Ethyl palmitate ameliorates lethal endotoxemia by inducing hepatic fetuin-A secretion: an in vivo and in vitro experiment
Highlight box
Key findings
• The fetuin-A level decreased in sepsis patients compared with non-septic controls based on serum proteome results.
• Ethyl palmitate (EP) treatment can promote hepatic fetuin-A production, reduce pro-inflammatory mediators, regulate macrophage polarization, thus exert protective effects in sepsis and endotoxemia.
What is known and what is new?
• Fetuin-A, a multifunctional plasma glycoprotein induced by EP, is associated with obesity, insulin resistance, non-alcoholic fatty liver disease and inflammation regulation. However, the effect of EP on sepsis and endotoxemia and its mechanisms are unknown.
• EP increases hepatic fetuin-A production, improves survival rate, reduces tissue injury and pro-inflammatory cytokines, and promotes M2 macrophage polarization in sepsis and endotoxemia mice.
What is the implication, and what should change now?
• Targeting fetuin-A with EP may be an effective strategy for reprogramming macrophage polarization to treat sepsis and endotoxemia. However, it still faces many challenges to translate into clinical practice.
Introduction
Sepsis and endotoxemia as a major global health concern are critical conditions caused by dysregulated inflammation of host defense against infection (1). The dysregulated systemic inflammatory cascade causes tissue damage and eventually leads to multisystem organ failure (MSOF) (1-3). Among the organs affected, the lung and liver are the primary and most susceptible targets (1,4). Despite extensive researches on sepsis and endotoxemia, the precise underlying mechanisms remain incompletely understood (4,5). To date, no efficacious Food and Drug Administration (FDA)-approved therapeutic interventions are available, contributing to the high mortality rate (4,6,7). Consequently, new therapeutic approaches are urgently needed.
Sepsis-induced organ injury and failure are attributed to the imbalance between inflammatory and anti-inflammatory responses (8-10). Macrophages are indispensable in host immune response (11,12), which can differentiate into distinct subtypes to promote or suppress inflammation depending on different micro-environment during sepsis and endotoxemia (13). Extensive researches indicate that potential strategies aiming at controlling macrophage polarization can effectively prevent excessive inflammatory response and multiple organ injuries in the management of sepsis (9,14).
Fetuin-A, also termed the alpha-2-HS glycoprotein (AHSG), is an abundant heterodimeric plasma glycoprotein predominantly synthesized by hepatocytes and exhibits multiple physiological and pathological functions (15,16). This glycoprotein has been identified as a novel hepatokine that is intimately linked to obesity, insulin resistance, non-alcoholic fatty liver disease, and the regulation of inflammation (17-19). Moreover, recent proteomic studies have emphasized the essentiality of fetuin-A as a plasma protein, strongly correlated with the survival of coronavirus disease 2019 (COVID-19) patients and downregulated in septic mice induced by cecal ligation and puncture (CLP) (20,21). In addition, researches have suggested that ethyl palmitate (EP) promotes hepatic production of fetuin-A and reduces inflammation in rats (17,22,23). Whether EP also modulates macrophage polarization by inducing hepatic fetuin-A secretion in sepsis and endotoxemia is unclear.
To explore the efficacy and mechanisms of EP in sepsis and endotoxemia, the present study conducted in vivo and in vitro experiments. These results uncovered the role of pharmacologic targeting fetuin-A with EP in mice and its beneficial effect on endotoxin-induced lung and liver injury and proposed a potentially relevant clinical strategy for leveraging EP as a therapeutic target for endotoxemia. We present this article in accordance with the MDAR and ARRIVE reporting checklists (available at https://jtd.amegroups.com/article/view/10.21037/jtd-24-1098/rc).
Methods
Reagents
Lipopolysaccharide (LPS) from Escherichia coli (O111:B4) (Cat. #L4193), dispase II, collagenase IA and deoxyribonuclease I were purchased from Sigma-Aldrich (St Louis, MO, USA). EP (Cat. #HY-N2086) was purchased from MedChem Express (NJ, USA). EP (purity >98%) (Cat. #E103505) and corn oil (Cat. #C116023) were purchased from Shanghai Aladdin Biochemical Technology Co., Ltd. Anti-mouse CD16/CD32 (Cat. #101320), Fixable Viability Dye 510 (Cat. #423111), APC/Cyanine7 rat anti-mouse CD45 (Cat. #147718), Brilliant Violet 605™ rat anti-mouse/human CD11b (Cat. #101257), Brilliant Violet 650™ rat anti-mouse CD86 (Cat. #105036) and Brilliant Violet 785™ rat anti-mouse CD206 (Cat. #141729) were obtained from Biolegend (San Diego, CA, USA). BD Horizon™ BV421 rat anti-mouse F4/80 (Cat. #565411) was obtained from BD Biosciences (San Jose, CA, USA). Mouse interleukin (IL)-1β (Cat. #432604), IL-6 (Cat. #431304) and tumor necrosis factor (TNF)-α (Cat. #430904) enzyme-linked immunosorbent assay (ELISA) kits were purchased from BioLegend. Mouse fetuin-A (Cat. #E-EL-M3069) ELISA kit was obtained from Elabscience Biotechnology Co. Ltd (Wuhan, China).
