Effectiveness of pulmonary rehabilitation on exercise capacity in adult patients with lung transplantation: a systematic review and single-arm meta-analysis
Highlight box
Key findings
• In this study we found that pulmonary rehabilitation (PR) shows evidence for being an effective adjunctive strategy for improving exercise capacity and muscle strength after lung transplantation (LTx).
What is known and what is new?
• PR is an effective way to improve exercise capacity and muscle strength in adult LTx recipients.
• This manuscript found that the duration of PR and the interval between LTx and PR may be the key factors influencing the enhancement of exercise capacity in adult LTx recipients.
What is the implication, and what should change now?
• This study will further validate the positive effect of PR on improving the exercise capacity in adults LTx recipients, while the results also suggest that the efficacy of PR may be influenced by the intervention duration and the interval between LTx and PR.
Introduction
Lung transplantation (LTx) is the last option for the treatment of end-stage lung disease that is not responsive to other treatments. As a result of advances in surgical techniques, peri-operative management, and immunosuppression strategies, the survival rate after LTx has seen a significant increase, with the median survival ranging from 4 to 6 years (1). However, exercise tolerance, as assessed by the 6-minute walking test (6MWT), is only 40–60% of the normal values, and is, therefore, still limited and poses a big obstacle to recovery after LTx (2). In addition, Bourgeois et al. have confirmed that the 6-minute walking distance (6MWD) and maximum oxygen consumption (VO2max) are important predictors of survival after LTx (3). Muscle strength, such as handgrip force (HGF) and quadriceps force (QF), are other variables that can be used as predictor of sarcopenia and other clinical and functional outcomes after LTx (4). Polastri et al. have even reported that handgrip seems to be positively related with lung function in various clinical populations (5). This implies that improving muscle strength is essential for improving exercise capacity in LTx recipients.
Pulmonary rehabilitation (PR) is a comprehensive intervention that has many benefits for patients with chronic respiratory disease (6). Schneeberger et al. showed that PR contributes to improving exercise tolerance, muscle strength, and health-related quality of life, and reducing symptoms and hospitalization in patients with chronic respiratory disease (7). Considering these benefits, PR may also be effective in patients after LTx. Previous studies have recommended PR as an optimal treatment for patients with heart (8) or kidney transplantation (9). However, because of the complexity of LTx, there is no clear consensus of whether PR is also beneficial for this group of patients.
Evidence to support the benefits of PR after LTx has been published in a few previous reviews (10-12). However, due to the variable design of the studies on this topic, no reliable meta-analysis has been conducted so far. We try to fill in this gap, and we have performed this single-arm meta-analysis to assess the efficacy of PR in LTx recipients. We present this article in accordance with the PRISMA reporting checklist (available at https://jtd.amegroups.com/article/view/10.21037/jtd-24-568/rc) (13).
Methods
We used a comprehensive retrieval method to search in Cochrane library and the International Prospective Register of Systematic Reviews (PROSPERO) and confirmed that no similar meta-analysis had been conducted. This single-arm meta-analysis was then registered at PROSPERO (www.crd.york.ac.uk/prospero, registration No. CRD42023460247).
Search strategy
Based on the methods employed by previously published systematic reviews in the field, we formulated a strategy to search for relevant studies in the following electronic databases: MEDLINE, Embase, CINAHL, Web of Science. Besides, we set weekly automatic updates for retrieval of relevant studies from the databases until January 2024 in order to ensure that no studies were left out. Combinations of the following keywords were used for searching: (“lung transplantation” OR “organ transplantation”) AND (“pulmonary rehabilitation” OR “exercise” OR “resistance training” OR “physical therapy” OR “aerobic exercise” OR “physical fitness” OR “balance training” OR “flexibility training” OR “stretch training” OR “education”). The search was limited to keywords and terms found in titles or abstracts. Additionally, reference lists and published systematic reviews were scanned by manual searching.
Inclusion and exclusion criteria
We screened studies according to the PICOS criteria (participants, interventions, comparators, outcomes, and study type). (I) Participants—adult LTx recipients irrespective of whether they underwent single or double LTx; (II) interventions: any form of PR (e.g., exercise, nutrition, education); (III) comparisons: preintervention; (IV) outcomes: primary outcome was exercise capacity (6MWT, VO2max) and secondary outcomes were muscle strength (handgrip strength, quadriceps strength); (V) study design: randomized controlled trials (RCTs) or quasi-experimental studies (single-group pretest-posttest design) or cohort study; (VI) language: studies published in English.
Exclusion criteria were as follows: (I) nonexperimental studies (e.g., conference abstracts or case reports, reviews, letters); (II) studies in which most of the participants required other types of surgery, in addition to LTx, such as transplantation of other organs and other heart surgery; (III) non-English studies; (IV) studies with insufficient data even after the author was contacted.
