Exploring the impact of variable power outputs on the efficacy and safety during microwave ablation for lung carcinoma: a real-world study
Highlight box
Key findings
• Although high-power microwave ablation (MWA) improves long-term prognosis for patients, it also results in an increase in postoperative complications. Utilizing a nomogram for stratifying patient prognosis may offer a more viable approach to developing individualized treatment plans.
What is known and what is new?
• MWA is a crucial technique for treating lung cancer, yet standardized guidelines for selecting the appropriate power are still lacking. This study investigated the effectiveness and safety of MWA across various power levels.
What is the implication, and what should change now?
• In the treatment of lung carcinoma, high-power MWA demonstrates greater efficacy compared to low-power MWA but is associated with a higher incidence of complications such as pneumothorax and hemorrhage. Implementing risk stratification based on our nomogram and developing a standardized treatment protocol for power selection will enhance the efficacy and safety of MWA.
Introduction
Lung cancer incidence and mortality rates are increasing both in China and overseas, which is primarily due to environmental pollution and the growing number of smokers. Despite recent advancements in treatment, lung cancer remains the leading cause of cancer-related deaths with one of the lowest 5-year survival rates among all cancer types. In women, it ranks as the third most common cancer type and the second leading cause of cancer-related deaths and adenocarcinoma is the predominant subtype of non-small cell lung cancer (NSCLC) (1). The increasing global burden of lung cancer and the limitations of current treatment strategies underscore the need for alternative therapies (2,3). In recent years, with the widespread adoption of low-dose lung computed tomography (CT) scans, pulmonary nodules (ground-glass opacity/ground-glass nodule, GGO/GGN), particularly mixed high-risk nodules with a significant potential for malignancy, have become more prevalently found especially in younger patients. This is especially true for those who have undergone comprehensive assessments, including positron emission tomography-CT (PET-CT) scans, that have not identified any metastatic disease. Consequently, these individuals often choose short-term follow-up observation (3). However, even for GGNs that remained stable over a 5-year period, nodule growth was found in 13.0% of patients during a 10-year follow-up (4,5). Simultaneously, there has been a significant shift in the treatment approach for certain patient populations which includes older individuals with multiple comorbidities and compromised cardiopulmonary function, who are not suitable candidates for surgery, patients with local therapies such as conformal radiotherapy, experience recurrence in the form of isolated lesions, similarly, individuals with a history of surgery for tumors at other anatomical sites, now presenting with either primary or metastatic pulmonary lesions, often express reluctance towards undergoing further surgical interventions (6). Thanks to the emergence of next-generation medical imaging techniques such as CT and electromagnetic navigation technology, image-guided thermal ablation treatment such as radiofrequency ablation (RFA), microwave ablation (MWA), and cryoablation has been gradually applied to the treatment of lung cancer, among which, MWA holds theoretical advantages over RFA, as it can generate a larger ablation zone in a shorter duration, which represents to be an important local radical or palliative treatment for lung cancer patients who are unable or unwilling to operate (7).
However, there is currently limited research comparing the prognosis of lung cancer patients undergoing MWA versus surgical intervention, and the conclusions drawn from existing studies are often conflicting (7-10). Moreover, many of these studies have relatively short follow-up periods, primarily consisting of retrospective analyses, and are predominantly focused on early-stage lung adenocarcinoma in stage I, offering limited guidance for different histological subtypes of lung cancer (11). On the other hand, regarding the treatment approach with MWA, particularly in terms of microwave power and ablation duration, there is a lack of standardized criteria. In most medical centers, decisions regarding power and treatment duration are often based on the subjective judgment and experience of surgeons, leading to random variations in treatment parameters, which significantly impact local recurrence rates and long-term prognoses for patients. Furthermore, GGN is typically found near pulmonary vessels or may have inter-nodular vessels, especially in cases of multiple nodules. MWA may result in relatively higher complications, including bleeding and pneumothorax, when power settings are determined solely by the surgeon’s subjective experience.
This real-world study reviewed the clinicopathological data of patients in recent 8 years who received MWA and novel power-based nomograms were developed to explore the relationship between MWA duration, power, and prognostic risk factors, in order to evaluate the safety and efficacy of various power standards in MWA for lung cancer, with the expectation of laying a theoretical foundation for the establishment of standardized diagnostic and therapeutic models in the future. We present this article in accordance with the TRIPOD reporting checklist (available at https://jtd.amegroups.com/article/view/10.21037/jtd-24-557/rc).