Experimental design
Proteomic analyses were undertaken utilizing blood samples sourced from sepsis patients and age- and sex-matched non-septic controls. In vivo experiments were designed to assess the effects of administering EP under LPS induced endotoxemia mice. These experiments involved monitoring survival rates, lung and liver injury, serum levels of pro-inflammatory cytokines and fetuin-A, and the proportion of macrophages and M1/M2 subtypes in the lung and liver tissues. Furthermore, the mouse hepatocyte cell line (AML-12) was exposed to different concentrations of EP to demonstrate that EP can promote the production of fetuin-A in hepatic cells in vitro.
Subjects
Patients diagnosed with sepsis admitted to the Surgical Intensive Care Unit (ICU) of Zhongshan Hospital affiliated with Fudan University from July 2018 to March 2021 were included in this study. The inclusion criteria for patients with suspected sepsis were as follows: suspected infection and quick Sequential Organ Failure Assessment (qSOFA) ≥2 (24). In the same time period, non-septic patients (with a SOFA score of 0 and no infection) who were admitted after elective surgery were recruited as control with similar age and sex ratio. The study was conducted in accordance with the Declaration of Helsinki (as revised in 2013). Zhongshan Hospital’s Clinical Research Ethics Committee approved the study protocol (B2021-501R). All participants or their authorized guardians signed an informed consent form. Medical information such as sex, Sequential Organ Failure Assessment score, primary site of infection, and diagnosis were recorded. Blood samples were collected from patients within the first 24 h after admission and performed using liquid chromatography-mass spectrometry (LC-MS) analysis. Proteomic results were further analysed to identify the biological indicators according to previous research (19,21). Differential proteins were determined by t test with P<0.05 and visualized using the R package gplots.
Animals
Wild-type male C57BL/6J mice (6–8 weeks) were derived from Zhejiang Vital River Laboratory Animal Technology Co., Ltd (Zhejiang, China). The mice were raised in a specific pathogen-free facility under a 12-h light/dark cycle at 20–24 °C and 50–60% relative humidity. All animal experiments were approved by the Animal Ethics Committee of Zhongshan Hospital (Approval No. 202311019S) and carried out in strict accordance with the Guide for the Care and Use of Laboratory Animals of the National Institutes of Health (NIH publications, 8th Edition, 2011).
For monitoring the survival of endotoxemia mice, mice were randomly assigned to five experimental groups: the phosphate-buffered saline (PBS) group (n=8), LPS group (n=8), EP + LPS 2 mL/kg group (n=8), EP + LPS 5 mL/kg group (n=8) and EP + LPS 10 mL/kg group (n=8). For changes in inflammation and macrophage polarization, mice were randomly assigned to four experimental groups: the control group (n=3), EP (5 mL/kg) group (n=3), LPS group (n=4), and EP + LPS group (n=4). Endotoxemia was induced with LPS intraperitoneally (a non-lethal dose 10 mg/kg or a lethal dose 15 mg/kg). EP (Cat. #E103505, Aladdin, Shanghai, China) dissolved in corn oil 30% (v/v) was intraperitoneally injected simultaneously with LPS and 12 h beforehand at a dose of 2, 5, 10 mL/kg (selected based on previous work ref.15). Control animals received only PBS vehicle. At 24 h after LPS administration, mice were anesthetized and samples were prepared for subsequent experiments. Anesthetization was induced by intraperitoneal injection using Avertin (Cat. #T48402, Sigma-Aldrich). We selected a small sample size because this experiment would produce homogeneous results during repeated execution. For each experiment, mice originating from the same batch and breeding environment were assigned to each group.
