Atrial functional mitral regurgitation in cardiology and cardiac surgery
Introduction
One of the most prevalent cardiac valvular abnormalities in the United States is mitral regurgitation (MR) (1). It can be classified as organic [structural abnormalities of the mitral valve (MV)] or functional (structurally normal leaflet tissue). A prevailing opinion among researchers in this field of study is that functional MR (FMR) results from the restriction of leaflet motion, which is caused by a vector shift of the papillary muscles (PMs) of the left ventricle (LV). It is clear that displaced PMs tether the MV leaflets into the LV cavity, preventing the MV from coapting adequately despite the closing forces imposed by left systolic pressure (2,3). In a clinical setting, the most common context for diagnosing FMR is in the context of an ischemic infarction of the inferoposterior wall, as well as in cases of dilated cardiomyopathy (4-6).
In their study, Gertz and his team (7) provided definitive evidence that FMR is not restricted to in vivo observations of LV remodeling. They also demonstrated that it can arise in patients with left atrial (LA) and mitral annular enlargement caused by atrial fibrillation (AF). In this study, the researchers conducted a retrospective analysis of 53 patients with AF and a LV size that was within normal limits, in addition to an ejection fraction (EF) of at least 50%. These individuals were initially referred for catheter ablation. The study subjects exhibited significant (moderate to severe) MR with structurally unimpaired leaflets and were therefore not eligible for surgical ablation. One year after catheter ablation, follow-up echocardiography revealed that patients with recurrent AF had significantly larger LA volumes and annular sizes, as well as a >3 times higher rate of significant MR (82% vs. 24%; P=0.005). It is noteworthy that mitral annular size was the most significant independent determinant of meaningful MR. Gertz and colleagues (7) distinguished atrial FMR (AFMR) from ventriculogenic FMR (VFMR) in patients with LV remodeling by defining the former as a separate entity.
There is considerable variation in the prevalence of AFMR across studies, reflecting differences in definitions, diagnostic modalities, study designs, and populations examined. The study conducted by Gertz and colleagues (7) revealed that 7% of patients exhibited significant AFMR when assessed by transthoracic echocardiography. A study that included patients who had already been diagnosed with AF and were undergoing a trans-thoracic echocardiogram revealed that 3% of those with AF for 1 year exhibited significant MR, compared to 28% of those with AF for over 10 years (8). This cohort of patients with severe MR and preserved LV systolic function had AFMR identified in almost 8% of those undergoing transesophageal echocardiography (8). The final word on this comes from a community-based study performed in Olmstead County: 27% of individuals with significant MR had AFMR, 38% had VFMR, 32% had primary MR, and 2% had MR of mixed etiology (9).
Another significant etiology of AFMR is heart failure (HF) with preserved ejection fraction (HFpEF) (10). The development of LA enlargement and mitral annular enlargement in HFpEF is a consequence of chronic LA hypertension resulting from LV diastolic dysfunction. It is important to note that AF occurs in 15% to 40% of patients with HFpEF likely due to atrial fibrosis, a well-established proarrhythmic substrate (11).
It has been proposed that controlling the rhythm of AF rather than the rate may preclude the development or ameliorate AFMR. However, this hypothesis has not been subjected to rigorous investigation (12). In addition, published reports and ongoing research vary in their definition of AFMR (2). This overview examines the pathophysiology of AFMR and emphasizes the necessity for a uniform definition of AFMR. This will inevitably result in enhanced data integrity and a deeper comprehension of the management and treatment of AFMR (13-19) (Figure 1).
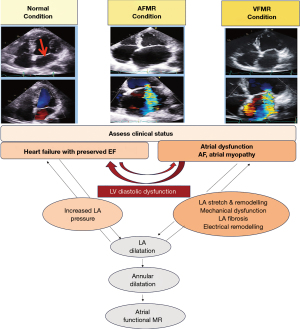
Definition of isolated AFMR
Despite the growing body of evidence on the clinical manifestations and epidemiology of AFMR, the underlying histopathological alterations remain incompletely understood. This is largely due to the dearth of autopsy studies in this field. It can be postulated that the pathogenesis of this disease is based on anatomic principles. The base of the LA and the posterior mitral annulus to which it is enclosed are located within the LV inlet (20). Therefore, it is logical that when these structures widen, they must emergently ex-cede the confines of the ventricle. The displacement of the annulus towards the crest of the LV free wall results in the posterior mitral leaflet (PML) becoming tethered in a superior direction (towards the basal region). Meanwhile, the contracting PMs tether the leaflet in an inferior direction (towards the apical region), thus limiting its role in the process of coaptation. These geometrical changes, known as atriogenic leaflet tethering (ALT), were first identified by Silbiger (21). Subsequent identification was made echocardiographically by Machino-Ohtsuka and colleagues (22), as well as by Ito and colleagues (23). ALT is a clear indication that both Carpentier type 1 (annular enlargement) and Carpentier type IIIb (PML restriction) MR are present.
In consideration of the previously outlined pathogenic mechanisms, it is evident that isolated AFMR can unambiguously and precisely be described and characterized as FMR. The subsequent primary characteristics, depicted in Figure 2, are fundamental for an understanding of isolated AFMR. (I) Both the global and regional LV cavity and systolic function are within the normal ranges. Additionally, there is no vectorial shift of PMs. However, it should be noted that the LV may dilate during later stages of significant AFMR. (II) There is evidence of mitral annular dilation and LA enlargement. (III) The mitral valve’s systolic leaflet does not demonstrate the expected concave orientation towards the LV (24,40-45).
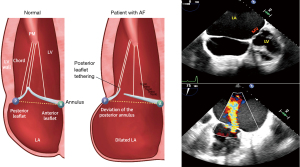
It is conceivable that the degree of tethering may result in the leaflet coaptation point shifting apically to the line connecting the annular hinge points in an apical four-chamber view. Nevertheless, this is not a precise indication and may vary (24,40-45). It is important to note that two features that do not define AFMR, increased leaflet thickness and MR jet direction, are not validated in this framework.
Increased leaflet thickness
It is well established that valve regurgitation can frequently result from thickened leaflets caused by fibrosis and cellular conversion of the valve (25-27,46-49). Similarly, the same thickened leaflets have been identified in individuals with AFMR (24,40-45,50). This is consistent with the inflammatory nature of AF and AFMR-associated HF, which fosters profibrotic cellular and matrix alterations in the valve (10,24,25,28,41,42,44,45,48,51). Nevertheless, there are instances where more leaflet thickness is not consistently noted.
MR jet direction
A central jet of MR is a defining feature of AFMR and a common occurrence in VFMR. However, jets of an eccentric look do in fact arise from a number of diverse mechanisms. (I) Changes in VFMR may result in the overshoot of one leaflet, which will cause a jet to be ejected from the valve in an eccentric manner. (II) An alternative explanation is that the spatial configuration of the MV is tethered to a shorter posterior leaflet excursion, which results in a more posterior jet origin (the Coanda effect) (21). Adherence to the LA posterior wall gives the appearance of an eccentric jet. In reality, the jet is centered and drawn towards the adjacent LA wall (3,22,27,50). Multiple independent reports have confirmed a connection between posterior leaflet tethering angles and AFMR (8,23,26,52). This subtype of AFMR is relatively rare but tends to reach worse results [see Figure 2 (21,24-39)].
Clinical framework and potential coexisting status in AFMR
AFMR is mostly caused by disproportionate LA enlargement and mitral annular dilation, which leads to leaflet tethering and malcoaptation (15,17,21,22,46). There are two usual clinical setting that result in disproportionate LA enlargement and subsequent mitral annular dilation in the context of preserved LV function (26,46,47). Both AF and HFpEF are syndromes caused by LA remodeling and dysfunction, which must be considered when diagnosing and treating these conditions. AFMR is both a cause and consequence of atrial remodeling—a fact that has been well established (52). Elevated pressure levels within the heart can result in detrimental alterations to the anatomical and functional structure of the left atrium in patients with HFpEF. It is essential that the LA be adequately dilated in order to achieve optimal compliance and reservoir function. Failure to perform this procedure may result in a reduction in the function of the LA booster pump. Both AF and HFpEF are associated with shared cardiovascular risk factors, which contribute to the exacerbation of both conditions.
Recent data clearly shows that hidden HFpEF is common among patients with AF who also have breathing difficulties, especially among those who don’t fully recover after sinus rhythm restoration (38). This has prompted various groups to identify a subtype of AFMR involving AF dominating HFpEF. This subtype is clearly distinguished by more severe LA myopathy that doesn’t correlate with the extent of LV failure (53-55). The unquestionable indicator of the advanced LA myopathy subtype in HFpEF is the presence of AFMR. This results in inadequate hemodynamics and exercise flow rate (56,57). A study conducted in a community setting found that approximately half of patients diagnosed with moderate-to-severe isolated MR had previously suffered from AFMR (9). The degree of severity of MR was not a significant factor in determining the outcomes associated with AFMR. Correction of LA myopathy demonstrated that the correlation was insignificant. This finding definitively proves that the degree of LA myopathy is more crucial than AFMR itself. This is yet more proof that treating AFMR in HF with preserved eject fraction improves LA myopathy and outcomes.
It is clear from the evidence that patients with AFMR demonstrate abnormal LA mechanics with reduced LA (storage) strain (8). Kuppahally et al. (58) demonstrated that patients with reduced LA strain exhibited a significantly higher prevalence of substantial atrial fibrosis as determined by delayed-enhanced cardiac magnetic resonance (CMR) imaging. It has been definitively demonstrated that reduced LA strain is inversely correlated with reduced atrial compliance. It has been definitively observed that reduced LA strain in patients with AFMR is correlated with higher mean LA and pulmonary artery systolic pressures. These findings indicate a significant increase in the LA V-wave height, disproportionate to the MR regurgitation volume. This is likely a result of poor LA compliance and warrants further investigation (59). Furthermore, the available evidence is not sufficiently robust to determine the causal sequence among AFMR, LA myopathy, and HFpEF. It seems reasonable to hypothesize that there is a bidirectional relationship between AFMR and LA myopathy (36). To direct the aforementioned questions, it is imperative that further prospective studies be conducted.
