES-SCLC (6,7). Our real-world evidence has solidified the fact that immunochemotherapy markedly improves PFS in comparison to chemotherapy alone. This combined therapeutic strategy has proven to be a pivotal prognostic element in patient care.
Since it has been noted that serum LDH levels frequently increase in cancer patients and are associated with suboptimal clinical outcomes and treatment resistance, the measurement of LDH has become an essential diagnostic and monitoring tool in the field of oncology (15). LDH has been widely investigated as a prognostic indicator in SCLC. Sagman et al.’ s research of 288 SCLC patients showed that serum LDH is a crucial, independent prognostic factor for SCLC, influencing disease stage, treatment response, and survival (16). The research conducted by He et al. demonstrated that pretreatment serum LDH levels of ≥215.70 U/L were significantly associated with prognosis in patients with SCLC receiving first-line platinum-based chemotherapy, regardless of the stage (17). Huang et al. identified LDH as a prognostic factor for PFS in SCLC patients receiving platinum-based chemotherapy, regardless of their stage, with a threshold of 263 U/L for OS prediction (18). In present study, we found that LDH, with an optimal cut-off value of 273 U/L, was an independent prognostic factor for the first-line treatment of SCLC patients; however, no statistical significance was found when LDH was included as a continuous variable, or at the clinical reference value of 245 U/L in the multivariate analysis.
NSE exhibits high specificity in neurons and peripheral neuroendocrine cells (19). It serves as a reliable tumor marker for patients with SCLC. In a prognostic analysis of 523 cases of SCLC by Zhou et al., it was discovered that NSE was an independent prognostic factor affecting OS, regardless of the stage of the disease (20). Additionally, studies have shown that pretreatment NSE levels of 40.80 or 15 ng/mL do not act as independent prognostic factors for OS in SCLC (17,21). In this study, we identified that a cut-off concentration of 102.6 ng/mL was an independent prognostic factor for patients with ES-SCLC receiving first-line treatment with chemotherapy ± immunotherapy.
Lipids are a vital nutritional source for tumor cells, and fluctuations in their levels may affect tumor growth and patient survival. Research into lipids as factors related to tumor prognosis has been increasing in recent years. Serum cholesterol, HDL, LDL, and ApoA-1 have been found to be associated with the prognosis of various cancers, such as breast, ovarian, and hepatocellular carcinomas (22-24). Wu et al. analyzed the link between lipid levels and SCLC prognosis, and identified TC, HDL, and the TG/HDL ratio as key independent factors for PFS in ES-SCLC patients (25). The TG level has also been found to be an independent risk factor for SCLC progression, with elevated TG levels suggesting rapid disease progression and a poor prognosis (26). In our study, we failed to find that TC, TG, and HDL were independent prognostic factors for the first-line treatment of ES-SCLC. A prospective study showed that ApoA1 was negatively associated with the risk of lung cancer, while high ApoB was associated with an increased risk of lung cancer (27). Subsequently, a pharmacogenetic Mendelian randomization analysis has demonstrated that inhibition of ApoB confers a reduction in the incidence of SCLC (28). In the context of our research, we have established that ApoA1 and ApoB, with optimal cut-off concentrations of 0.9 and 0.8 g/L, respectively, are independent prognostic factors for ES-SCLC. Zhou et al. confirmed that lower LDL and low-density lipoprotein receptor (LDLR) expression are independent prognostic factors associated with longer OS in SCLC patients treated with platinum-based chemotherapy (29). Our study demonstrated that high levels of LDL were an independent prognostic factor for improved PFS in the entire treatment population, which appears to contradict previous findings on first-line chemotherapy regimens that showed that patients with low LDL levels had a better prognosis. A further analysis of the chemotherapy-alone cohort revealed a trend that suggested that lower LDL levels may improve patient prognosis, but the difference was not statistically significant.
As a first-line treatment for ES-SCLC, immunochemotherapy provides a sustained benefit to only a small fraction of patients, and traditional predictive biomarkers are not applicable to SCLC. A recent transcriptomic analysis identified an inflammatory SCLC subtype (SCLC-I) that exhibited an enhanced benefit with the addition of atezolizumab during chemotherapy (30). Hardy-Werbin et al. (31) found that elevated levels of interleukin-2 (IL-2) serve as a predictive biomarker for sensitivity to ipilimumab in the context of SCLC immunochemotherapy. In our study, a pretreatment LDL level of >1.8 mmol/L was not only an independent prognostic factor for the overall population with ES-SCLC, it was also a potential predictive marker that patients would receive a greater benefit from the combined modality of chemotherapy and immunotherapy. Additionally, patients without bone metastasis were more likely to benefit from immunochemotherapy, while no significant difference in the benefit to PFS between immunochemotherapy and chemotherapy alone was found for those with bone metastasis.