Cell culture and experiments
AML-12 mouse hepatocyte cell line (CRL-2254, ATCC, Manassas, VA, USA) was cultured in DMEM/F12 (Cat. #C11330500VT, Gibco, Waltham, MA, USA) with 10% fetal bovine serum (FBS, Cat. #16000-044, Gibco), 40 ng/mL dexamethasone and 1% penicillin and streptomycin (Cat. # SV30010, Hyclone, Logan, UT, USA) at 37 °C, 5% CO2 incubator. The AML-12 cells were exposed to different concentrations of EP (Cat. #HY-N2086).
Real-time quantitative polymerase chain reaction (RT-PCR)
Total RNA was extracted from lung and liver tissues using TRIzol reagent (Cat. #9109, Takara, Shiga, Japan) according to the manual. The expression levels of target genes were determined using the SYBR Green Master Mix (Cat. #11203ES08, Yeasen, Shanghai, China) with ABI RT-PCR 384 System (Life Technologies, Waltham, MA, USA). Primers used for reverse transcription and PCR are listed in Table S1. The ΔΔCt method relative to levels of the β-actin gene were used to calculate expression levels.
Western blot analysis
Total proteins were extracted using radioimmunoprecipitation assay (RIPA) lysis buffer (Cat. #P0013, Beyotime, Shanghai, China) and assayed using the bicinchoninic acid (BCA) method (Cat. #P0012, Beyotime). The proteins (15 µg per lane) were separated on 10% SDS-PAGE, transferred onto a PVDF membrane and incubated overnight at 4 °C with the primary rabbit polyclonal antibodies against fetuin-A (Cat. #ab187051, 1:2,000, Abcam, Cambridge, UK) and β-actin (Cat. #4967, 1:2,000, CST, Boston, MA, USA). Membranes were washed and incubated with secondary antibody, and imaged using enhanced chemiluminescence (Tanon, Shanghai, China).
ELISA
Serum concentrations of fetuin-A, IL-1β, IL-6, and TNF-α in plasma of mice were assayed using specific ELISA kits according to the manufacturer’s instructions.
Flow cytometry analysis
The lung and liver tissues were removed from mice and prepared into single-cell suspensions as previously described (25,26). The single-cell suspensions were incubated at 4 °C for 15 min with anti-mouse CD16/32 antibody, then labeled with primary or isotype control antibodies at 4 °C for 30 min. Isotype antibodies and unstained controls were set to demonstrate specificity of staining and to establish the criteria for target populations. Subsequently, the cell suspensions were washed twice and analyzed with a flow cytometer (LSR Fortessa, BD Biosciences).
Histological analysis
At the 24th hour after LPS administration, lung and liver tissues were collected and fixed in 4% paraformaldehyde, embedded in paraffin, and sectioned at 5 µm thickness. The sections were stained with haematoxylin and eosin. The histological features were observed and captured blindly under a light microscope by evaluators.
For Immunohistochemistry analysis, liver tissues were incubated with anti-fetuin-A (rabbit, Cat. #GB112376-100, 1:700, Servicebio, Wuhan, China) antibody followed by incubation with the secondary antibody (Cat. #GB23303, 1:200, Servicebio) conjugated with horseradish peroxidase. Pictures were captured using a Carl Zeiss microscope.
Statistical analysis
No data or experiments were excluded from the analysis. Results were presented as mean ± standard deviation (SD) of three independent experiments. Differences between groups were assessed for significance using Student’s t-test or one-way analysis of variance (ANOVA) with Turkey’s correction for post hoc paired comparisons. The Chi-squared test was used for categorical variables. Survival of treatment groups was compared using the log-rank test. Data analyses were performed using GraphPad Prism 8.0 (La Jolla, CA, USA) and FlowJo 10.8.1 (Ashland, OR, USA). Statistical significance was defined as *, P<0.05; **, P<0.01; ***, P<0.001.
Results
Plasma fetuin-A level decreases in septic patients and mice
Our study enrolled 18 non-septic ICU patients (controls) and 55 sepsis patients who met the inclusion criteria. The baseline characteristics and clinical parameters of patients in each group were shown in supplemental information Table S2. The two groups were similar in terms of age, sex ratio and body mass index (BMI). To identify the differential proteins involved in the pathogenesis of sepsis, their expression patterns in the two groups were analysed and compared. The results showed that there were 120 differential proteins between the two groups (data were not shown), among which fetuin-A was decreased in peripheral plasma of sepsis patients compared with non-septics (Figure 1A). Besides, plasma fetuin-A levels were also decreased in endotoxemic mice induced by LPS in a time-dependent fashion, with maximal reduction (by 50–60%) at 24 h after LPS administration (Figure 1B). These data suggest that plasma fetuin-A level is a potential biomarker for sepsis and endotoxemia.