Methods
Study population
This study included patients who received MWA treatment for lung carcinoma at our hospital from January 2012 to December 2020. Patient inclusion criteria were defined as follows: (I) age greater than 18 years; (II) confirmed NSCLC based on pathological results; (III) absence of metastasis; (IV) presence of interstitial lung diseases, pulmonary fibrosis, or severe emphysema [30%≤ forced expiratory volume in the first second (FEV1) <50%, with more than two acute exacerbations of pulmonary symptoms in the past year, or at least one hospitalization for these symptoms (Group C); or severe ventilation limitation, with at least two acute exacerbations of pulmonary symptoms in the past year, or at least one hospitalization for these symptoms (Group D)] as underlying pulmonary conditions; (V) Eastern Cooperative Oncology Group (ECOG) performance status score of 0–1; (VI) no history of radiation therapy; (VII) no prior neoadjuvant therapy before surgery; (VIII) absence of the following surgical history: ipsilateral thoracotomy, ipsilateral thoracoscopic lung or esophageal resection, mediastinal surgery (excluding procedures for lung bullae and rib fractures), contralateral thoracotomy or thoracoscopic surgery (excluding procedures for lung bullae and rib fractures); (IX) documentation of informed consent from both patients and their families concerning the surgical procedure and the research protocol.
Exclusion criteria for this study included: (I) pregnancy or lactation; (II) co-occurring mental health disorders; (III) simultaneous presence of active bacterial or fungal infections; (IV) presence of interstitial lung diseases, pulmonary fibrosis, or severe emphysema as underlying pulmonary conditions; (V) considered unsuitable for inclusion following evaluation by the research team; (VI) history of angina pectoris, myocardial infarction, or heart failure within the preceding 6 months; (VII) the patient was ultimately unable to complete the follow-up due to reasons such as population mobility and changes in phone numbers. The study was conducted in accordance with the Declaration of Helsinki (as revised in 2013) and was approved by the Ethics Committee of Beijing Chaoyang Hospital (2023-D.-471). Since this is a retrospective study, the requirement for informed consent was waived.
Data collection and follow-up
The data were gathered through an electronic medical record system, encompassing patient baseline details, laboratory test outcomes, surgery-associated information (including MWA power, surgery duration, intraoperative blood loss, etc.), postoperative complications (such as pneumothorax, hemothorax, and pulmonary hemorrhage), and pathological results (pathological type, degree of differentiation, gene mutations, etc.).
We ensured ongoing patient follow-up via phone calls, outpatient consultations, or in-hospital monitoring until December 2022 or in the event of a patient’s death. For discharged patients, follow-up assessments were conducted at 6 months, 1 year, and 2 years after the surgical procedure to evaluate both short-term and long-term complications, along with their survival status. The overall survival (OS) was characterized as the duration from the initial procedure to either the patient’s demise or the latest observation, while disease-free survival (DFS) was described as the interval from the initial procedure to any recurrence.
MWA procedure
Each MWA procedure utilizes the MTC-3C microwave ablation system (Zhongyuan Medical Device Research Center), incorporating CT and magnetic navigation systems for precise MWA guidance. Meanwhile, we use a thermographic imaging system to monitor and evaluate the effects of the MWA and the work range of the lungs (Figure S1). We used a frequency of 2,450±50 MHz, and this study categorizes the high power group as having an output power of 60 W for 8 min, whereas the low power group is classified as having an output power of 40 W for 8 min, meanwhile, for the microwave antenna, the effective length was 180 mm and the dominant outside diameter was 18 G. A water circulation cooling system was employed to cool the surface temperature of the antennae. To ensure the quality of the MWA, all operations are performed by chief physicians with rich experience in tumor ablation. The radiographic evidence of a successful surgery is indicated by the lesions post-ablation being 5–10 mm larger than the targeted lesion.
Statistical analysis
Group comparisons for continuous variables were conducted using either the Student’s t-test or the Mann-Whitney U test. For categorical variables, group assessments were performed using either Pearson’s Chi-squared test or Fisher’s exact test. Kaplan-Meier curves alongside the log-rank test were utilized for the purpose of survival analysis. In univariate analysis, if P<0.2, then it will be included in multivariate analysis. The Cox regression model is used to identify independent prognostic factors associated with OS. Based on the independent risk factors, create a nomogram to predict the long-term prognosis of MWA patients. The criterion for statistical significance was defined as a two-sided P<0.05. SPSS (version 26.0), GraphPad Prism (version 8.0) and R software (version 4.3.1) were utilized for all statistical analyses.