Our study had certain limitations. In our study, OS data was missing by more than 20%. Considering the potential impact on the reliability of the results, we opted to use PFS for our research. Additionally, it was a single-center, retrospective study, which inherently introduces potential biases. Second, our sample size was comparatively modest, particularly that of the subgroup of patients with an LDL level of ≤1.8 mmol/L in the immunochemotherapy cohort. We were unable to identify demographic features or pathological immunohistochemical markers as prognostic or potential predictive factors, a shortfall that may be due to the smaller sample size, which might have potentially affected the validity of our results. Consequently, larger, well-designed, prospective studies need to be conducted to validate these findings.
Conclusions
In conclusion, an immunochemotherapy regimen and LDL level of >1.8 mmol/L were independent prognostic factors of favorable PFS, while a LDH level of >273 U/L, NSE concentration of >102.6 ng/mL, ApoA1 concentration of >0.9 g/L, and ApoB concentration of >0.8 g/L were independent prognostic factors of poorer PFS in all the ES-SCLC patients. Additionally, for patients with LDL levels of >1.8 mmol/L or those without bone metastasis, ICIs combined with chemotherapy is the preferred first-line treatment. For patients with LDL levels of ≤1.8 mmol/L or those with bone metastasis, both immunochemotherapy and single chemotherapy are viable options for first-line therapy.
Acknowledgments
Funding: The present study was financially supported by
Footnote
Reporting Checklist: The authors have completed the STROBE reporting checklist. Available at https://jtd.amegroups.com/article/view/10.21037/jtd-24-929/rc
Data Sharing Statement: Available at https://jtd.amegroups.com/article/view/10.21037/jtd-24-929/dss
Peer Review File: Available at https://jtd.amegroups.com/article/view/10.21037/jtd-24-929/prf
Conflicts of Interest: All authors have completed the ICMJE uniform disclosure form (available at https://jtd.amegroups.com/article/view/10.21037/jtd-24-929/coif). A.R. serves as an unpaid editorial board member of Journal of Thoracic Disease from August 2023 to July 2025. A.R. reports stock options by IQVIA Holdings Inc. The other authors have no conflicts of interest to declare.
Ethical Statement: The authors are accountable for all aspects of the work in ensuring that questions related to the accuracy or integrity of any part of the work are appropriately investigated and resolved. The study was conducted in accordance with the Declaration of Helsinki (as revised in 2013). The study was approved by Ethics Committee of The Affiliated Lihuili Hospital of Ningbo University (No. KY2022SL458-02) and individual consent for this retrospective analysis was waived.
Open Access Statement: This is an Open Access article distributed in accordance with the Creative Commons Attribution-NonCommercial-NoDerivs 4.0 International License (CC BY-NC-ND 4.0), which permits the non-commercial replication and distribution of the article with the strict proviso that no changes or edits are made and the original work is properly cited (including links to both the formal publication through the relevant DOI and the license). See: https://creativecommons.org/licenses/by-nc-nd/4.0/.
References
- Bade BC, Dela Cruz CS. Lung Cancer 2020: Epidemiology, Etiology, and Prevention. Clin Chest Med 2020;41:1-24. [Crossref] [PubMed]
- Gazdar AF, Bunn PA, Minna JD. Small-cell lung cancer: what we know, what we need to know and the path forward. Nat Rev Cancer 2017;17:725-37. [Crossref] [PubMed]
- Schwendenwein A, Megyesfalvi Z, Barany N, et al. Molecular profiles of small cell lung cancer subtypes: therapeutic implications. Mol Ther Oncolytics 2021;20:470-83. [Crossref] [PubMed]
- Oronsky B, Abrouk N, Caroen S, et al. A 2022 Update on Extensive Stage Small-Cell Lung Cancer (SCLC). J Cancer 2022;13:2945-53. [Crossref] [PubMed]
- Demedts IK, Vermaelen KY, van Meerbeeck JP. Treatment of extensive-stage small cell lung carcinoma: current status and future prospects. Eur Respir J 2010;35:202-15. [Crossref] [PubMed]
- Liu SV, Reck M, Mansfield AS, et al. Updated Overall Survival and PD-L1 Subgroup Analysis of Patients With Extensive-Stage Small-Cell Lung Cancer Treated With Atezolizumab, Carboplatin, and Etoposide (IMpower133). J Clin Oncol 2021;39:619-30. [Crossref] [PubMed]
- Goldman JW, Dvorkin M, Chen Y, et al. Durvalumab, with or without tremelimumab, plus platinum-etoposide versus platinum-etoposide alone in first-line treatment of extensive-stage small-cell lung cancer (CASPIAN): updated results from a randomised, controlled, open-label, phase 3 trial. Lancet Oncol 2021;22:51-65. [Crossref] [PubMed]