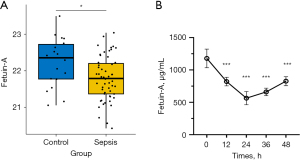
EP promotes production of hepatic fetuin-A
Given that plasma fetuin-A level decreases in septic patients and mice, and EP has been found to promote the synthesis of fetuin-A in non-alcoholic fatty liver disease (17), we analyzed the effects of EP on the hepatic expression of fetuin-A under static and endotoxemia. Mouse hepatocyte cell line AML-12 was treated with EP at different concentrations. The intensity of fetuin-A with the higher molecular weight, the glycosylated form, was gradually enhanced with the increase of EP concentration (Figure 2A). Meanwhile, mice were administered with EP at a dose of 5 mL/kg simultaneously with LPS and 12 h beforehand. Immunohistochemical analysis of murine liver tissues revealed that the region around the central vein was significantly positive (as indicated by the arrows in Figure 2B), suggesting that EP administration significantly upregulated the hepatic expression of fetuin-A. Conversely, the induction of endotoxemia via LPS injection led to a notable downregulation of fetuin-A (Figure 2B). Taken together, these results indicate that the hepatic expression and secretion of fetuin-A increased with EP treatment in mice, and decreased with LPS exposure.
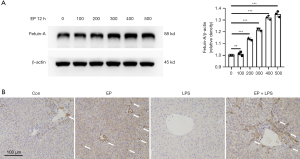
EP improves survival in septic mice and attenuates systemic inflammatory response
To evaluate the effect of EP on endotoxemia, the survival rate was assessed for 7 days in the lethal dose LPS (15 mg/kg, i.p.)-induced septic mice. As presented in Figure 3A, LPS-induced septic mice died after 24 h of LPS injection and the survival rate was 50%. Treatment with EP (2 mL/kg) simultaneously with LPS and 12 h beforehand improved the survival rate of 87.5%, but there was no significance (P=0.21). While administration with EP (5 and 10 mL/kg), the survival rates significantly increased to 100% (P=0.04). Therefore, the subsequent experiments to examine the potential protective effects of EP were carried out using a dose of 5 mL/kg simultaneously with a non-lethal LPS dose of 10 mg/kg and 12 h beforehand. Compared with the control group, LPS increased the serum levels of inflammatory cytokines TNF-α, IL-1β and IL-6 at 24 h (Figure 3B). These effects were significantly attenuated by EP administration (Figure 3B). The above results demonstrate that EP effectively improves the survival rate of septic mice and attenuates production of pro-inflammatory cytokines.
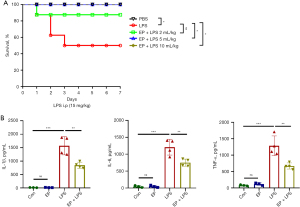
EP alleviates lung injury and macrophage infiltration of septic mice
Hematoxylin and eosin staining was performed to explore the effect of EP on lung injury and revealed that the EP + LPS group exhibited reduced inflammatory cells infiltration, decreased pulmonary edema and a thinner alveolar capillary wall compared with the LPS group (Figure 4A). Furthermore, administering EP simultaneously with LPS and 12 h beforehand markedly suppressed the mRNA expressions of Il1b, Il6, Tnfα and C-C motif chemokine ligand 2 (Ccl2) in the lung tissues (Figure 4B). Meanwhile, flow cytometry was performed to investigate the effect of EP on macrophage infiltration into the lung tissue of mice with endotoxemia. There were no differences in the proportion of macrophages (CD45+CD11b+F4/80+) between the control and EP groups, indicating that EP did not affect macrophage populations in normal lung tissue (Figure 4C). In addition, LPS increased the infiltration of macrophages in the lung tissue, but this was abrogated by EP administration (Figure 4C). Thus, EP administration alleviates lung injury and production of pro-inflammatory cytokines and prevents the infiltration of macrophages into the lung tissue of mice with endotoxemia.