Results
Patient’s basic information
This study included a total of 265 patients with NSCLC who underwent MWA, including 192 individuals in the low power group and 73 individuals in the high power group. Compared to the low power group, the high power group had a lower smoking rate (16.4% vs. 19.8%, P<0.001), larger tumor volume (44.6 vs. 11.5 cm3, P=0.04), higher tumor differentiation (P<0.001), more complications (24.0% vs. 63.0%, P<0.001), and a significantly prolonged length of hospital stay (5.1±4.3 vs. 3.1±2.1, P<0.001) (Table 1). Between the two groups, there were no statistically significant differences in terms of age, comorbidities, genetic mutations, and counts of peripheral blood cell subgroups (such as neutrophils, monocytes, lymphocytes, and platelets).
Table 1
Variables | Total (n=265) | Low power group (n=192) | High power group (n=73) | P |
---|---|---|---|---|
Gender (male) | 177 (66.8) | 134 (69.8) | 43 (58.9) | <0.001 |
Age (years) | 69.1±9.7 | 69.0±9.1 | 69.2±11.1 | 0.91 |
Smoking | 50 (18.9) | 38 (19.8) | 12 (16.4) | <0.001 |
Comorbidity | ||||
Diabetes | 35 (13.2) | 24 (12.5) | 11 (15.1) | 0.58 |
Hypertension | 68 (25.7) | 52 (27.1) | 16 (21.9) | 0.39 |
Coronary heart disease | 15 (5.7) | 9 (4.7) | 6 (8.2) | 0.27 |
Tumor volume (cm3) | 17.1±66.3 | 11.5±51.9 | 44.6±74.8 | 0.04 |
Tumor differentiation | <0.001 | |||
Poor | 33 (12.5) | 26 (13.5) | 7 (9.6) | |
Moderate and high | 232 (87.5) | 166 (86.5) | 66 (90.4) | |
Gene mutation | 66 (24.9) | 46 (24.0) | 20 (27.4) | 0.56 |
Complication | 92 (34.7) | 46 (24.0) | 46 (63.0) | <0.001 |
Neutrophil (×109/L) | 4.0±1.9 | 4.0±1.9 | 4.0±1.8 | 0.86 |
Monocyte (×109/L) | 0.5±0.2 | 0.5±0.2 | 0.5±0.2 | 0.77 |
Lymphocyte (×109/L) | 1.7±0.6 | 1.7±0.6 | 1.7±0.6 | 0.96 |
Platelet (×109/L) | 207.3±58.8 | 204.5±57.1 | 214.8±62.5 | 0.20 |
Length of hospital stay | 3.7±3.0 | 3.1±2.1 | 5.1±4.3 | <0.001 |
Data are presented as n (%) and mean ± standard deviation.
Safety and effectiveness of MWA at different power levels
Pneumothorax, hemothorax, and pulmonary hemorrhage are the most common complications after MWA. We collected relevant data to assess the safety of surgeries between the low power group and the high power group. It is obvious that the incidence of pneumothorax (39.7% vs. 16.1%, P<0.001), hemothorax (6.8% vs. 1.6%, P=0.04), and pulmonary hemorrhage (21.9% vs. 10.4%, P=0.03) in the high power group were significantly higher than those in the low power group (Table 2).
Table 2
Complications | Total (n=265) | Low power group (n=192) | High power group (n=73) | P value |
---|---|---|---|---|
Pneumothorax | 60 (22.6%) | 31 (16.1%) | 29 (39.7%) | <0.001 |
Hemothorax | 8 (3.0%) | 3 (1.6%) | 5 (6.8%) | 0.04 |
Pulmonary hemorrhage | 36 (13.6%) | 20 (10.4%) | 16 (21.9%) | 0.03 |
The median follow-up time for this study was 43.8 months. We compared the long-term prognosis of patients in the low power group and the high power group using Kaplan-Meier survival analysis. Compared to the low power group, the OS rate of the high power group was significantly higher (log-rank test, P=0.04) (Figure 1A). As for the DFS rate, the high power group was also higher than the low power group, but there was no statistical difference (log-rank test, P=0.08) (Figure 1B).
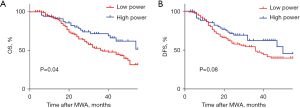
Constructing a nomogram for evaluating the long-term prognosis of patients
In this study, we observed that high-power MWA improved patient survival rates, but it was also accompanied by an increase in complications. Therefore, screening out patients with poorer prognosis and applying high power MWA may effectively improve long-term prognosis. For patients with a better prognosis, the application of low power MWA can effectively reduce the occurrence of complications without affecting long-term survival. Thus, we attempted to establish a nomogram for predicting long-term prognosis in patients, aiming to assist clinicians in devising personalized treatment plans.