Mechanism of AFMR and its implication in VFMR
FMR states that LV dysfunction is defined as a misbalance of forces that cause the MV to close during systole. The phenomenon is attributed to the augmented tethering forces and diminished closing forces generated by the LV (24,40-45). It is evident that tethering arises when the mitral leaflets are anchored via displacement of the PMs or annular dilation, either independently or in concert. Therefore, in the context of a typical LV geometry and feature, localised annular enlargement, which is a hallmark of AFMR, can lead to enhanced MV tethering (25,27,51,60-63). MR can be caused by an enlarged annulus that weakens leaflet alignment. This is not an exclusive only issue with AF. It can arise from any related annular expansion. It can be reasonably assumed that decreased annular area movement may also result in increased tethering (2-4,7-10). The phenomenon in question can be attributed to the expansion of any ad hoc annular structure, which can be demonstrated by the following points.
Systolic MR may display a biphasic pattern in many cases
In VFMR, MR mitral is characterized by two distinct peaks during the early and late systolic phases. These peaks exemplify the interplay between the closing forces and tethering. As a result, when the peak LV systolic pressure compels the MV leaflets to close, there is a reduction in the effective regurgitant orifice area (EROA) of the MR in mid-systole. This has been demonstrated in several studies (27,42-45,60,61,64-66) Nevertheless, additionally research is necessary to ascertain whether these mechanisms are applicable to AFMR.
Variable displacement of the point where the leaflets meet should be minimized for optimal cardiac functioning
In the event that tethering is observed, it can be noted that the leaflet coaptation point is displaced in the apical direction, away from the annular hinge points, in an apical four-chamber view. The degree of displacement is less pronounced in this instance than it is in VFMR. However, the shift depends on the extent of the annular stretch and the compensation of the leaflets, resulting in a variable level of regurgitation (2-6). Consequently, apical displacement of the coaptation site represents a relatively minor mechanism in AFMR, when compared with the more significant effect of loss of leaflet concavity relative to the LV. The AFMR results in a mild tethering of the leaflets, as a consequence of the necessity for the leaflets and chordae to span the distance between the PM and the annulus. Despite the absence of motion observed in the PM as a consequence of LV remodeling, the persistence of leaflet tethering remains evident. It is important to highlight that there is a distinct lack of tethering in the realm of valve adaptation; this will be addressed in greater depth at a later point. An increase in the area of the leaflets serves to offset the effects of annular dilation, thereby restoring the leaflets to their normal position (2-6,8-10).
Decrease or leakage of the standard leaflet concavity towards the LV
Usually, when the LV is exerting pressure, the leaflets curve inwards towards it (as endorsed in Figure 3) (23,24,39-45). When opposing forces act against LV pressure, the leaflets lose their curve or curve inwards towards the LA. These opposing forces are transmitted through the chordae tendineae, which result in a straightening of the MV.
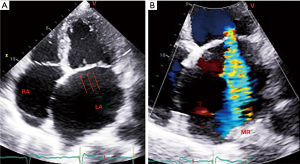
MV leaflet maladaptation
The existence of MV leaflet maladaptation is well established in both AFMR and VFMR. It is evident that mechanical shift of the PM results in mitral leaflet stretch, which subsequently initiates de novo growth in the surface area of the mitral leaflet (27,40,43,46-48). This in vivo evidence demonstrates that the restoration of the embryonic growth mechanism—endothelial-to-mesenchymal transformation—is responsible for the observed growth. Following a myocardial infarction, there are clear changes in the valve. The valve becomes smaller and stiffer, and its coaptation ability is impaired (26,27,46-50). Additionally, annular expansion is correlated with a de novo elevation in leaflet area (4,7). Nevertheless, this expansion is inadequate for larger annular regions, resulting in MR due to leaflet failure (23,24,43-45). A noteworthy aspect of this process is the lack of correlation between the adaptation of the valve leaflets and the posterior-predominant increase in the size of the expanding annulus (23,24,27,43-45,61,65). The presence of maladaptive de novo alterations of the valve in AFMR is accompanied by a notable thickening of the leaflets in these individuals (4,44,45). This finding aligns with the inflammatory typology associated with AF and AF-related HF. Furthermore, the observed endothelial-to-mesenchymal processing in the atrial wall of patients with AF is consistent with the findings (66-69). The aforementioned deficiencies with regard to leaflet adaptations are observed irrespective of significant AFMR developments. It remains to be seen whether a favourable change (8,10), as observed in postinfarction VFMR, can be achieved.
Epidemiology and evolution of AFMR
The increasing prevalence of AFMR and its impact on clinical outcomes are becoming increasingly apparent. It is hypothesized that as much as 33% of the cases of isolated and moderate-to-severe MR in Olmsted County may be attributable to AFMR. The majority of the patients were elderly women, and that a significant proportion of them exhibited a number of cardiovascular risk factors, along with AF (9,70). Although the condition appears benign with a relatively small EROA, ranging from 0.08 to 0.20 cm2, it was found to be associated with significantly more unfavorable outcomes, including impaired LV diastolic function, increased systolic pulmonary artery pressure, and elevated rates of HF (9,70) when compared with those presenting with high-volume degenerative MR.
A further recent retrospective investigation, which concentrated on severe MR, corroborated the unfavorable outcomes observed with regard to degenerative MR (8).
Moreover, AFMR was found to be associated with a higher 5-year mortality rate (up to 50%) than that of age- and gender-matched control arms (9,70). Nevertheless, it is crucial to acknowledge that the precise predominance of potential underpinning HFpEF could not be quantified through the epidemiological investigations conducted, as it was deemed highly likely.
In particular, the European Society of Cardiology (ESC) Heart Failure Registry estimated that 20% of patients with HFpEF exhibited moderate-to-severe FMR (71).
A review of the Acute Decompensated Heart Failure Syndromes (ATTED) registry (72) revealed that 1,800 individuals presenting with acute decompensated HFpEF exhibited a higher predominance of mild or severe FMR at discharge, regardless of whether they had cardiovascular risk factors. Furthermore, mild MR was definitively related to hostile outcomes. This was observed regardless of whether patients were undergoing any pharmacological treatment and irrespective of whether they had AF. The adjusted hazard ratio was calculated at 1.40 for all-cause mortality and HF readmission, which is a significant figure. Currently, there is a paucity of data regarding the relationship between moderate-to-severe AFMR, LA myopathy, and subsequent prognosis. It is reasonable to hypothesize that the plethora of co-morbidities in patients with AFMR may be a contributor to an unfavorable global outcome. Nonetheless, latest epidemiological investigations have indicated that even mild forms of AFMR can have a deleterious impact. This indicates that minimized regurgitant volume may represent a significant prognostic factor in a nondilated, noncompliant LV with restrictive physiology as evidenced by the disproportionality concept of VFMR. Furthermore, there is a paucity of data concerning the dynamic characteristics of AFMR. In addition, it remains to be determined whether AFMR or underpinning LA myopathy in HFpEF contributes to poor hemodynamics and outcomes. A randomized controlled prospective trial is needed to determine whether reducing moderate or worse AFMR can improve patient prognosis, as both conditions often coexist. HFpEF is an effective treatment option, particularly since the presence of substantial leaflet tethering can complicate the management of AFMR.
Proposed approach for assessing the severity and quantification of MR
In accordance with the American Society of Echocardiography’s guidelines, a built-in strategy to assess the severity of AFMR is recommended (73). This involves utilizing color Doppler parameters and precise quantitative measurements of EROA and regurgitant volume, in conjunction with qualitative supporting signs including the density, profile and duration of the MR jet on continuous wave Doppler, as well as a pulmonary vein flow pattern and the mitral inflow E-wave velocity (74,75).
A critical consideration is the timing of the echocardiographic evaluation. Cardioversion can significantly improve moderate or severe MR in individuals with sudden occurrence of trial fibrillation and accelerated ventricular response (10). Similarly, the recovery of sinus rhythm through ablation can markedly diminish the severity of MR in patients with AFMR.
However, assessment of the severity of MR is complicated by the presence of AF, especially in the presence of rapid or extremely irregular rhythms. Measuring MR severity, LV and LA volumes and strain during sinus rhythm or AF with a controlled heart rate and minimal R-R variation is strongly recommended (7). The indexed beat method can be utilized when selecting a beat for which both the MR jet area and the volumes of both the LV and LA, respectively, are required for analysis. Comparative analysis of the R-R intervals prior to and following the observation under consideration indicates a range of similarity. Nevertheless, the specific methodology employed in most AFMR studies is insufficient to provide insight into the timing of measurements, the number of beats measured, heart rate, and R-R variability in AF. The dearth of such granular details renders it challenging to comprehend published reports and to contrast disparate research findings.
Even in the absence of confounding factors, AF presents a challenge to the majority of conventional methods for assessing MR. For instance, the volumetric method (which subtracts LV outflow stroke volume from either LV stroke volume or mitral inflow stroke volume) presents additional challenges in the context of AF, given the inherent difficulties in accurately measuring cardiac function in this clinical scenario, dependent to the impact of heart rate variability. In the context of AF, the combination of an error in measurement and the 16–28% beat-to-beat variation in stroke volume reported in AF (76,77) renders the volumetric approach unsuitable.
The variability of the regurgitated orifice area, whether observed during an intra- or inter-beat period, poses a challenge for the difficult proximal convergence method. In addition, the common elliptical aperture shape observed in AFMR leads to an underestimation of MR when the standard proximal convergence formula is applied. This method may be improved in the future with advances in three-dimensional (3D) color Doppler. One has to acknowledge that even some of the indirect indicators of the degree of regurgitation may prove less reliable. One such indicator is pulmonary venous Doppler. It may show decreased systolic flow due to AF or increased LA pressure alone, without MR [Figure 4 (3)].
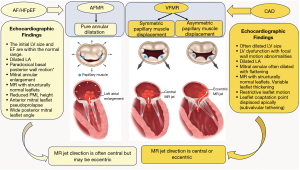
In order to accurately quantify the severity of AFMR, it is essential to determine the normal size and function of the LV. A number of published reports on AFMR have proved that LV end-diastolic volume (LVEDV) remains within the normal range (3,4,7,8). This indicates that in cases of significant MR, the regurgitant volume is often lower than the typical 60 milliliters observed in primary MR and a remodeled, enlarged LV. A total stroke volume of 60 mL would result from a typical LVEDV of 100 mL and a 60% left ventricular ejection fraction (LVEF). However, 60 mL regurgitant volume, commonly associated with severe MR, is not achieved. The regurgitant fraction has been estimated to be approximately 50% in an individual with an EROA of 0.2 cm2 and a regurgitant volume of 30 mL. Consequently, the assessment of the severity of AF with mild MR in patients with an appropriate LV size and function may necessitate the consideration of lower, quantifiable measures of regurgitant volume. There has been evidence that large LA volumes may be associated with AFMR. In addition, if the LA is compliant, it may be capable of tolerating the regurgitant volume. However, LA stiffening may occur even with low regurgitant volumes after extensive ablation, resulting in elevated LA pressures (78). To clarify the prognostic significance of both EROA and regurgitant volume in AFMR, large cohort studies would be required. Nevertheless, these studies will necessitate meticulous attention to both quantitative methodology and temporal aspects, as previously observed in this review [Figure 4 (3)].