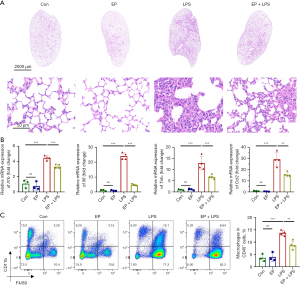
EP attenuates liver injury and macrophage infiltration of septic mice
Sepsis is a systemic inflammatory response, thus the effect of EP on liver injury was also examined. Histopathologic analysis revealed that the EP + LPS group exhibited reduced inflammatory cells infiltration compared with the LPS group (Figure 5A). Additionally, EP co- and pretreatment markedly reduced levels of mRNAs encoding the proinflammatory cytokines of Il1b, Il6, Tnfα and Ccl2 in the liver tissues compared with LPS group (Figure 5B). Flow cytometry analysis was also examined and revealed that the proportion of macrophages (CD45+CD11b+F4/80+) between the control and EP groups had no difference, indicating that EP also did not affect macrophage populations in normal liver tissue (Figure 5C). LPS challenge also significantly promoted the infiltration of macrophages in liver tissue, and this effect was suppressed under EP co- and pretreatment (Figure 5C). These results suggest that EP alleviates pathologic damage and the infiltration of macrophages to liver of mice with endotoxemia.
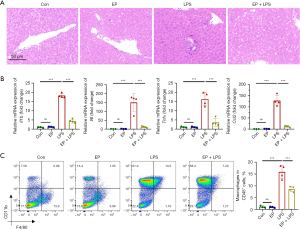
EP reduces inflammation in septic mice via regulating the polarization of macrophages
Preventing excessive inflammation and regulating the balance between M1/M2 polarization are potential strategies for sepsis and endotoxemia management (11). Therefore, we explored the effect of EP on the polarization of liver macrophages in endotoxemia mice. EP treatment decreased the mRNA expression level of M1 marker gene inducible nitric oxide synthase (iNOS) and increased it of M2 marker gene arginase-1 (Arg1) in liver tissue compared with the LPS group (Figure 6A). Additionally, the populations of M1/M2 macrophages in the liver tissue were quantified by flow cytometry. EP administration decreased the population of M1 macrophage (CD86+) and increased it of M2 macrophage (CD206+) in liver tissue compared with the LPS group (Figure 6B,6C). Taken together, these results indicate that EP not only inhibits inflammatory macrophage infiltration into the tissue of endotoxemia mice but also regulates macrophage polarization to suppress systemic inflammation.
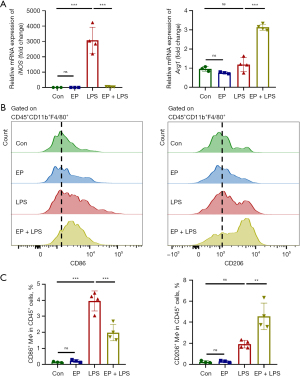
Discussion
Sepsis and endotoxemia, associated with high mortality, involve uncontrolled systemic inflammatory responses induced by activated innate and adaptive immunity, leading to multiple organ dysfunction (27,28). Despite significant strides in understanding the treatment and mechanisms of sepsis in recent decades, the quest for effective pharmacotherapy remains elusive (7,29). Modulating macrophage polarization may help attenuate inflammation and improve outcomes in patients with sepsis and endotoxemia (11,30). The present study elucidates one possible strategy for endotoxemia by showing that fetuin-A induced by EP improves survival rate, ameliorates lung and liver injury and reduces expression of pro-inflammatory mediators and macrophage infiltration in mice with LPS-induced endotoxemia. Flow cytometric analyses further suggest that EP may shift macrophage polarization from M1 to M2 phenotype in the liver of septic mice.
Previous research has provided evidence that fetuin-A can modulate cytokine production partly by inhibiting active high mobility group box 1 protein (HMGB1) release in mice (31). Supplementation of fetuin-A significantly attenuates the proinflammatory response and increases survival rates in both CLP and LPS-induced endotoxemia murine models, while genetic knocking out of fetuin-A eliminates this effect (31). Fetuin-A is a potential target for inflammatory disease that is mainly produced by liver cells (15,31). In the current study, based on our proteomic results, we found that the level of fetuin-A in plasma was significantly lower in sepsis patients compared with non-septic controls. Although the two groups were selected for age and sex matching (as shown in Table S2), due to the lack of more detailed clinical data, there may be a selection bias, which will be better avoided in future studies. Furthermore, consistent with previous findings, our study demonstrated a significant reduction of plasma fetuin-A level in mice after intraperitoneal administration of LPS, especially at 24 h (31-33). These results suggest that fetuin-A plays a critical role in sepsis and endotoxemia, and that plasma fetuin-A level may be a useful biomarker for sepsis.