Firstly, we applied Cox regression analysis to screen for independent relevant factors that affect the long-term prognosis of patients undergoing MWA. The results indicated that smoking, tumor volume, tumor differentiation, gene mutation, neutrophil count, and lymphocyte count were independent factors influencing the OS of patients (P<0.001) (Table 3). Subsequently, based on the above factors, we constructed a nomogram to assess the long-term prognosis of such patients (Figure 2). The nomograms assign a particular score to each factor, after which the cumulative overall risk score is computed using these scores. Later, drawing a vertical line allows for an intuitive estimation of the long-term outlook for each patient following MWA. Finally, the receiver operating characteristic (ROC) curve was used to evaluate the predictive value of the nomogram for the OS of NSCLC patients undergoing MWA. The results showed that the areas under the curve (AUCs) corresponding to 1, 2, and 3 years were 0.941, 0.903, and 0.905, respectively (Figure 3).
Table 3
Variables | Univariable Cox regression | Multivariable Cox regression | |||
---|---|---|---|---|---|
HR (95% CI) | P | HR (95% CI) | P | ||
Gender (male) | 0.689 (0.361–1.313) | 0.69 | |||
Age | 0.998 (0.976–1.021) | 0.88 | |||
Smoking | 1.834 (1.120–3.002) | <0.001 | 2.945 (1.858–4.667) | <0.001 | |
Tumor volume | 1.003 (1.002–1.004) | <0.001 | 1.003 (1.002–1.004) | <0.001 | |
Tumor differentiation (Low) | 6.453 (3.974–10.477) | <0.001 | 4.285 (2.553–7.191) | <0.001 | |
Gene mutation | 0.170 (0.077–0.372) | <0.001 | 0.208 (0.094–0.463) | <0.001 | |
Complication | 1.618 (1.062–2.466) | 0.03 | 1.156 (0.756–1.766) | 0.50 | |
Neutrophil | 1.315 (1.145–1.510) | <0.001 | 1.287 (1.157–1.432) | <0.001 | |
Monocyte | 1.181 (0.273–5.104) | 0.82 | |||
Lymphocyte | 0.590 (0.410–0.849) | 0.004 | 0.447 (0.308–0.650) | <0.001 | |
Platelet | 1.001 (0.998–1.004) | 0.55 |
HR, hazard ratio; CI, confidence interval.
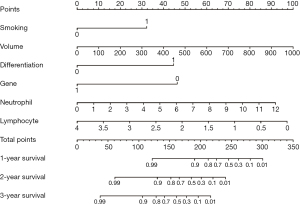
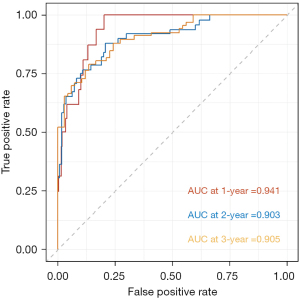
Discussion
This is the largest single center real world study conducted to date to explore the efficacy and safety of MWA in lung cancer patients under different standard of power and to analyze the risk factors affecting the long-term prognosis following MWA. A total of 265 patients were retrospectively enrolled. Treatment efficacy was comparable between two groups. The incidence of complications such as pneumothorax and intrapulmonary hemorrhage was lower in patients treated with the standard low power groups than in those treated with standard high power.
MWA represents to be a minimally invasive treatment that rapidly deactivates lung tumor cells in situ, while causing minimal damage to the surrounding normal tissue and reversible (12). Its principle involves the application of high-frequency electromagnetic waves ranging from 915 to 2,450 MHz to the target tumor. This induces rapid rotation of polar water molecules, converting kinetic energy into heat. When tissue temperature rises to 60 ℃ or higher, it leads to complete coagulative necrosis of tissue cells, thus deactivating the target tissue (13). Furukawa et al. reported immediate changes in the tissue surrounding the electrodes following MWA, including fibrosis and collagen fiber thickening. Subsequently, the ablated pulmonary tissues were gradually replaced by fibrous scar tissues over a period of 6 months (14). Meanwhile, the clinical safety of MWA has been demonstrated in several clinical studies. Wolf et al. reported on 50 lung cancer patients treated with MWA, with a median survival time of 19 months following treatment. The first recorded death occurred 256 days after MWA, attributed to fatal massive hemoptysis. The median survival time for those who succumbed to cancer-related causes was 22 months, with survival rates at 1, 2, and 3 years of 83%, 73%, and 61%, respectively (15). Nevertheless, more data, especially in large patient group, are still needed for evaluating the safety and effectiveness of MWA in treating pulmonary malignancies.