The absence of longitudinal investigations may hinder our understanding of the pathophysiology and natural history of the disease. For example, AFMR may indicate that LV dilation occurs concomitantly with an enlargement of the mitral annulus and left atrium. The sequence of events can be most precisely assessed when successive studies explicitly identify the sequence of events. Subsequent, more prolonged studies are necessary to address this issue. Longitudinal studies must be conducted to elucidate the natural history of AFMR and to assess the progression rate to VFMR [Figure 4 (3)].
Evaluation of AFMR with magnetic resonance and cardiac computed tomography (CT)
It is recommended that two-dimensional (2D) and 3D echocardiographic techniques be employed to optimise the assessment of LV size, function, mitral apparatus, annular size, and LA size in order to identify the underlying mechanism of MR. Echocardiography is currently the most widely used method for evaluating MV morphology and hemodynamics. Its explanatory nature and accessibility make it an ideal choice for this purpose. In instances where echocardiography is not an appropriate diagnostic tool or additional information is required, CMR and CT can be employed to assess AFMR. In the event that echocardiography is of insufficient quality, CMR and CT can provide a 2D or 3D assessment of mitral leaflet morphology, mitral annular size, and its geometric impairment across the cardiac cycle. Additionally, they can assess tethered leaflet motion and orifice opening. Both CMR and CT are not constrained by inadequate imaging windows or the subject’s body shape. Moreover, they can accurately assess the size and function of the LV and left atrium in real time. Nevertheless, cardiac gating is necessary for CMR and CT, and there is a potential for a decrease in image quality and difficulty in quantifying LVEF in patients with AF due to an irregular cardiac rhythm. CMR offers a comprehensive approach to cine imaging of the mitral apparatus. By precisely measuring the severity of MR, it enables the assessment of relevant hemodynamics. CMR has the potential to outperform CT in accurately detecting and characterizing annular sprains or anomalus patterns of leaflet motion due to the high temporal resolution of cine imaging (typically 40–45 ms). CMR phase-contrast imaging offers precise measurement of aortic systolic blood flow, which allows for the derivation of mitral regurgitant volume and regurgitant fraction. This is achieved by subtraction of LV stroke volume, which can be obtained from cine imaging. The use of CMR to quantify mitral regurgitant volume and fraction has been shown to be more reproducible than echocardiography (78). In addition, CMR provides a comprehensive assessment of LV remodeling. It includes measurements of chamber size, LV function, and tissue fibrosis (79,80). In patients with normal sinus rhythm, CT angiography is an accurate assessment of annular geometry as well as ventricular and atrial volumes. Nevertheless, the absence of hemodynamic information precludes the use of CT angiography in establishing the severity of MR. In patients with FMR who undergo percutaneous edge-to-edge mitral repair, CT angiography has demonstrated the potential to predict procedural success (81,82).
Characterizing the pattern of myocardial scar, fibrosis, or infiltration using CMR imaging techniques such as late gadolinium enhancement imaging and T1 mapping can provide highly informative information about the severity and origin of the underlying cardiomyopathy that may predispose to the development of AFMR in individuals who have a number of risk factors for HFpEF or who have undergone thoracic radiation therapy and have evidence of impaired LV diastolic filling. CT is capable of identifying and characterizing calcification within the mitral apparatus. The former is characterised by the formation of calcium deposits around the MV, whereas the latter involves the presence of caseous material, which is a type of necrotic tissue. In both cases, the calcification can lead to MR.
Treatment
Medical treatment and rhythm control
The Japanese Circulation Society’s 2020 guidelines for the management of heart valve disease suggest Class I recommendation for standard HF therapy for patients with AFMR who present with symptoms (83). It is recommended that the treatment regimen comprise diuretics, which have been shown to have the potential to reduce the volume of the left atrium.
A number of studies have indicated that the reduction of AFMR can be achieved through a combination of two main strategies: promoting atria/annular reverse remodeling and re-establishing atriogenic and ventriculogenic contributions to annular contraction. These approaches have been demonstrated to be effective in some minor clinical studies (14,18,84,85) A study revealed a decrease in the mean effective regurgitant orifice area from 0.27 to 0.15 cm2 in 15 patients with persistent AF following an electrical cardioversion (84). Additionally, another research study demonstrated a mean reduction in vena contracta width from 0.40 to 0.21 cm in 47 patients with paroxysmal or persistent AF following catheter ablation and/or electrical cardioversion (18). In their investigation, Masuda and associates (86) observed that a substantial proportion (greater than three-fifths) of individuals diagnosed with moderate AFMR demonstrated at least one improvement in MR grade following catheter ablation. Additional in-depth research is necessary to determine the effect of rhythm control on individuals afflicted by AFMR. The existing corpus of evidence regarding the impact of rhythm control approaches on clinical prognosis in individuals with AFMR is currently inadequate to allow for a definitive conclusion to be drawn. A clinical investigation revealed that catheter ablation is an effective intervention, reducing hospitalization for HF and stroke by a significant margin when compared to subjects who received the best available treatment, including calcium channel blockers, beta blockers, digoxin, and antiarrhythmic medications over a 3-year study period (87).
In addition to the aforementioned effects of decreasing myocardial MR and enhancing LVEF, rhythm control is also associated with the mitigation of circulating brain natriuretic peptide levels, as demonstrated in studies involving patients with HFpEF and AF (86,88). The impairment of diastolic function can be evidenced by a decline in the ratio between transmitral E-wave velocity (E) and mitral annular E0 velocity (E/E0) and the strain rate during isovolumic relaxation (E/SRIVR) (89). The Japanese Circulation Society’s 2020 guidelines for managing heart valve diseases suggest that catheter ablation may be a suitable option for patients with symptomatic persistent AF and severe MR. This approach is classified as a Class IIa recommendation, contingent upon the likelihood of maintaining sinus rhythm.
Surgery
The 2020 Japanese Circulation Society guidelines for heart valve disease management indicate that surgical intervention represents a Class IIa recommendation for the treatment of symptomatic patients with severe MR who have demonstrated an inadequate response to standard HF therapies (83). Conversely, the 2020 heart valve disease guidelines issued by the American College of Cardiology/American Heart Association (ACC/AHA) suggest surgery as an alternative treatment in symptomatic patients exhibiting severe MR, who have not responded to therapy for HF, AF, or comorbidities (90). The two recommendations are based on consensus (level of evidence: C), and further research is necessary to resolve the inconsistencies between them. In the context of valvular heart disease, the 2021 ESC guidelines for the management of AFMR currently lack formal recommendations for treatment. Nevertheless, the guidelines do acknowledge that surgical intervention and catheter ablation have proven to be efficacious treatment modalities, although the evidence supporting this assertion is limited (91).
There are several effective procedures for treating AFMR. These include addressing the MV annular component, correcting any leaflet prolapse, and restoring a normal heart rhythm (6,14-18). It is widely acknowledged that MV surgery typically involves restricted ring annuloplasty (92-95). It can be hypothesized that a significant annular area may hinder the coaptation process, particularly when the ring is substantially undersized. This, however, may potentially increase the risk of dehiscence and mitral stenosis. To avoid this complication, we have developed a new, highly effective annuloplasty procedure that involves performing a doble row overlapping suture. This procedure will reduce the risk of dehiscence. In addition, it offers a more effective solution for leaflet restriction and a significant improvement in the tenting volume. We can confirm that there was no MV stenosis (96). In most cases, pseudoprolapse of the anterior mitral leaflet can be effectively addressed through restrictive annuloplasty (96).
Another potential approach to consider is the use of autologous pericardium for patch augmentation of the PML (97). A potential limitation to the durability of this repair must be considered, given the tendency for leaflets to shrink and stiffen. However, it is crucial to highlight that the most compelling results, based on extensive follow-up data, were observed in patients with rheumatic MV disease who were treated with an anterior leaflet enlargement patch (98,99).
A two-stage MV repair procedure that includes the valvular and subvalvular component with a relocation of the PMs is only indicated in the presence of a VFMR that is documented by a displacement of the PMs. In these patients, ventricular remodeling inevitably leads to dyssynchrony in the normal contraction of the PMs. If the leaflets are significantly tethered, undersizing alone may not be enough to restore normal geometry (8,100-106). In such cases, MV replacement may be a possible option to consider. MV replacement must be aimed at preserving the subvalvular apparatus to ensure the preservation of the architecture of the LV. This is the only way to prevent short-term ventricular dilatation (107,108).
The precise purpose of restoring sinus rhythm during surgical repair of the MV in patients presenting with AFMR remains uncertain. In a retrospective study, it was observed that patients who underwent concurrent Cox maze IV procedures appeared to experience a higher level of freedom from recurrent MR after 3 years, with a 94% success rate, compared to a 44% success rate among those who did not undergo the procedure (109). Although the evidence presented may appear to be compelling, an analysis of 11 AFMR surgical series has demonstrated that only 50% of persons who undergo MV repair undergo either the Cox maze or the CryoMAZE procedure (92,93).
In the context of preferred surgical choice for AF, it is imperative to consider both the duration of the condition and the size of the left atrium (110). As regards the latter, restoring sinus rhythm is rendered unlikely when the diameter of the left atrium surpasses 6 cm (111). Additionally, tricuspid regurgitation (TR), caused by enlargement of the tricuspid annulus, often occurs concomitantly with MR and AF (112).
In accordance with the 2020 Japanese Guidelines for Atrial Fibrillation and Mitral Regurgitation, surgical intervention of the tricuspid valve is advised for patients with AF and MR, as well as for patients with concomitant TR. Nevertheless, the specifics pertaining to tricuspid valve intervention with regard to annular size, the severity of TR, and right ventricular function remain unclear. This represents a Class IIa recommendation (83).