EP has been demonstrated to be rapidly hydrolyzed to free palmitate in the blood (34), which can stimulate the production of fetuin-A (23). Consistent with these findings, our study demonstrated that EP significantly increased the production of fetuin-A in mouse hepatocyte cell line AML-12 and liver tissue after EP administration by immunoblotting and immunohistochemical staining. It has been shown that fetuin-A is a multifunctional protein that exhibits different pro- or anti-inflammatory effects in different disease, while it can induce inflammatory cytokine expression and suppress adiponectin production in atherosclerosis and insulin resistance (35,36). On the contrary, Zhao et al. have reported that fetuin-A suppress oxidative stress and necroptosis levels in glutamate-treated microglial cells, thereby attenuating the inflammation following traumatic brain injury (19). Consequently, to evaluate the effect of fetuin-A induced by EP on inflammation during sepsis and lethal endotoxemia, we conducted a mouse model of LPS induced endotoxemia. Our results suggested that EP co and pre-treatment protected mice against the lethal endotoxemia, alleviated pathological damage, reduced the expression of pro-inflammatory cytokines and chemokines and blocked the infiltration of macrophages into the murine lung and liver tissue.
Macrophages are critical immune populations in sepsis and endotoxemia that not only modulate immune homeostasis but also promote the resolution of inflammation and repair of tissues (37). At the initial of sepsis and endotoxemia, stimuli such as LPS and interferon (IFN)-γ orchestrate the macrophages polarization towards an M1-like phenotype. These M1 macrophages can produce pro-inflammatory cytokines, including IL-6, IL-1β, TNF-α, iNOS and Ccl2, while expressing surface markers CD68, CD80 and CD86 (12). In contrast, the M2 macrophages mainly promote the resolution of inflammation, angiogenesis and tissue remodeling in the late stage of sepsis. These cells mainly express Arg-1 and CD206 (12). Besides, Rudloff et al. have demonstrated that fetuin-A is a hypoxia-inducible factor (HIF) target that modulates macrophage polarization and attenuates inflammation to preserve kidney function under hypoxia (38). In this study, our results suggested that EP also regulated macrophage polarization towards M2-like subtype thereby alleviating sepsis-induced inflammatory cytokine storm. These findings reveal that EP may be an effective treatment for sepsis and endotoxemia.
There are some limitations in this study. First, the study was designed to assess the protective effect of EP only in LPS induced septic mice. Therefore, the effect of EP in other sepsis mice models were not examined. Second, although flow cytometry analysis showed EP promoted macrophage polarization towards M2-like subtype in liver tissue during sepsis, more experimental studies are needed to clarify the specific mechanisms. Furthermore, using immortal cell lines may not fully represent the in vivo situation, further experiments with primary hepatocytes and knock-out mice or pharmacological inhibitors are warranted to elucidate the relevant pathways.
Conclusions
In conclusion, our study presents the inaugural evidence of the protective effect of EP against sepsis and endotoxemia through inhibiting pro-inflammatory factor production and modulating macrophage polarization by inducing hepatic fetuin-A secretion. These findings will facilitate further investigations and application of EP in the treatment of sepsis and endotoxemia.
Acknowledgments
We thank laboratory technicians in central laboratory and all staff in Department of Zhongshan Hospital of China for assistance with animal caretaking and laboratory analyses.
Funding: This work was supported by
Footnote
Reporting Checklist: The authors have completed the MDAR and ARRIVE reporting checklists. Available at https://jtd.amegroups.com/article/view/10.21037/jtd-24-1098/rc
Data Sharing Statement: Available at https://jtd.amegroups.com/article/view/10.21037/jtd-24-1098/dss
Peer Review File: Available at https://jtd.amegroups.com/article/view/10.21037/jtd-24-1098/prf
Conflicts of Interest: All authors have completed the ICMJE uniform disclosure form (available at https://jtd.amegroups.com/article/view/10.21037/jtd-24-1098/coif). M.Z. serves as an unpaid editorial board member of Journal of Thoracic Disease from June 2024 to June 2026. The other authors have no conflicts of interest to declare.
Ethical Statement: The authors are accountable for all aspects of the work in ensuring that questions related to the accuracy or integrity of any part of the work are appropriately investigated and resolved. The study was conducted in accordance with the Declaration of Helsinki (as revised in 2013). Zhongshan Hospital’s Clinical Research Ethics Committee approved the study protocol (B2021-501R). All participants or their authorized guardians signed an informed consent form. All animal experiments were approved by the Animal Ethics Committee of Zhongshan Hospital (Approval No.202311019S) and carried out in strict accordance with the Guide for the Care and Use of Laboratory Animals of the National Institutes of Health (NIH publications, 8th Edition, 2011).