In the course of MWA therapy, multiple factors can affect coagulation patterns and extent, with microwave power and ablation duration significantly influencing the range of ablation. The majority of pulmonary lesion ablations are presently conducted under local anesthesia, and managing pain arising from MWA is of paramount concern. The primary source of pain is the thermal stimulation of the pleura. Throughout the treatment, surgeons customarily modulate microwave power and duration in response to the patient’s pain threshold to avoid excessive pleural injury that could result in unbearable pain and disruption of the ablation procedure. In general, power levels below 45 W are linked to manageable discomfort, but when dealing with lesions near the pleura requiring power levels surpassing 50 W, meticulous needle positioning and the application of local anesthesia are necessary (16).
Hence, many surgeons prefer using lower MWA power settings, prioritizing the safety, tolerance, and comfort of their patients during surgery. However, achieving a specific ablation range often requires extending the duration of the ablation procedure. While low-power ablation with extended duration enhances safety, it may affect local ablation precision. Factors such as thermal sink effects and heat dispersion due to blood flow can limit the ablation range, particularly when dealing with larger, partially solid lesions, especially those in close proximity to blood vessels. This aligns with the findings of our real-world study, which suggests that for patients with high-risk factors, although low-power ablation is associated with a reduced incidence of complications, it carries the risk of incomplete tumor inactivation and is therefore not recommended (17). High-power, short-duration MWA can provide an ample ablative energy supply, presenting a minimally invasive and swiftly effective treatment approach. High power represents the optimal approach to counteract heat dissipation from intravascular blood flow as well, resulting in the expansion of the ablation zone and the effective elimination of tumor lesions. After ablation, blood vessels may experience intraluminal thrombosis, with their walls potentially undergoing necrosis and structural collapse. Nevertheless, excessive heat can concomitantly harm the collagenous tissues of the lung, giving rise to complications such as bronchopleural fistula, cavity formation, pulmonary abscesses, and lung infections. Moreover, reports in the literature suggest that despite high-power ablation’s ability to counteract heat dissipation caused by blood flow, the size of the ablative zone markedly diminishes within regions located within 3 millimeters of larger airways, hinting at a pronounced cooling effect attributed to air circulation (18). Excessive power could potentially lead to the carbonization of tumor tissues adjacent to the electrode, impeding the conduction of heat to the surrounding region, ultimately resulting in a diminished ablation zone (19-21). Our study revealed that patients in the high-power group exhibited superior long-term recurrence and survival outcomes compared to those in the low-power group. Nevertheless, in the assessment of complications, the high-power group demonstrated a markedly elevated incidence compared to the low-power group. Consequently, for patients classified as relatively low risk according to our novel nomogram results, the low-power, extended-duration MWA approach represents a more favorable choice for enhancing prognosis.
In our group, all patients survived within 30 days, and complications occurred in 92 patients. The most common complication after MWA was pneumothorax and the incidence rate of pneumothorax was about 15% to 45% (21,22), particularly among the elderly population with concurrent chronic obstructive pulmonary disease (COPD), pneumothorax is more likely to manifest due to diminished lung elasticity and structural alterations. Should lung collapse exceed 30% or if concomitant symptoms of asthma are present, immediate interventions such as thoracic puncture for air evacuation or closed chest drainage should be initiated. To mitigate the risk of pneumothorax, several key considerations should be taken into account: firstly, administer preoperative oral cough suppressants and temporarily halt the ablation procedure if the patient experiences coughing during surgery; secondly, when selecting the puncture route, make an effort to avoid regions with lung bullae, bullous emphysema, or fissures; thirdly, stratify patients according to our nomogram outcomes and apply the appropriate ablation power to prevent excessive ablation, which may lead to the formation of cavities or pneumothorax; finally, during needle removal, gently rotate it in place to minimize the risk of adhesions pulling on lung tissue and inducing pneumothorax. Within our study cohort, 37 patients encountered instances of bleeding, all of which promptly ceased post-ablation. Intraoperative bleeding predominantly consisted of minor intrapulmonary bleeding or minimal hemoptysis. The ablation process inherently encourages blood clotting, resulting in an exceedingly low occurrence of significant hemoptysis. Nonetheless, both domestic and international literature have documented cases of MWA contributing to perioperative mortality due to excessive bleeding. To mitigate pulmonary bleeding, it is imperative to select the appropriate MWA power based on our risk factor stratification. In the case of high-risk patients with high-power settings, a shorter puncture path should be favored, and preoperative three-dimensional reconstructions should be conducted meticulously to accurately delineate the location of blood vessels, thereby enabling precise placement of the ablation needle while avoiding them. Other complications such as needle tract tumor implantation, bronchopleural fistula and air embolism did not occur in this real-world study cohort.