The potential benefits of addressing AF during tricuspid valve repair surgery in reducing the burden of TR remain uncertain, as there has been no systematic examination of this topic. Nevertheless, a study of patients who underwent MV surgery for MR and/or mitral stenosis in conjunction with tricuspid valve repair for mild to moderate TR demonstrated that failure to address AF during surgery was associated with an increased risk of a composite endpoint that included tricuspid valve reoperation, HF, and mortality. A few case series have indicated a reduction in the severity and symptomatology of MR following MV surgery (113-118). Deferm and colleagues (84) reported on the surgical outcomes of 97 patients with moderate to severe MR. Half the patients underwent tricuspid annuloplasty, and 30% had a Cox maze IV procedure. After 5 years, the mortality rate due to all causes was 15%. The hospitalization rate for patients with HF was found to be 13% in this study. Sixteen percent of patients experienced a moderate or severe MR recurrence within 5 years of their initial diagnosis.
To date, the largest series of patients with AFMR undergoing surgical intervention has been reported, comprising 123 patients. In all patients, mitral annuloplasty was performed (111). In addition, 50% underwent tricuspid annuloplasty, and 60% received the maze procedure with LA appendage removal. In this collective of patients, the 5-year survival rate was approximately 75%. A follow-up examination conducted at an advanced stage of the disease revealed that 5% of patients exhibited recurrent moderate-to-severe MR (defined as a grade of >2). A median follow-up period of approximately 3 years revealed that nearly three-quarters of patients maintained sinus rhythm (111).
It’s so important to have a team of experts looking after patients with AFMR. This team should include HF experts, interventional cardiologists, arrhythmia cardiologists, cardiac surgeons and HF managers. The team works together to help the patient find the best way to get better. They use the best treatments available (83,90,91). For those with advanced disease and a life expectancy of less than a year, and for those with severe co-morbidities, it might be helpful to consider referring them to palliative care. The patient’s journey may take a different path than AFMR surgery. Sometimes, additional interventions may be needed, such as coronary artery bypass grafting, management of severe TR, and direct mechanical intervention to correct arrhythmias (90,91).
It’s so interesting how many different geometrical laws have been used to explain the success or failure of restrictive mitral annuloplasty (RMA) (63,95,103,106,119). There’s been a lot of excitement recently about subvalvular apparatus surgery, such as papillary muscle approximation (6,103), and PM sling (102,104,105,120), because it’s clear that we need a more holistic approach to treating MR that includes both the MV and LV with subvalvular apparatus. These procedures are designed to help relieve the geometric stress on the LV and the valve tethering caused by an asymmetrically dilated LV.
A lot of time and effort has been put into figuring out the best way to restrict the annulus for mitral annuloplasty. This is to make sure that the risks of stenosis, systolic anterior motion, and recurrent MR don’t happen because of overcorrection or undersizing (94,102). From a biomechanical perspective, undersizing the annulus would result in forces on the heart’s fibrous skeleton that may not align with the expected displacement vectors relative to LV dilation in these patients. It is possible that an imbalance of internal forces within the ventricle could potentially lead to a recurrence of MR. This is thought to be due to a shift towards an unstable mechanical equilibrium (50,63,121,122).
Percutaneous intervention
Minimally invasive procedures for the treatment of MV regurgitation in patients with AF are currently available in three categories: edge-to-edge repair, direct annuloplasty, and indirect annuloplasty. Examples of devices used in these procedures include: MitraClip from Abbott Vascular, PASCAL from Edwards Lifesciences, Cardioband Mitral System from Edwards Lifesciences, and Millipede IRIS Ring from Boston Scientific for direct annuloplasty; Carillon Mitral Contour System from Cardiac Dimensions for indirect annuloplasty. A paucity of data is available regarding the utilization of transcatheter heart valves in patients presenting with AFMR. A number of retrospective studies have examined the efficacy of transcatheter edge-to-edge repair in patients with AFMR (123-126).
A multicenter study conducted by the Spanish MitraClip registry (124) revealed that MR was acutely reduced from a mean of 3 to a mean of 4+ down to a mean of ≤2+ in 94% of patients. Prior to the procedure, 90% of patients exhibited functional limitations consistent with New York Heart Association (NYHA) classification in functional classes III to IV. Following 12 months, 20% of patients demonstrated functional limitations in NYHA class III, while none were classified as NYHA class IV. Nevertheless, a significant number of patients (20%) exhibited a recurrence of MR above grade 2 within the initial 12-month period. Furthermore, a 12-month median follow-up period was recorded in the MITRA-TUNE registry, a multicenter Italian registry dedicated to the transcatheter treatment of FMR. The findings indicated an MR recurrence rate of approximately 11% over a mid-term period (125). This evidence lends support to the hypothesis that there has been a consistent reduction in the anteroposterior and intercommissural annular dimensions (125). It is noteworthy that a reduction in the anteroposterior dimension subsequent to transcatheter edge-to-edge repair is associated with fibrosis induced by the MitraClip. This ultimately results in the de novo formation of a tissue bridge between the anterior and posterior segments of the annulus. However, it remains to be seen how this long-term structural modification will affect the severity of MR (125,126).
The European Registry of Transcatheter Repair for Secondary Mitral Regurgitation has documented a total of 126 cases of symptomatic AFMR, which is the most extensive group of patients with the condition to date. The registry data affords insights into real-world settings. A total of 87% of registrants achieved procedural success, which is defined as a MR of 2+ or less. The 2-year survival rate was 70%. Prior to repair, approximately 90% of patients were classified as belonging to NYHA functional class III/IV, whereas approximately 40% were classified as belonging to this class following the procedure. In addition to the presence of NYHA functional class IV and right ventricular dysfunction, defined as a ratio of tricuspid annular plane systolic excursion to pulmonary artery systolic pressure below 0.34 mm/mmHg, were identified as significant, independent predictors of 2-year survival (127).
A recently conducted substudy of the COAPT (Cardiovascular Outcomes Assessment of the MitraClip Percutaneous Therapy for Heart Failure Patients with Functional Mitral Regurgitation) trial (62) compared echocardiographic characteristics of patients exhibiting and not exhibiting AF. It is noteworthy that patients with AF demonstrated a less pronounced reduction in LVEF and a lower incidence of LV enlargements, while exhibiting a higher prevalence of LA and mitral annular enlargements. The investigators of the COAPT study concluded that these modifications exhibit a distinctive FMR phenotype, exhibiting characteristics of both AFMR and VFMR. The enlargement of both the atria and the annulus are largely attributed to the effects of AF. Conversely, the development of LV abnormalities is primarily the result of severe MR-related volume overload.
Despite the lack of extensive investigation, it can be posited that the occurrence of recurrent MR following transcatheter edge-to-edge repair may be predicted by several key factors. These include a wide posterior leaflet angle, the presence of significant annular enlargement, pseudoprolapse and jet eccentricity, and a shortened residual PML. The Italian MITRA-TUNE registry revealed that patients with a pre-procedural inter-commissural annular diameter exceeding 34 mm exhibited worse outcomes, including higher all-cause mortality and HF hospitalization rates (125).
Further investigation is warranted. However, it seems prudent to conduct routine echocardiographic monitoring for recurrent MR following transcatheter edge-to-edge repair, particularly in patients with elevated-risk MV morphology and/or eccentric jets. While transcatheter edge-to-edge repair is capable of reducing MR and improving symptoms, its approach to treating annular issues is valvular (125). However, it is currently unclear whether restoring sinus rhythm to address annular enlargement results in an additional reduction in MR, and further randomized trials are needed to assess the efficacy of this combined treatment.
For those with AFMR who are at increased surgical risk or who have anatomy that is not conducive to transcatheter edge-to-edge repair (e.g., those with a small annulus or a thick septum), transcatheter annuloplasty devices may be a viable alternative (126,128,129). In a clinical investigation of 15 patients with moderate-to-severe AFMR, implantation of the Carillon device was associated with a significant reduction in annular anteroposterior diameter (4.3 vs. 3.8 cm; P<0.05) and effective regurgitant orifice area (0.28 vs. 0.20 cm2; P<0.05) within 3 months (128). The statistical significance of the result could not be confirmed by a benefit in clinical symptoms because the reported results and short-term follow-up were not sufficiently robust to demonstrate this effect. A second study, which employed a relatively small sample size, compared the efficacy of the Carillon device to that of the MitraClip in managing patients with HF and AFMR. After 12 months, no significant difference in functional status was observed (129).
Moving from an integrated approach to managing AFMR
There is currently no widely agreed upon approach to managing patients with AF and AFMR due to insufficient data. Nevertheless, it is imperative to recognize that patients with AF and mild MR (1–2+) may experience symptoms resulting from LV diastolic dysfunction and must be treated accordingly. While the evidence is inconclusive regarding the efficacy of rhythm control in reducing MR severity, other benefits of this approach justify its implementation. The Early Treatment of Atrial Fibrillation for Stroke Prevention Trial (EAST-AFNET) (130), which was conducted by a team of researchers from multiple countries, demonstrated that restoration of sinus rhythm within 1 year of the onset of AF was associated with a reduction in the incidence of cardiovascular death, stroke, hospitalisation for HF and acute coronary syndrome, compared to a rate-control strategy. The de novo recovery of sinus rhythm is also associated with a reduced probability of haemodynamic decompensation resulting from AF with a rapid ventricular response. It is recommended that rhythm control strategies be pursued regardless of the severity of MR or the presence or absence of symptoms. Patients presenting with advanced MR (grades 3–4) should be referred for surgical or transcatheter intervention, based on the estimated surgical risk and interdisciplinary heart team assessment. Asymptomatic patients with a MR of 3 to 4+ require periodic clinical and echocardiographic follow-up to detect deterioration of LV function, development of pulmonary hypertension, or signs of HF. In the event that these conditions are identified, an evaluation for surgical or transcatheter intervention should be initiated. Furthermore, rhythm control should be considered.
Prognosis
It is of paramount importance to recognize that patients with AFMR represent a high-risk cohort. The patients frequently exhibit advanced age and a considerable array of concomitant conditions, including AF, hypertension, diabetes, and LV diastolic dysfunction. A longitudinal study of patients admitted to the hospital for HF and moderate-to-severe AFMR (89) revealed that over a third of patients required readmission within the median follow-up period of 9 months. In a recent study, Mesi and colleagues (8) observed a significantly higher mortality rate among patients with severe AFMR (41%) compared to those with severe primary MR (19%; P=0.004). It is also worth noting that patients with AFMR and eccentric MR jets exhibited a statistically significant trend toward increased mortality and higher hospitalization for HF compared to patients with central MR jets (P<0.07).