- Petty WJ, Paz-Ares L. Emerging Strategies for the Treatment of Small Cell Lung Cancer: A Review. JAMA Oncol 2023;9:419-29. [Crossref] [PubMed]
- Hermes A, Waschki B, Reck M. Hyponatremia as prognostic factor in small cell lung cancer--a retrospective single institution analysis. Respir Med 2012;106:900-4. [Crossref] [PubMed]
- Ichikawa K, Watanabe S, Miura S, et al. Prognostic significance of procalcitonin in small cell lung cancer. Transl Lung Cancer Res 2022;11:43-52. [Crossref] [PubMed]
- Yang L, Zhou Y, Wang G, et al. Clinical features and prognostic factors of combined small cell lung cancer: development and validation of a nomogram based on the SEER database. Transl Lung Cancer Res 2021;10:4250-65. [Crossref] [PubMed]
- Zhang S, Wang Y, Li S, et al. A retrospective analysis of prognostic factors and treatment choices in small cell lung cancer with liver metastasis. J Thorac Dis 2023;15:6776-87. [Crossref] [PubMed]
- Kalemkerian GP, Schneider BJ. Advances in Small Cell Lung Cancer. Hematol Oncol Clin North Am 2017;31:143-56. [Crossref] [PubMed]
- Yip D, Harper PG. Predictive and prognostic factors in small cell lung cancer: current status. Lung Cancer 2000;28:173-85. [Crossref] [PubMed]
- Forkasiewicz A, Dorociak M, Stach K, et al. The usefulness of lactate dehydrogenase measurements in current oncological practice. Cell Mol Biol Lett 2020;25:35. [Crossref] [PubMed]
- Sagman U, Feld R, Evans WK, et al. The prognostic significance of pretreatment serum lactate dehydrogenase in patients with small-cell lung cancer. J Clin Oncol 1991;9:954-61. [Crossref] [PubMed]
- He M, Chi X, Shi X, et al. Value of pretreatment serum lactate dehydrogenase as a prognostic and predictive factor for small-cell lung cancer patients treated with first-line platinum-containing chemotherapy. Thorac Cancer 2021;12:3101-9. [Crossref] [PubMed]
- Huang W, Liu P, Zong M, et al. Combining Lactate Dehydrogenase and Fibrinogen: Potential Factors to Predict Therapeutic Efficacy and Prognosis of Patients with Small-Cell Lung Cancer. Cancer Manag Res 2021;13:4299-307. [Crossref] [PubMed]
- Isgrò MA, Bottoni P, Scatena R. Neuron-Specific Enolase as a Biomarker: Biochemical and Clinical Aspects. Adv Exp Med Biol 2015;867:125-43. [Crossref] [PubMed]
- Zhou M, Wang Z, Yao Y, et al. Neuron-specific enolase and response to initial therapy are important prognostic factors in patients with small cell lung cancer. Clin Transl Oncol 2017;19:865-73. [Crossref] [PubMed]
- Li J, Dai CH, Chen P, et al. Survival and prognostic factors in small cell lung cancer. Med Oncol 2010;27:73-81. [Crossref] [PubMed]
- Liang Z, Zhang Z, Tan X, et al. Lipids, cholesterols, statins and liver cancer: a Mendelian randomization study. Front Oncol 2023;13:1251873. [Crossref] [PubMed]
- Zhou P, Li B, Liu B, et al. Prognostic role of serum total cholesterol and high-density lipoprotein cholesterol in cancer survivors: A systematic review and meta-analysis. Clin Chim Acta 2018;477:94-104. [Crossref] [PubMed]
- Li X, Liu ZL, Wu YT, et al. Status of lipid and lipoprotein in female breast cancer patients at initial diagnosis and during chemotherapy. Lipids Health Dis 2018;17:91. [Crossref] [PubMed]
- Wu M, He Y, Pan C. Analysis of Baseline Serum Lipid Profile for Predicting Clinical Outcomes of Patients with Extensive-Stage Small Cell Lung Cancer. Cancer Manag Res 2023;15:773-83. [Crossref] [PubMed]
- Cui YN, Gao Q, Zhu SS, et al. Effect of serum lipid level on prognosis of patients with small cell lung cancer at the initial treatment. Zhonghua Zhong Liu Za Zhi 2021;43:318-23. [Crossref] [PubMed]
- Borgquist S, Butt T, Almgren P, et al. Apolipoproteins, lipids and risk of cancer. Int J Cancer 2016;138:2648-56. [Crossref] [PubMed]
- Li H, Zhang L, Yang F, et al. Lipid-lowering drugs affect lung cancer risk via sphingolipid metabolism: a drug-target Mendelian randomization study. Front Genet 2023;14:1269291. [Crossref] [PubMed]
- Zhou T, Zhan J, Fang W, et al. Serum low-density lipoprotein and low-density lipoprotein expression level at diagnosis are favorable prognostic factors in patients with small-cell lung cancer (SCLC). BMC Cancer 2017;17:269. [Crossref] [PubMed]
- Gay CM, Stewart CA, Park EM, et al. Patterns of transcription factor programs and immune pathway activation define four major subtypes of SCLC with distinct therapeutic vulnerabilities. Cancer Cell 2021;39:346-360.e7. [Crossref] [PubMed]
- Hardy-Werbin M, Rocha P, Arpi O, et al. Serum cytokine levels as predictive biomarkers of benefit from ipilimumab in small cell lung cancer. Oncoimmunology 2019;8:e1593810. [Crossref] [PubMed]