The degree of tumor differentiation serves as a pivotal factor in assessing malignancy. Poorly differentiated tumors display increased cellular heterogeneity, significant deviations from normal tissue, and a greater likelihood of localized tumor necrosis. Zuo et al. (23) developed a model based on clinical data from stage IB NSCLC patients in the Surveillance, Epidemiology and End Results (SEER) database, revealing histologically low differentiation as an independent prognostic factor. Our research likewise emphasizes that patients with lower differentiation experience markedly reduced OS compared to their better-differentiated counterparts. Tumor volume, additionally, stands out as a pivotal factor impacting the prognosis of patients undergoing MWA. In clinical practice, the primary tumor’s maximum diameter assumes a critical role in the tumor-node-metastasis (TNM) staging of tumors, with numerous studies corroborating its status as an independent prognostic factor for lung cancer (24,25). Additionally, greater tumor diameters exhibit a strong association with notably elevated recurrence rates following MWA. Nevertheless, prior investigations have predominantly relied on maximum tumor diameter as a proxy for tumor volume (26). In our research, we leveraged three-dimensional reconstruction systems and patient CT imaging data to meticulously reconstruct tumor volumes, yielding a more accurate representation. We posit that within this predictive model, heightened aggressiveness in larger tumors and incomplete ablation due to excessive volume are both linked to diminished postoperative survival rates among patients. The involvement of immune-inflammatory responses and associated cells in tumor progression has progressively emerged, encompassing markers like the neutrophil-to-lymphocyte ratio (NLR) and the platelet-to-lymphocyte ratio (PLR), which hold prognostic significance across diverse malignancies, representative neutrophils secrete inflammatory mediators, fostering tumor proliferation, metastasis, and augmenting tumor cell adhesion at remote sites, moreover, their release of growth factors and chemokines stimulates tumor angiogenesis. Lymphocyte count is intricately linked to acquired immunity, and its decline facilitates tumor immune evasion, thus serving as a crucial component in the tumor defense system (27,28). Our findings validate this perspective, as heightened neutrophils in lung cancer patients following MWA exhibit a substantial association with an unfavorable prognosis while lymphocytes represent protective factors. This phenomenon may, in part, be ascribed to local inflammatory responses induced by the ablation procedure, and multiple studies propose that MWA exerts an immune-enhancing impact on the body’s cellular immunity against tumors (29).
In recent years, with the deepening of research into genetic mutations in lung cancer, genetic testing of patients has gained significant importance in guiding treatment decisions and predicting prognosis. Common mutations in lung cancer include EGFR, KRAS, EML4-ALK, Ros1, and c-MET, among others. Personalized targeted therapies directed at specific mutation sites have emerged as a primary treatment approach for patients with identified gene mutations. The ADJUVANT study, which initially confirmed the benefits of EGFR-TKI in postoperative NSCLC patients with EGFR-positive mutations, significantly extending both DFS and OS compared to traditional chemotherapy, has been substantiated by numerous subsequent clinical studies (30). Our findings underscore that patients with identified gene mutations after MWA treatment experience significantly improved prognoses compared to those without such mutations, with EGFR mutation-positive patients showing superior outcomes in comparison to KRAS mutation-positive patients. The reasons for this observation may include subsequent targeted drug therapy tailored to patients with gene mutations. Furthermore, adjunctive targeted therapy may not be the exclusive factor contributing to the extended prognoses of mutation-positive patients. Cellular experiments have indicated that the thermal effect elevates the level of the T790M mutation in cells, thereby prolonging the duration of medication efficacy for EGFR-TKI-resistant patients. Xu and collaborators observed significant benefits for patients with limited TKI resistance following additional local ablation therapy (31). Therefore, it is evident that MWA treatment may extend the prognoses of patients with gene mutations by augmenting the effectiveness of targeted therapies.