Open Access Statement: This is an Open Access article distributed in accordance with the Creative Commons Attribution-NonCommercial-NoDerivs 4.0 International License (CC BY-NC-ND 4.0), which permits the non-commercial replication and distribution of the article with the strict proviso that no changes or edits are made and the original work is properly cited (including links to both the formal publication through the relevant DOI and the license). See: https://creativecommons.org/licenses/by-nc-nd/4.0/.
References
- Singer M, Deutschman CS, Seymour CW, et al. The Third International Consensus Definitions for Sepsis and Septic Shock (Sepsis-3). JAMA 2016;315:801-10. [Crossref] [PubMed]
- van der Poll T, van de Veerdonk FL, Scicluna BP, et al. The immunopathology of sepsis and potential therapeutic targets. Nat Rev Immunol 2017;17:407-20. [Crossref] [PubMed]
- Liu B, Zhou Q. Clinical phenotypes of sepsis: a narrative review. J Thorac Dis 2024;16:4772-9. [Crossref] [PubMed]
- Huang M, Cai S, Su J. The Pathogenesis of Sepsis and Potential Therapeutic Targets. Int J Mol Sci 2019;20:5376. [Crossref] [PubMed]
- Schlapbach LJ, de Oliveira CF, Raman S, et al. Metabolic resuscitation in pediatric sepsis: a narrative review. Transl Pediatr 2021;10:2678-88. [Crossref] [PubMed]
- Niederman MS, Baron RM, Bouadma L, et al. Initial antimicrobial management of sepsis. Crit Care 2021;25:307. [Crossref] [PubMed]
- Rudd KE, Johnson SC, Agesa KM, et al. Global, regional, and national sepsis incidence and mortality, 1990-2017: analysis for the Global Burden of Disease Study. Lancet 2020;395:200-11. [Crossref] [PubMed]
- Delano MJ, Ward PA. Sepsis-induced immune dysfunction: can immune therapies reduce mortality? J Clin Invest 2016;126:23-31. [Crossref] [PubMed]
- Wang Z, Wang Z. The role of macrophages polarization in sepsis-induced acute lung injury. Front Immunol 2023;14:1209438. [Crossref] [PubMed]
- Chousterman BG, Swirski FK, Weber GF. Cytokine storm and sepsis disease pathogenesis. Semin Immunopathol 2017;39:517-28. [Crossref] [PubMed]
- Chen X, Liu Y, Gao Y, et al. The roles of macrophage polarization in the host immune response to sepsis. Int Immunopharmacol 2021;96:107791. [Crossref] [PubMed]
- Locati M, Curtale G, Mantovani A. Diversity, Mechanisms, and Significance of Macrophage Plasticity. Annu Rev Pathol 2020;15:123-47. [Crossref] [PubMed]
- Liu YC, Zou XB, Chai YF, et al. Macrophage polarization in inflammatory diseases. Int J Biol Sci 2014;10:520-9. [Crossref] [PubMed]
- Hu Q, Lyon CJ, Fletcher JK, et al. Extracellular vesicle activities regulating macrophage- and tissue-mediated injury and repair responses. Acta Pharm Sin B 2021;11:1493-512. [Crossref] [PubMed]
- Mori K, Emoto M, Inaba M. Fetuin-A: a multifunctional protein. Recent Pat Endocr Metab Immune Drug Discov 2011;5:124-46. [Crossref] [PubMed]
- Chekol Abebe E, Tilahun Muche Z. The structure, biosynthesis, and biological roles of fetuin-A: A review. Front Cell Dev Biol 2022;10:945287. [Crossref] [PubMed]
- Jung TW, Youn BS, Choi HY, et al. Salsalate and adiponectin ameliorate hepatic steatosis by inhibition of the hepatokine fetuin-A. Biochem Pharmacol 2013;86:960-9. [Crossref] [PubMed]
- Gerst F, Wagner R, Kaiser G, et al. Metabolic crosstalk between fatty pancreas and fatty liver: effects on local inflammation and insulin secretion. Diabetologia 2017;60:2240-51. [Crossref] [PubMed]
- Zhao P, Wei Y, Sun G, et al. Fetuin-A alleviates neuroinflammation against traumatic brain injury-induced microglial necroptosis by regulating Nrf-2/HO-1 pathway. J Neuroinflammation 2022;19:269. [Crossref] [PubMed]