A glimpse into atrial functional TR (AFTR)
AFMR commonly results in AFTR, which stems from the dilation of the tricuspid annulus caused by enlargement of the right atrium (131). TR is the primary disorder in nearly 60% of cases, possibly due to the enlargement of the tricuspid annulus. The co-occurrence of severe AFMR and TR is rare (132).
The tricuspid annulus contracts during normal sinus rhythm, reducing its area by around 30%. This is greatly diminished in AF and is believed to exacerbate the general severity of TR (122). Annular enlargement associated with AFTR avoids the fibrous part of the tricuspid annulus, instead affecting the softer and lipid-rich segment of the annulus situated along the right ventricular free wall. As the septoposterior axis demonstrates the most significant increase in annular size (133,134), the posterior tricuspid leaflet experiences the most notable displacement, leading to uneven regurgitation in the area between the septal and posterior leaflets (134). It is uncertain if patients with AFTR experience adaptive leaflet growth, which could explain the variable severity of TR in individuals with similar levels of tricuspid annular growth. The mechanism of TR varies between AFTR and VFTR. In the case of AFTR, the right ventricle experiences volume overload, which typically restricts the tricuspid leaflets to the annular plane. Because ventricular remodelling tends to spare the more apical segments of the right ventricle that support the PM, leaflet tethering is rarely observed even in cases where TR is significant. In those cases where the right ventricle is affected, the right ventricle takes on a conical shape (134-137). However, in patients presenting with significant ventricular fibrillation with tachycardia due to left heart disease or pulmonary hypertension, the right ventricle exhibits a disproportionately apical shape accompanied by leaflet tethering. In such instances, the right ventricle typically assumes an oval or spherical shape (134,136). It is of paramount importance to recognize that echocardiographic measurement of the effective regurgitant orifice area may be underestimated in AF with TR due to the typical association of this lesion with a hemielliptical rather than a hemispheric convergence zone. Moreover, it is imperative to exercise caution when estimating the severity of TR through hepatic venous pulsed-wave Doppler, as systolic flow reversal may indicate loss of atrial function caused by AF, rather than severe TR (138).
Moderate to severe TR has a negative impact on survival, regardless of LVEF or pulmonary artery systolic pressure (139). Additionally, studies have demonstrated that isolated moderate to severe TR caused by electronic device leads (140) or a flail tricuspid valve can worsen survival (141). A study specifically focusing on patients with severe isolated TR reported that 42% of cases required hospitalization for right HF, 7% of cases resulted in death due to right HF, and the all-cause mortality rate was 29% over a 5-year period (116).
Despite the negative outlook linked to moderate-to-severe TR when it is isolated, surgery is rarely carried out except when there is infective endocarditis. This is likely due to concerns about mortality rates in hospital, which are estimated at around 10% (142), as well as uncertainty surrounding long-term surgical results. As a result, surgical referral is often postponed until right ventricular dysfunction becomes intractable, making surgery unfeasible (143).
The treatment of AFTR is accomplished through the administration of diuretics. These agents relieve the symptoms associated with the disease by reducing the pressure within the right atrium. The efficacy of rhythm control in reducing TR severity is currently limited by a paucity of data. Nevertheless, one study suggests that restoring normal heart rhythm through catheter ablation or electrical cardioversion can significantly reduce right atrial volume from 29 to 23 mL/m2 and TR vena contracta width from 0.35 to 0.25 cm (12).
The heart valve disease guidelines published in 2020 by the ACC and the AHA assign a Class IIa indication for surgery in patients suffering from symptomatic severe AFTR (90). Surgery has demonstrated noteworthy efficacy in alleviating symptoms, but its effect on survival rate merits further investigation. It is worth noting that surgery is most effective when carried out prior to the onset of right ventricular, hepatic and renal dysfunctions (143). Unfortunately, there is insufficient data available to determine the optimal timing of surgery based on TR severity, annular size, or right ventricular function. When assessing right ventricular systolic function using echocardiography, it is critical to consider that the incompetent tricuspid valve may conceal the true extent of right ventricular dysfunction. Surgery is typically used to treat AFTR, with ring annuloplasty being the most common procedure.
Valve replacement (142) and leaflet augmentation (144) have been proposed as alternatives when there is a high chance of residual TR. The role of percutaneous interventions for patients with AFTR has yet to be established.
Conclusions
Further research is needed to determine cut-off values for left atrium and LV size and function, which will allow us to differentiate between AFMR and mixed AFMR/VFMR. Clinical trials comparing these treatments are necessary. Moreover, it is imperative to determine if integrating these approaches with restoring normal sinus rhythm will result in improved outcomes. There is a strong case for using specialised transcatheter heart valves in the mitral position, and further studies should investigate their effectiveness in treating patients with AFMR with confidence. Further investigation of the molecular basis of endothelial-mesenchymal transition will undoubtedly result in the development of effective new treatments for individuals who lack sufficient growth of their valve leaflets.
Finally, a randomized trial comparing medical therapy with early surgical intervention in patients with AFTR would be a valuable addition to the existing literature (Figure 5).
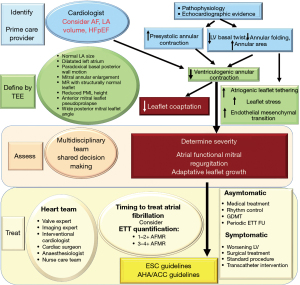
Acknowledgments
Funding: None.
Footnote
Peer Review File: Available at https://jtd.amegroups.com/article/view/10.21037/jtd-24-189/prf
Conflicts of Interest: The author has completed the ICMJE uniform disclosure form (available at https://jtd.amegroups.com/article/view/10.21037/jtd-24-189/coif). The author has no conflicts of interest to declare.
Ethical Statement: The author is accountable for all aspects of the work in ensuring that questions related to the accuracy or integrity of any part of the work are appropriately investigated and resolved.
Open Access Statement: This is an Open Access article distributed in accordance with the Creative Commons Attribution-NonCommercial-NoDerivs 4.0 International License (CC BY-NC-ND 4.0), which permits the non-commercial replication and distribution of the article with the strict proviso that no changes or edits are made and the original work is properly cited (including links to both the formal publication through the relevant DOI and the license). See: https://creativecommons.org/licenses/by-nc-nd/4.0/.
References
- Enriquez-Sarano M, Akins CW, Vahanian A. Mitral regurgitation. Lancet 2009;373:1382-94. [Crossref] [PubMed]
- Farhan S, Silbiger JJ, Halperin JL, et al. Pathophysiology, Echocardiographic Diagnosis, and Treatment of Atrial Functional Mitral Regurgitation: JACC State-of-the-Art Review. J Am Coll Cardiol 2022;80:2314-30. [Crossref] [PubMed]
- Nappi F. Assessing emerging causes of mitral regurgitation: atrial functional mitral regurgitation. J Int Med Res 2024;52:3000605241240583. [Crossref] [PubMed]
- Kumanohoso T, Otsuji Y, Yoshifuku S, et al. Mechanism of higher incidence of ischemic mitral regurgitation in patients with inferior myocardial infarction: quantitative analysis of left ventricular and mitral valve geometry in 103 patients with prior myocardial infarction. J Thorac Cardiovasc Surg 2003;125:135-43. [Crossref] [PubMed]
- Asgar AW, Mack MJ, Stone GW. Secondary mitral regurgitation in heart failure: pathophysiology, prognosis, and therapeutic considerations. J Am Coll Cardiol 2015;65:1231-48. [Crossref] [PubMed]
- Nappi F, Avatar Singh SS, Santana O, et al. Functional mitral regurgitation: an overview for surgical management framework. J Thorac Dis 2018;10:4540-55. [Crossref] [PubMed]
- Gertz ZM, Raina A, Saghy L, et al. Evidence of atrial functional mitral regurgitation due to atrial fibrillation: reversal with arrhythmia control. J Am Coll Cardiol 2011;58:1474-81. [Crossref] [PubMed]
- Mesi O, Gad MM, Crane AD, et al. Severe Atrial Functional Mitral Regurgitation: Clinical and Echocardiographic Characteristics, Management and Outcomes. JACC Cardiovasc Imaging 2021;14:797-808. [Crossref] [PubMed]
- Dziadzko V, Dziadzko M, Medina-Inojosa JR, et al. Causes and mechanisms of isolated mitral regurgitation in the community: clinical context and outcome. Eur Heart J 2019;40:2194-202. [Crossref] [PubMed]
- Deferm S, Bertrand PB, Verbrugge FH, et al. Atrial Functional Mitral Regurgitation: JACC Review Topic of the Week. J Am Coll Cardiol 2019;73:2465-76. [Crossref] [PubMed]
- Kotecha D, Lam CS, Van Veldhuisen DJ, et al. Heart Failure With Preserved Ejection Fraction and Atrial Fibrillation: Vicious Twins. J Am Coll Cardiol 2016;68:2217-28. [Crossref] [PubMed]
- Soulat-Dufour L, Lang S, Addetia K, et al. Restoring Sinus Rhythm Reverses Cardiac Remodeling and Reduces Valvular Regurgitation in Patients With Atrial Fibrillation. J Am Coll Cardiol 2022;79:951-61. [Crossref] [PubMed]
- Sannino A, Smith RL 2nd, Schiattarella GG, et al. Survival and Cardiovascular Outcomes of Patients With Secondary Mitral Regurgitation: A Systematic Review and Meta-analysis. JAMA Cardiol 2017;2:1130-9. [Crossref] [PubMed]
- Nappi F, Antoniou GA, Nenna A, et al. Treatment options for ischemic mitral regurgitation: A meta-analysis. J Thorac Cardiovasc Surg 2022;163:607-622.e14. [Crossref] [PubMed]
- Obadia JF, Messika-Zeitoun D, Leurent G, et al. Percutaneous Repair or Medical Treatment for Secondary Mitral Regurgitation. N Engl J Med 2018;379:2297-306. [Crossref] [PubMed]
- Stone GW, Abraham WT, Lindenfeld J, et al. Five-Year Follow-up after Transcatheter Repair of Secondary Mitral Regurgitation. N Engl J Med 2023;388:2037-48. [Crossref] [PubMed]
- Fiore A, Avtaar Singh SS, Nappi F. Learning from Controversy and Revisiting the Randomized Trials of Secondary Mitral Regurgitation. Rev Cardiovasc Med 2022;23:88. [Crossref] [PubMed]
- Nappi F, Avtaar Singh SS. Subannular repair or transcatheter edge-to-edge repair for secondary mitral regurgitation? More data for international guidelines. JTCVS Open 2022;10:176-80.