This study had several limitations. First of all, this was a retrospective real world study rather than a prospective study. Secondly, different baseline characteristics between the groups may have influenced efficacy and safety, which needs to be further confirmed in prospective studies with large samples from multiple centers to reduce the bias caused by the data.
Conclusions
In conclusion, in the treatment of lung carcinoma, MWA performed with high power represents better efficacy compared with the patients in the low power group, but was associated with more complications of pneumothorax and hemorrhage. Risk stratification of patients based on our nomogram and further standardized treatment mode for power selection will better improve the efficacy and safety of MWA.
Acknowledgments
Funding: None.
Footnote
Reporting Checklist: The authors have completed the TRIPOD reporting checklist. Available at https://jtd.amegroups.com/article/view/10.21037/jtd-24-557/rc
Data Sharing Statement: Available at https://jtd.amegroups.com/article/view/10.21037/jtd-24-557/dss
Peer Review File: Available at https://jtd.amegroups.com/article/view/10.21037/jtd-24-557/prf
Conflicts of Interest: All authors have completed the ICMJE uniform disclosure form (available at https://jtd.amegroups.com/article/view/10.21037/jtd-24-557/coif). The authors have no conflicts of interest to declare.
Ethical Statement:
Open Access Statement: This is an Open Access article distributed in accordance with the Creative Commons Attribution-NonCommercial-NoDerivs 4.0 International License (CC BY-NC-ND 4.0), which permits the non-commercial replication and distribution of the article with the strict proviso that no changes or edits are made and the original work is properly cited (including links to both the formal publication through the relevant DOI and the license). See: https://creativecommons.org/licenses/by-nc-nd/4.0/.
References
- Bade BC, Dela Cruz CS. Lung Cancer 2020: Epidemiology, Etiology, and Prevention. Clin Chest Med 2020;41:1-24. [Crossref] [PubMed]
- Wang J, Hu B, Li T, et al. The EGFR-rearranged adenocarcinoma is associated with a high rate of venous thromboembolism. Ann Transl Med 2019;7:724. [Crossref] [PubMed]
- Mal R, Domini J, Wadhwa V, et al. Thermal ablation for primary and metastatic lung tumors: Single-center analysis of peri-procedural and intermediate-term clinical outcomes. Clin Imaging 2023;98:11-5. [Crossref] [PubMed]
- Lee HW, Jin KN, Lee JK, et al. Long-Term Follow-Up of Ground-Glass Nodules After 5 Years of Stability. J Thorac Oncol 2019;14:1370-7. [Crossref] [PubMed]
- Chen Q, Ji Y, Wang J, et al. Risk factor analysis of postoperative cerebral infarction in thoracic surgery patients by propensity-score matching-a single institution retrospective cohort study. J Thorac Dis 2023;15:620-6. [Crossref] [PubMed]
- Chen Q, Chen S, Wang J, et al. Construction and validation of a hypoxia-related risk signature identified EXO1 as a prognostic biomarker based on 12 genes in lung adenocarcinoma. Aging (Albany NY) 2023;15:2293-307. [Crossref] [PubMed]
- Zhao Q, Wang J, Fu YL, et al. Radiofrequency ablation for stage <IIB non-small cell lung cancer: Opportunities, challenges, and the road ahead. Thorac Cancer 2023;14:3181-90. [Crossref] [PubMed]
- Bartlett EC, Rahman S, Ridge CA. Percutaneous image-guided thermal ablation of lung cancer: What is the evidence? Lung Cancer 2023;176:14-23. [Crossref] [PubMed]
- Smith S, Jennings P. Thoracic intervention and surgery to cure lung cancer: image-guided thermal ablation in primary lung cancer. J R Soc Med 2019;112:218-25. [Crossref] [PubMed]
- Yao W, Lu M, Fan W, et al. Comparison between microwave ablation and lobectomy for stage I non-small cell lung cancer: a propensity score analysis. Int J Hyperthermia 2018;34:1329-36. [Crossref] [PubMed]
- Yang X, Ye X, Zheng A, et al. Percutaneous microwave ablation of stage I medically inoperable non-small cell lung cancer: clinical evaluation of 47 cases. J Surg Oncol 2014;110:758-63. [Crossref] [PubMed]
- Wang J, Cui SP, Zhao Q, et al. Preoperative systemic immune-inflammation index-based nomogram for lung carcinoma following microwave ablation -a real world single center study. Front Oncol 2024;14:1305262. [Crossref] [PubMed]
- Liu J, Gao H, Wang J, et al. Recent research advances on simulation modeling of temperature distribution in microwave ablation of lung tumors. Comput Assist Surg (Abingdon) 2023;28:2195078. [Crossref] [PubMed]
- Furukawa K, Miura T, Kato Y, et al. Microwave coagulation therapy in canine peripheral lung tissue. J Surg Res 2005;123:245-50. [Crossref] [PubMed]
- Wolf FJ, Grand DJ, Machan JT, et al. Microwave ablation of lung malignancies: effectiveness, CT findings, and safety in 50 patients. Radiology 2008;247:871-9. [Crossref] [PubMed]
- Watson RA, Tol I, Gunawardana S, et al. Is microwave ablation an alternative to stereotactic ablative body radiotherapy in patients with inoperable early-stage primary lung cancer? Interact Cardiovasc Thorac Surg 2019;29:539-43. [Crossref] [PubMed]
- Stone J, Hartley-Blossom Z, Healey T. The Emerging Role of Percutaneous Thermal Ablation in the Treatment of Thoracic Malignancies: A Review. Surg Technol Int 2020;36:257-64.