- Miao H, Chen S, Ding R. Evaluation of the Molecular Mechanisms of Sepsis Using Proteomics. Front Immunol 2021;12:733537. [Crossref] [PubMed]
- Völlmy F, van den Toorn H, Zenezini Chiozzi R, et al. A serum proteome signature to predict mortality in severe COVID-19 patients. Life Sci Alliance 2021;4:e202101099. [Crossref] [PubMed]
- Saeed NM, El-Demerdash E, Abdel-Rahman HM, et al. Anti-inflammatory activity of methyl palmitate and ethyl palmitate in different experimental rat models. Toxicol Appl Pharmacol 2012;264:84-93. [Crossref] [PubMed]
- Mukhopadhyay S, Mondal SA, Kumar M, et al. Proinflammatory and antiinflammatory attributes of fetuin-a: a novel hepatokine modulating cardiovascular and glycemic outcomes in metabolic syndrome. Endocr Pract 2014;20:1345-51. [Crossref] [PubMed]
- Vincent JL, Moreno R, Takala J, et al. The SOFA (Sepsis-related Organ Failure Assessment) score to describe organ dysfunction/failure. On behalf of the Working Group on Sepsis-Related Problems of the European Society of Intensive Care Medicine. Intensive Care Med 1996;22:707-10. [Crossref] [PubMed]
- Daemen S, Chan MM, Schilling JD. Comprehensive analysis of liver macrophage composition by flow cytometry and immunofluorescence in murine NASH. STAR Protoc 2021;2:100511. [Crossref] [PubMed]
- Liu Z, Gu Y, Shin A, et al. Analysis of Myeloid Cells in Mouse Tissues with Flow Cytometry. STAR Protoc 2020;1:100029. [Crossref] [PubMed]
- Zhang YY, Ning BT. Signaling pathways and intervention therapies in sepsis. Signal Transduct Target Ther 2021;6:407. [Crossref] [PubMed]
- Ranjit S, Kissoon N. Challenges and Solutions in translating sepsis guidelines into practice in resource-limited settings. Transl Pediatr 2021;10:2646-65. [Crossref] [PubMed]
- Sun Y, Song J, Liu H, et al. Calcium-sensing receptor alleviates gut damage caused by endotoxemia by regulating gut microbiota. Transl Pediatr 2023;12:2179-90. [Crossref] [PubMed]
- Delano MJ, Ward PA. The immune system's role in sepsis progression, resolution, and long-term outcome. Immunol Rev 2016;274:330-53. [Crossref] [PubMed]
- Li W, Zhu S, Li J, et al. A hepatic protein, fetuin-A, occupies a protective role in lethal systemic inflammation. PLoS One 2011;6:e16945. [Crossref] [PubMed]
- Daveau M, Davrinche C, Djelassi N, et al. Partial hepatectomy and mediators of inflammation decrease the expression of liver alpha 2-HS glycoprotein gene in rats. FEBS Lett 1990;273:79-81. [Crossref] [PubMed]
- Daveau M. The synthesis of human alpha-2-HS glycoprotein is down-regulated by cytokines in hepatoma HepG2 cells. FEBS Lett 1988;241:191-4. [Crossref] [PubMed]
- Ogawa Y, Imajo K, Honda Y, et al. Palmitate-induced lipotoxicity is crucial for the pathogenesis of nonalcoholic fatty liver disease in cooperation with gut-derived endotoxin. Sci Rep 2018;8:11365. [Crossref] [PubMed]
- Hennige AM, Staiger H, Wicke C, et al. Fetuin-A induces cytokine expression and suppresses adiponectin production. PLoS One 2008;3:e1765. [Crossref] [PubMed]
- Pal D, Dasgupta S, Kundu R, et al. Fetuin-A acts as an endogenous ligand of TLR4 to promote lipid-induced insulin resistance. Nat Med 2012;18:1279-85. [Crossref] [PubMed]
- Shapouri-Moghaddam A, Mohammadian S, Vazini H, et al. Macrophage plasticity, polarization, and function in health and disease. J Cell Physiol 2018;233:6425-40. [Crossref] [PubMed]
- Rudloff S, Janot M, Rodriguez S, et al. Fetuin-A is a HIF target that safeguards tissue integrity during hypoxic stress. Nat Commun 2021;12:549. [Crossref] [PubMed]