- Grayburn PA, Sannino A, Packer M. Proportionate and Disproportionate Functional Mitral Regurgitation: A New Conceptual Framework That Reconciles the Results of the MITRA-FR and COAPT Trials. JACC Cardiovasc Imaging 2019;12:353-62. [Crossref] [PubMed]
- McAlpine WA. Heart and coronary arteries: an anatomic atlas for clinical diagnosis, radiologic investigation and surgical treatment. Berlin, Germany: Springer-Verlag Publishers; 1975:15.
- Silbiger JJ. Does left atrial enlargement contribute to mitral leaflet tethering in patients with functional mitral regurgitation? Proposed role of atriogenic leaflet tethering. Echocardiography 2014;31:1310-1. [Crossref] [PubMed]
- Machino-Ohtsuka T, Seo Y, Ishizu T, et al. Novel Mechanistic Insights Into Atrial Functional Mitral Regurgitation - 3-Dimensional Echocardiographic Study. Circ J 2016;80:2240-8. [Crossref] [PubMed]
- Ito K, Abe Y, Takahashi Y, et al. Mechanism of atrial functional mitral regurgitation in patients with atrial fibrillation: A study using three-dimensional transesophageal echocardiography. J Cardiol 2017;70:584-90. [Crossref] [PubMed]
- Kim DH, Heo R, Handschumacher MD, et al. Mitral Valve Adaptation to Isolated Annular Dilation: Insights Into the Mechanism of Atrial Functional Mitral Regurgitation. JACC Cardiovasc Imaging 2019;12:665-77. [Crossref] [PubMed]
- Dal-Bianco JP, Aikawa E, Bischoff J, et al. Active adaptation of the tethered mitral valve: insights into a compensatory mechanism for functional mitral regurgitation. Circulation 2009;120:334-42. [Crossref] [PubMed]
- Nappi F, Nenna A, Mihos C, et al. Ischemic functional mitral regurgitation: from pathophysiological concepts to current treatment options. A systemic review for optimal strategy. Gen Thorac Cardiovasc Surg 2021;69:213-29. [Crossref] [PubMed]
- Nappi F, Spadaccio C. Biomechanics of failed ischemic mitral valve repair: Discovering new frontiers. J Thorac Cardiovasc Surg 2017;154:832-3. [Crossref] [PubMed]
- Van De Heyning CM, Claeys MJ. Annular Dynamics in Patients With Atrial Fibrillation and AFMR: The Next Piece of the Puzzle. JACC Cardiovasc Imaging 2022;15:14-6. [Crossref] [PubMed]
- Lang RM, Badano LP, Mor-Avi V, et al. Recommendations for cardiac chamber quantification by echocardiography in adults: an update from the American Society of Echocardiography and the European Association of Cardiovascular Imaging. Eur Heart J Cardiovasc Imaging 2015;16:233-70. [Crossref] [PubMed]
- Bertrand PB, Schwammenthal E, Levine RA, et al. Exercise Dynamics in Secondary Mitral Regurgitation: Pathophysiology and Therapeutic Implications. Circulation 2017;135:297-314. [Crossref] [PubMed]
- Kwan J, Shiota T, Agler DA, et al. Geometric differences of the mitral apparatus between ischemic and dilated cardiomyopathy with significant mitral regurgitation: real-time three-dimensional echocardiography study. Circulation 2003;107:1135-40. [Crossref] [PubMed]
- Grayburn PA, Thomas JD. Basic Principles of the Echocardiographic Evaluation of Mitral Regurgitation. JACC Cardiovasc Imaging 2021;14:843-53. [Crossref] [PubMed]
- Lancellotti P, Tribouilloy C, Hagendorff A, et al. Recommendations for the echocardiographic assessment of native valvular regurgitation: an executive summary from the European Association of Cardiovascular Imaging. Eur Heart J Cardiovasc Imaging 2013;14:611-44. [Crossref] [PubMed]
- Caldarera I, Van Herwerden LA, Taams MA, et al. Multiplane transoesophageal echocardiography and morphology of regurgitant mitral valves in surgical repair. Eur Heart J 1995;16:999-1006. [Crossref] [PubMed]
- Oliveira D, Srinivasan J, Espino D, et al. Geometric description for the anatomy of the mitral valve: A review. J Anat 2020;237:209-24. [Crossref] [PubMed]
- Ring L, Dutka DP, Boyd J, et al. The Normal Mitral Valve Annulus in Humans Defined Using 3-Dimensional Transesophageal Echocardiography. JACC Cardiovasc Imaging 2018;11:510-2. [Crossref] [PubMed]
- Sonne C, Sugeng L, Watanabe N, et al. Age and body surface area dependency of mitral valve and papillary apparatus parameters: assessment by real-time three-dimensional echocardiography. Eur J Echocardiogr 2009;10:287-94. [Crossref] [PubMed]
- Reddy YNV, Obokata M, Gersh BJ, et al. High Prevalence of Occult Heart Failure With Preserved Ejection Fraction Among Patients With Atrial Fibrillation and Dyspnea. Circulation 2018;137:534-5. [Crossref] [PubMed]
- Yap J, Bolling SF, Rogers JH. Contemporary review in interventional cardiology: mitral annu- loplasty in secondary mitral regurgitation. Struct Heart 2021;5:247-62.
- Nappi F, Carotenuto AR, Avtaar Singh SS, et al. Euler's Elastica-Based Biomechanics of the Papillary Muscle Approximation in Ischemic Mitral Valve Regurgitation: A Simple 2D Analytical Model. Materials (Basel) 2019;12:1518. [Crossref] [PubMed]
- Nappi F, Attias D, Avtaar Singh SS, et al. Finite element analysis applied to the transcatheter mitral valve therapy: Studying the present, imagining the future. J Thorac Cardiovasc Surg 2019;157:e149-51. [Crossref] [PubMed]
- Sacks MS, He Z, Baijens L, et al. Surface strains in the anterior leaflet of the functioning mitral valve. Ann Biomed Eng 2002;30:1281-90. [Crossref] [PubMed]
- Nappi F, Spadaccio C, Mihos CG, et al. Biomechanics raises solution to avoid geometric mitral valve configuration abnormalities in ischemic mitral regurgitation. J Thorac Dis 2017;9:S624-8. [Crossref] [PubMed]
- Prot V, Skallerud B, Sommer G, et al. On modelling and analysis of healthy and pathological human mitral valves: two case studies. J Mech Behav Biomed Mater 2010;3:167-77. [Crossref] [PubMed]
- Prot V, Skallerud B. Contributions of prestrains, hyperelasticity, and muscle fiber activation on mitral valve systolic performance. Int J Numer Method Biomed Eng 2017; [Crossref]
- Beaudoin J, Dal-Bianco JP, Aikawa E, et al. Mitral Leaflet Changes Following Myocardial Infarction: Clinical Evidence for Maladaptive Valvular Remodeling. Circ Cardiovasc Imaging 2017;10:e006512. [Crossref] [PubMed]
- Bischoff J, Casanovas G, Wylie-Sears J, et al. CD45 Expression in Mitral Valve Endothelial Cells After Myocardial Infarction. Circ Res 2016;119:1215-25. [Crossref] [PubMed]
- Bartko PE, Dal-Bianco JP, Guerrero JL, et al. Effect of Losartan on Mitral Valve Changes After Myocardial Infarction. J Am Coll Cardiol 2017;70:1232-44. [Crossref] [PubMed]
- Marsit O, Clavel MA, Côté-Laroche C, et al. Attenuated Mitral Leaflet Enlargement Contributes to Functional Mitral Regurgitation After Myocardial Infarction. J Am Coll Cardiol 2020;75:395-405. [Crossref] [PubMed]
- Nappi F, Spadaccio C, Fraldi M. Reply: Papillary Muscle Approximation Is an Anatomically Correct Repair for Ischemic Mitral Regurgitation. J Am Coll Cardiol 2016;68:1147-8. [Crossref] [PubMed]
- Nappi F, Spadaccio C. Obstructive Cardiomyopathy and Tethering in Ischemic Mitral Regurgitation: Two Sides of the Coin. Ann Thorac Surg 2019;107:1911-2. [Crossref] [PubMed]
- Bisbal F, Baranchuk A, Braunwald E, et al. Atrial Failure as a Clinical Entity: JACC Review Topic of the Week. J Am Coll Cardiol 2020;75:222-32. [Crossref] [PubMed]
- Patel RB, Shah SJ. Therapeutic Targeting of Left Atrial Myopathy in Atrial Fibrillation and Heart Failure With Preserved Ejection Fraction. JAMA Cardiol 2020;5:497-9. [Crossref] [PubMed]
- Reddy YNV, Obokata M, Verbrugge FH, et al. Atrial Dysfunction in Patients With Heart Failure With Preserved Ejection Fraction and Atrial Fibrillation. J Am Coll Cardiol 2020;76:1051-64. [Crossref] [PubMed]
- Packer M, Lam CSP, Lund LH, et al. Interdependence of Atrial Fibrillation and Heart Failure With a Preserved Ejection Fraction Reflects a Common Underlying Atrial and Ventricular Myopathy. Circulation 2020;141:4-6. [Crossref] [PubMed]
- Si J, Ding Z, Chen X, et al. Effects of Rhythm Control for Atrial Fibrillation on Cardiac Remodeling and Valvular Regurgitation in Patients with Heart Failure. Cardiovasc Drugs Ther 2023; Epub ahead of print. [Crossref]
- Tamargo M, Obokata M, Reddy YNV, et al. Functional mitral regurgitation and left atrial myopathy in heart failure with preserved ejection fraction. Eur J Heart Fail 2020;22:489-98. [Crossref] [PubMed]
- Kuppahally SS, Akoum N, Burgon NS, et al. Left atrial strain and strain rate in patients with paroxysmal and persistent atrial fibrillation: relationship to left atrial structural remodeling detected by delayed-enhancement MRI. Circ Cardiovasc Imaging 2010;3:231-9. [Crossref] [PubMed]
- Matta M, Ayoub C, Abou Hassan OK, et al. Anatomic and functional determinants of atrial functional mitral regurgitation. Structural Heart 2021;5:498-507.