- Iguchi T, Hiraki T, Matsui Y, et al. In Vivo Microwave Ablation of Normal Swine Lung at High-power, Short-duration Settings. Acta Med Okayama 2022;76:57-62. [Crossref] [PubMed]
- Kodama H, Ueshima E, Gao S, et al. High power microwave ablation of normal swine lung: impact of duration of energy delivery on adverse event and heat sink effects. Int J Hyperthermia 2018;34:1186-93. [Crossref] [PubMed]
- Cai H, Tian H, Wei Z, et al. Microwave ablation of the lung: Comparison of 19G with 14G and 16G microwave antennas in ex vivo porcine lung. J Cancer Res Ther 2022;18:1876-83. [Crossref] [PubMed]
- Gillams A. Tumour ablation: current role in the kidney, lung and bone. Cancer Imaging 2009;9 Spec No A:S68-70.
- Carrafiello G, Mangini M, De Bernardi I, et al. Microwave ablation therapy for treating primary and secondary lung tumours: technical note. Radiol Med 2010;115:962-74. [Crossref] [PubMed]
- Zuo Z, Zhang G, Song P, et al. Survival Nomogram for Stage IB Non-Small-Cell Lung Cancer Patients, Based on the SEER Database and an External Validation Cohort. Ann Surg Oncol 2021;28:3941-50. [Crossref] [PubMed]
- Li Z, Xu W, Gu T, et al. Tumor size, but not consolidation-to-tumor ratio, is an independent prognostic factor for part-solid clinical T1 non-small cell lung cancer. Thorac Cancer 2023;14:602-11. [Crossref] [PubMed]
- Wang L, Dou X, Liu T, et al. Tumor size and lymph node metastasis are prognostic markers of small cell lung cancer in a Chinese population. Medicine (Baltimore) 2018;97:e11712. [Crossref] [PubMed]
- Ambrogi MC, Fanucchi O, Cioni R, et al. Long-term results of radiofrequency ablation treatment of stage I non-small cell lung cancer: a prospective intention-to-treat study. J Thorac Oncol 2011;6:2044-51. [Crossref] [PubMed]
- Pine JK, Morris E, Hutchins GG, et al. Systemic neutrophil-to-lymphocyte ratio in colorectal cancer: the relationship to patient survival, tumour biology and local lymphocytic response to tumour. Br J Cancer 2015;113:204-11. [Crossref] [PubMed]
- Ying HQ, Deng QW, He BS, et al. The prognostic value of preoperative NLR, d-NLR, PLR and LMR for predicting clinical outcome in surgical colorectal cancer patients. Med Oncol 2014;31:305. [Crossref] [PubMed]
- Shao D, Chen Y, Huang H, et al. LAG3 blockade coordinates with microwave ablation to promote CD8(+) T cell-mediated anti-tumor immunity. J Transl Med 2022;20:433. [Crossref] [PubMed]
- Zhong WZ, Wang Q, Mao WM, et al. Gefitinib versus vinorelbine plus cisplatin as adjuvant treatment for stage II-IIIA (N1-N2) EGFR-mutant NSCLC (ADJUVANT/CTONG1104): a randomised, open-label, phase 3 study. Lancet Oncol 2018;19:139-48. [Crossref] [PubMed]
- Xu Q, Liu H, Meng S, et al. First-line continual EGFR-TKI plus local ablative therapy demonstrated survival benefit in EGFR-mutant NSCLC patients with oligoprogressive disease. J Cancer 2019;10:522-9. [Crossref] [PubMed]