- Krishnamurthy G, Ennis DB, Itoh A, et al. Material properties of the ovine mitral valve anterior leaflet in vivo from inverse finite element analysis. Am J Physiol Heart Circ Physiol 2008;295:H1141-9. [Crossref] [PubMed]
- He S, Fontaine AA, Schwammenthal E, et al. Integrated mechanism for functional mitral regurgitation: leaflet restriction versus coapting force: in vitro studies. Circulation 1997;96:1826-34. [Crossref] [PubMed]
- Kagiyama N, Mondillo S, Yoshida K, et al. Subtypes of Atrial Functional Mitral Regurgitation: Imaging Insights Into Their Mechanisms and Therapeutic Implications. JACC Cardiovasc Imaging 2020;13:820-35. [Crossref] [PubMed]
- Fraldi M, Spadaccio C, Mihos CG, et al. Analysing the reasons of failure of surgical mitral repair approaches-do we need to better think in biomechanics? J Thorac Dis 2017;9:S661-4. [Crossref] [PubMed]
- Nappi F, Nenna A, Chello M. Structural Heart Valve Disease in the Era of Change and Innovation: The Crosstalk between Medical Sciences and Engineering. Bioengineering (Basel) 2022;9:230. [Crossref] [PubMed]
- Schwammenthal E, Chen C, Benning F, et al. Dynamics of mitral regurgitant flow and orifice area. Physiologic application of the proximal flow convergence method: clinical data and experimental testing. Circulation 1994;90:307-22. [Crossref] [PubMed]
- Bartko PE, Heitzinger G, Spinka G, et al. Principal Morphomic and Functional Components of Secondary Mitral Regurgitation. JACC Cardiovasc Imaging 2021;14:2288-300. [Crossref] [PubMed]
- Bai W, Liu ZQ, He PY, et al. The role of IL-6, IL-10, TNF-α and PD-1 expression on CD4 T cells in atrial fibrillation. Heliyon 2023;9:e18818. [Crossref] [PubMed]
- Hu YF, Chen YJ, Lin YJ, et al. Inflammation and the pathogenesis of atrial fibrillation. Nat Rev Cardiol 2015;12:230-43. [Crossref] [PubMed]
- Kato T, Sekiguchi A, Sagara K, et al. Endothelial-mesenchymal transition in human atrial fibrillation. J Cardiol 2017;69:706-11. [Crossref] [PubMed]
- Dziadzko V, Clavel MA, Dziadzko M, et al. Outcome and undertreatment of mitral regurgitation: a community cohort study. Lancet 2018;391:960-9. [Crossref] [PubMed]
- Chioncel O, Lainscak M, Seferovic PM, et al. Epidemiology and one-year outcomes in patients with chronic heart failure and preserved, mid-range and reduced ejection fraction: an analysis of the ESC Heart Failure Long-Term Registry. Eur J Heart Fail 2017;19:1574-85. [Crossref] [PubMed]
- Kajimoto K, Sato N, Takano T, et al. Functional mitral regurgitation at discharge and outcomes in patients hospitalized for acute decompensated heart failure with a preserved or reduced ejection fraction. Eur J Heart Fail 2016;18:1051-9. [Crossref] [PubMed]
- Zoghbi WA, Adams D, Bonow RO, et al. Recommendations for Noninvasive Evaluation of Native Valvular Regurgitation: A Report from the American Society of Echocardiography Developed in Collaboration with the Society for Cardiovascular Magnetic Resonance. J Am Soc Echocardiogr 2017;30:303-71. [Crossref] [PubMed]
- Lee CS, Lin TH, Hsu PC, et al. Measuring left ventricular peak longitudinal systolic strain from a single beat in atrial fibrillation: validation of the index beat method. J Am Soc Echocardiogr 2012;25:945-52. [Crossref] [PubMed]
- Kusunose K, Yamada H, Nishio S, et al. Index-beat assessment of left ventricular systolic and diastolic function during atrial fibrillation using myocardial strain and strain rate. J Am Soc Echocardiogr 2012;25:953-9. [Crossref] [PubMed]
- Lin CP, Kuo CT, Wang CL, et al. Left ventricular systolic function is sensitive to cycle-length ir- regularity in patients with atrial fibrillation and systolic dysfunction. Acta Cardiol Sin 2012;28:103-10.
- Kerr AJ, Simmonds MB, Stewart RA. Influence of heart rate on stroke volume variability in atrial fibrillation in patients with normal and impaired left ventricular function. Am J Cardiol 1998;82:1496-500. [Crossref] [PubMed]
- Cawley PJ, Hamilton-Craig C, Owens DS, et al. Prospective comparison of valve regurgitation quantitation by cardiac magnetic resonance imaging and transthoracic echocardiography. Circ Cardiovasc Imaging 2013;6:48-57. [Crossref] [PubMed]
- Penicka M, Vecera J, Mirica DC, et al. Prognostic Implications of Magnetic Resonance-Derived Quantification in Asymptomatic Patients With Organic Mitral Regurgitation: Comparison With Doppler Echocardiography-Derived Integrative Approach. Circulation 2018;137:1349-60. [Crossref] [PubMed]
- Delgado V, Hundley WG. Added Value of Cardiovascular Magnetic Resonance in Primary Mitral Regurgitation. Circulation 2018;137:1361-3. [Crossref] [PubMed]
- Rottländer D, Gödde M, Degen H, et al. Procedural planning of CS-based indirect mitral annuloplasty using CT-angiography. Catheter Cardiovasc Interv 2021;98:1393-401. [Crossref] [PubMed]
- Rottländer D, Ballof J, Gödde M, et al. CT-Angiography to predict outcome after indirect mitral annuloplasty in patients with functional mitral regurgitation. Catheter Cardiovasc Interv 2021;97:495-502. [Crossref] [PubMed]
- Izumi C, Eishi K, Ashihara K, et al. JCS/JSCS/JATS/JSVS 2020 Guidelines on the Management of Valvular Heart Disease. Circ J 2020;84:2037-119. [Crossref] [PubMed]
- Deferm S, Bertrand PB, Verhaert D, et al. Outcome and durability of mitral valve annuloplasty in atrial secondary mitral regurgitation. Heart 2021;107:1503-9. [Crossref] [PubMed]
- Salsano A, Nenna A, Molinari N, et al. Impact of Mitral Regurgitation Recurrence on Mitral Valve Repair for Secondary Ischemic Mitral Regurgitation. J Cardiovasc Dev Dis 2023;10:124. [Crossref] [PubMed]
- Masuda M, Sekiya K, Asai M, et al. Influence of catheter ablation for atrial fibrillation on atrial and ventricular functional mitral regurgitation. ESC Heart Fail 2022;9:1901-13. [Crossref] [PubMed]
- Wu JT, Zaman JAB, Yakupoglu HY, et al. Catheter Ablation of Atrial Fibrillation in Patients With Functional Mitral Regurgitation and Left Ventricular Systolic Dysfunction. Front Cardiovasc Med. 2020;7:596491. [Crossref] [PubMed]
- Kawaji T, Shizuta S, Aizawa T, et al. Impact of catheter ablation for atrial fibrillation on cardiac disorders in patients with coexisting heart failure. ESC Heart Fail 2021;8:670-9. [Crossref] [PubMed]
- Saito C, Minami Y, Arai K, et al. Prevalence, clinical characteristics, and outcome of atrial functional mitral regurgitation in hospitalized heart failure patients with atrial fibrillation. J Cardiol 2018;72:292-9. [Crossref] [PubMed]
- Writing Committee Members. 2020 ACC/AHA Guideline for the Management of Patients With Valvular Heart Disease: A Report of the American College of Cardiology/American Heart Association Joint Committee on Clinical Practice Guidelines. J Am Coll Cardiol 2021;77:e25-197. Erratum in: J Am Coll Cardiol 2021;77:509; J Am Coll Cardiol 2021;77:1275; J Am Coll Cardiol 2023;82:969. [Crossref] [PubMed]
- Vahanian A, Beyersdorf F, Praz F, et al. 2021 ESC/EACTS Guidelines for the management of valvular heart disease. Eur Heart J 2022;43:561-632. [Crossref] [PubMed]
- Amabile A, Fereydooni S, Mori M, et al. Variable definitions and treatment approaches for atrial functional mitral regurgitation: A scoping review of the literature. J Card Surg 2022;37:1182-91. [Crossref] [PubMed]
- Shibata T, Takahashi Y, Fujii H, et al. Surgical considerations for atrial functional regurgitation of the mitral and tricuspid valves based on the etiological mechanism. Gen Thorac Cardiovasc Surg 2021;69:1041-9. [Crossref] [PubMed]
- Kainuma S, Taniguchi K, Daimon T, et al. Does stringent restrictive annuloplasty for functional mitral regurgitation cause functional mitral stenosis and pulmonary hypertension? Circulation 2011;124:S97-106. [Crossref] [PubMed]
- Kainuma S, Funatsu T, Kondoh H, et al. Beneficial effects of restrictive annuloplasty on subvalvular geometry in patients with functional mitral regurgitation and advanced cardiomyopathy. J Thorac Cardiovasc Surg 2018;156:630-638.e1. [Crossref] [PubMed]
- Nappi F, Spadaccio C, Chello M, et al. Double row of overlapping sutures for downsizing annuloplasty decreases the risk of residual regurgitation in ischaemic mitral valve repair. Eur J Cardiothorac Surg 2016;49:1182-7. [Crossref] [PubMed]
- Takahashi Y, Shibata T, Hattori K, et al. Extended posterior leaflet extension for mitral regurgitation in giant left atrium. J Heart Valve Dis 2014;23:88-90.
- Nappi F, Acar C. The Use of Anterior Mitral Leaflet Augmentation With Autologous Pericardium: Why Not? Ann Thorac Surg 2021;112:688-9. [Crossref] [PubMed]
- Olivito S, Lalande S, Nappi F, et al. Structural deterioration of the cryopreserved mitral homograft valve. J Thorac Cardiovasc Surg 2012;144:313-20, 320.e1.
- Nappi F. A Geometric Approach to Ischemic Mitral Regurgitation: Evaluating the Evidence of Valve Distortion. Ann Thorac Surg 2020;109:982. [Crossref] [PubMed]
- Nappi F, Gambardella I. Combined Replacement and Subvalvular Repair for Functional Mitral Regurgitation: The New Frontier? Ann Thorac Surg 2020;109:303-4. [Crossref] [PubMed]
- Nappi F, Lusini M, Spadaccio C, et al. Papillary Muscle Approximation Versus Restrictive Annuloplasty Alone for Severe Ischemic Mitral Regurgitation. J Am Coll Cardiol 2016;67:2334-46. [Crossref] [PubMed]
- Pausch J, Harmel E, Reichenspurner H, et al. Subannular repair in secondary mitral regurgitation with restricted leaflet motion during systole. Heart 2023;109:1394-400. [Crossref] [PubMed]
- Nappi F, Spadaccio C, Nenna A, et al. Is subvalvular repair worthwhile in severe ischemic mitral regurgitation? Subanalysis of the Papillary Muscle Approximation trial. J Thorac Cardiovasc Surg 2017;153:286-295.e2. [Crossref] [PubMed]
- Nappi F, Lusini M, Avtaar Singh SS, et al. Risk of Ischemic Mitral Regurgitation Recurrence After Combined Valvular and Subvalvular Repair. Ann Thorac Surg 2019;108:536-43. [Crossref] [PubMed]
- Nappi F, Nenna A, Spadaccio C, et al. Predictive factors of long-term results following valve repair in ischemic mitral valve prolapse. Int J Cardiol 2016;204:218-28. [Crossref] [PubMed]
- Yun KL, Sintek CF, Miller DC, et al. Randomized trial comparing partial versus complete chordal-sparing mitral valve replacement: effects on left ventricular volume and function. J Thorac Cardiovasc Surg 2002;123:707-14. [Crossref] [PubMed]
- Goldstein D, Moskowitz AJ, Gelijns AC, et al. Two-Year Outcomes of Surgical Treatment of Severe Ischemic Mitral Regurgitation. N Engl J Med 2016;374:344-53. [Crossref] [PubMed]
- Chen J, Wang Y, Lv M, et al. Mitral valve repair and surgical ablation for atrial functional mitral regurgitation. Ann Transl Med 2020;8:1420. [Crossref] [PubMed]
- Chew DS, Jones KA, Loring Z, et al. Diagnosis-to-ablation time predicts recurrent atrial fibrillation and rehospitalization following catheter ablation. Heart Rhythm O2 2021;3:23-31. [Crossref] [PubMed]
- Sunderland N, Maruthappu M, Nagendran M. What size of left atrium significantly impairs the success of maze surgery for atrial fibrillation? Interact Cardiovasc Thorac Surg 2011;13:332-8. [Crossref] [PubMed]
- Ortiz-Leon XA, Posada-Martinez EL, Trejo-Paredes MC, et al. Tricuspid and mitral remodelling in atrial fibrillation: a three-dimensional echocardiographic study. Eur Heart J Cardiovasc Imaging 2022;23:944-55. [Crossref] [PubMed]
- Ro SK, Kim JB, Jung SH, et al. Mild-to-moderate functional tricuspid regurgitation in patients undergoing mitral valve surgery. J Thorac Cardiovasc Surg 2013;146:1092-7. [Crossref] [PubMed]
- Gatti G, Dell'Angela L, Fiore A, et al. Basic pathophysiology and options of treatment for surgical management of functional tricuspid regurgitation: a systematic review. J Thorac Dis 2022;14:4521-44. [Crossref] [PubMed]
- Carino D, Lapenna E, Ascione G, et al. Is mitral annuloplasty an effective treatment for severe atrial functional mitral regurgitation? J Card Surg 2021;36:596-602. [Crossref] [PubMed]
- Takahashi Y, Abe Y, Sasaki Y, et al. Mitral valve repair for atrial functional mitral regurgitation in patients with chronic atrial fibrillation. Interact Cardiovasc Thorac Surg 2015;21:163-8. [Crossref] [PubMed]
- Kawamoto N, Fukushima S, Kainuma S, et al. Mitral valve surgery for atrial functional mitral regurgitation: predicting recurrent mitral regurgitation and mid-term outcome. Gen Thorac Cardiovasc Surg 2022;70:761-9. [Crossref] [PubMed]
- Balogh Z, Mizukami T, Bartunek J, et al. Mitral Valve Repair of Atrial Functional Mitral Regurgitation in Heart Failure with Preserved Ejection Fraction. J Clin Med 2020;9:3432. [Crossref] [PubMed]
- Kron IL, Green GR, Cope JT. Surgical relocation of the posterior papillary muscle in chronic ischemic mitral regurgitation. Ann Thorac Surg 2002;74:600-1. [Crossref] [PubMed]
- Hvass U, Joudinaud T. The papillary muscle sling for ischemic mitral regurgitation. J Thorac Cardiovasc Surg 2010;139:418-23. [Crossref] [PubMed]
- Padala M. Papillary Muscle Approximation Is an Anatomically Correct Repair for Ischemic Mitral Regurgitation. J Am Coll Cardiol 2016;68:1146-7. [Crossref] [PubMed]
- Wagner CM, Brescia AA, Watt TMF, et al. Surgical strategy and outcomes for atrial functional mitral regurgitation: All functional mitral regurgitation is not the same!. J Thorac Cardiovasc Surg 2024;167:647-55. [Crossref] [PubMed]
- Yoshida J, Ikenaga H, Nagaura T, et al. Impact of Percutaneous Edge-to-Edge Repair in Patients With Atrial Functional Mitral Regurgitation. Circ J 2021;85:1001-10. [Crossref] [PubMed]
- Benito-González T, Carrasco-Chinchilla F, Estévez-Loureiro R, et al. Clinical and echocardiographic outcomes of transcatheter mitral valve repair in atrial functional mitral regurgitation. Int J Cardiol 2021;345:29-35. [Crossref] [PubMed]
- Popolo Rubbio A, Testa L, Grasso C, et al. Transcatheter edge-to-edge mitral valve repair in atrial functional mitral regurgitation: insights from the multi-center MITRA-TUNE registry. Int J Cardiol 2022;349:39-45. [Crossref] [PubMed]
- Rottländer D, Golabkesh M, Degen H, et al. Mitral valve edge-to-edge repair versus indirect mitral valve annuloplasty in atrial functional mitral regurgitation. Catheter Cardiovasc Interv 2022;99:1839-47. [Crossref] [PubMed]
- Doldi P, Stolz L, Orban M, et al. Transcatheter Mitral Valve Repair in Patients With Atrial Functional Mitral Regurgitation. JACC Cardiovasc Imaging 2022;15:1843-51. [Crossref] [PubMed]
- Rogers JH, Boyd WD, Smith TW, et al. Transcatheter Mitral Valve Direct Annuloplasty with the Millipede IRIS Ring. Interv Cardiol Clin 2019;8:261-7. [Crossref] [PubMed]
- Miller M, Thourani VH, Whisenant B. The Cardioband transcatheter annular reduction system. Ann Cardiothorac Surg 2018;7:741-7. [Crossref] [PubMed]
- Kirchhof P, Camm AJ, Goette A, et al. Early Rhythm-Control Therapy in Patients with Atrial Fibrillation. N Engl J Med 2020;383:1305-16. [Crossref] [PubMed]
- Guta AC, Badano LP, Tomaselli M, et al. The Pathophysiological Link between Right Atrial Remodeling and Functional Tricuspid Regurgitation in Patients with Atrial Fibrillation: A Three-Dimensional Echocardiography Study. J Am Soc Echocardiogr 2021;34:585-594.e1. [Crossref] [PubMed]
- Abe Y, Akamatsu K, Ito K, et al. Prevalence and Prognostic Significance of Functional Mitral and Tricuspid Regurgitation Despite Preserved Left Ventricular Ejection Fraction in Atrial Fibrillation Patients. Circ J 2018;82:1451-8. [Crossref] [PubMed]
- Fukuda S, Saracino G, Matsumura Y, et al. Three-dimensional geometry of the tricuspid annulus in healthy subjects and in patients with functional tricuspid regurgitation: a real-time, 3-dimensional echocardiographic study. Circulation 2006;114:I492-8. [Crossref] [PubMed]
- Utsunomiya H, Harada Y, Susawa H, et al. Tricuspid valve geometry and right heart remodelling: insights into the mechanism of atrial functional tricuspid regurgitation. Eur Heart J Cardiovasc Imaging 2020;21:1068-78. [Crossref] [PubMed]
- Utsunomiya H, Itabashi Y, Mihara H, et al. Functional Tricuspid Regurgitation Caused by Chronic Atrial Fibrillation: A Real-Time 3-Dimensional Transesophageal Echocardiography Study. Circ Cardiovasc Imaging 2017;10:e004897. [Crossref] [PubMed]
- Topilsky Y, Khanna A, Le Tourneau T, et al. Clinical context and mechanism of functional tricuspid regurgitation in patients with and without pulmonary hypertension. Circ Cardiovasc Imaging 2012;5:314-23. [Crossref] [PubMed]
- Florescu DR, Muraru D, Florescu C, et al. Right heart chambers geometry and function in patients with the atrial and the ventricular phenotypes of functional tricuspid regurgitation. Eur Heart J Cardiovasc Imaging 2022;23:930-40. [Crossref] [PubMed]
- Silbiger JJ. Atrial functional tricuspid regurgitation: An underappreciated cause of secondary tricuspid regurgitation. Echocardiography 2019;36:954-7. [Crossref] [PubMed]
- Nath J, Foster E, Heidenreich PA. Impact of tricuspid regurgitation on long-term survival. J Am Coll Cardiol 2004;43:405-9. [Crossref] [PubMed]
- Delling FN, Hassan ZK, Piatkowski G, et al. Tricuspid Regurgitation and Mortality in Patients With Transvenous Permanent Pacemaker Leads. Am J Cardiol 2016;117:988-92. [Crossref] [PubMed]
- Messika-Zeitoun D, Thomson H, Bellamy M, et al. Medical and surgical outcome of tricuspid regurgitation caused by flail leaflets. J Thorac Cardiovasc Surg 2004;128:296-302. [Crossref] [PubMed]
- Zack CJ, Fender EA, Chandrashekar P, et al. National Trends and Outcomes in Isolated Tricuspid Valve Surgery. J Am Coll Cardiol 2017;70:2953-60. [Crossref] [PubMed]
- Fender EA, Zack CJ, Nishimura RA. Isolated tricuspid regurgitation: outcomes and therapeutic interventions. Heart 2018;104:798-806. [Crossref] [PubMed]
- Dreyfus GD, Martin RP, Chan KM, et al. Functional tricuspid regurgitation: a need to revise our understanding. J Am Coll Cardiol 2015;65:2331-6. [Crossref] [PubMed]