Study selection
After duplicate studies were removed, two authors (P.W. and B.G.) independently screened the titles and abstracts of the remaining studies. A third author (S.W.) solved any disagreements that arose between the two. Finally, studies were included after full-text inspection according to the inclusion and exclusion criteria.
Data extraction
Two authors (P.W. and B.G.) were responsible for collecting data on the following variables from the eligible studies: basic information (first author, year of publication, and location), participants’ characteristics (sample size, age, sex, body mass index, and original disease), interventions prescribed (exercise components, frequency of sessions, duration, and intensity), primary and secondary outcome.
Risk of bias assessment
Two authors (P.W. and B.G.) independently performed risk of bias assessment of the included studies, and the third author (S.W.) solved any disagreements that arose. The Physiotherapy Evidence (PEDro) Scale was utilized for RCTs, and the National Heart, Lung, and Blood Institute (NHLBI) assessment tool was used for quasi-experimental studies.
Statistical analysis
In this single-arm meta-analysis, for quasi-experimental trials we compared pre- and post-intervention outcomes, whereas RCTs were compared for pre- and post-intervention outcomes in the interventional group. In addition, for some included RCTs, in which participants in both experimental and control group received different types of PR, we split them as independent quasi-experimental studies.
All data extracted from eligible studies were analyzed with STATA (version 17.0) in this meta-analysis. Heterogeneity among studies was analyzed by calculating I2: values ≥50% were considered to indicate a high degree of heterogeneity between studies. A random-effects model was used for analysis when I2 was ≥50% (that is, when the heterogeneity was high), and a fixed-effects model was used when the heterogeneity was low. Besides, if the heterogeneity existed, we carried out sensitivity analysis by omitting each study one at a time to evaluate the robustness of the pooled estimates. We also performed meta-regression analysis and subgroup analysis by durations of PR (duration <4 weeks, 4≤ duration <8 weeks, 8≤ duration <12 weeks and duration ≥12 weeks) and intervals between LTx and PR (interval <1 month, 1≤ interval <12 months and interval ≥12 months) to access resources of heterogeneity. Continuous data were expressed as weighted mean difference (WMD) or standard mean difference (SMD), and 95% confidence intervals (CIs) were calculated. Moreover, we acquired data from figures in the individual study reports if it was necessary. To facilitate interpretation of SMDs, we utilized cut-offs as follows: 0.2–0.5 (small effect), 0.5–0.8 (moderate effect) and >0.8 (large effect) (14). We performed Egger’s test to assess publication bias.
Results
Study characteristics
A total of 2,845 studies were obtained after the initial search. After screening, 21 studies (15-35) [12 quasi-experimental studies (15-23,33-35) and 9 RCTs (24-32)] with 1,597 participants (57.1% male) were included in the meta-analysis (Figure 1). Twenty-one included studies were from Australia (n=5), Germany (n=5), Belgium (n=2), China (n=1), USA (n=2), Norway (n=1), Israel (n=1), Austria (n=1), Turkey (n=1), Britain (n=1) and Hungary (n=1). The published year ranged from 1998 to 2024. The type of LTx performed was reported in 18 studies with 1,039 participants (15,17-26,29-35), and the majority of the patients (70.0%) underwent double lung transplantation (DLT). Besides, 1 study reported the effects of PR on single lung transplantation (SLT) and DLT separately (24), and 2 studies recruited only DLT recipients (19,34). The time interval between LT and the start of PR ranged from 4 days to 4.6 years. All participants received immunosuppressive therapy during the PR. The baseline demographic and clinical characteristics of the study participants are summarized in Table 1.
Table 1
Reference, publication year, country | Design | Group | Simple size (M/F) | Age (y) | Transplant type (DLT/SLT) |
Interval between LTx and PR | Interventions | Duration | Outcomes |
---|---|---|---|---|---|---|---|---|---|
Ihle, 2011, Germany | RCT | EG | 30 (13/17) | 49 [13.7] | 19/11 | 4.5 [3.2] y | (I) Endurance training; (II) upper and lower strength training; (III) stretching; (IV) range-of-motion exercises; (V) education; (VI) breath exercise | 23 [5] d | 6MWD; VO2max |
CG | 30 (13/17) | 50 [12.3] | 20/10 | (I) Airway clearance; (II) breath exercise; (III) cardiovascular exercise | 18 [3] d | ||||
Langer, 2012, Belgium | RCT | EG | 18 (9/9) | 59 [4] | 15/3 | 5.8 [3.9] m | (I) Cycling; (II) walking; (III) lower limb strength training | 20 weeks | 6MWD; VO2max; QF; HGF |
Gloeckl, 2015, Germany | RCT | EG | 34 (16/18) | 56 [7] | 27/7 | 2.5 [3] m | (I) Cycling; (II) breath exercise; (III) strength training; (IV) education; (V) whole body vibration therapy | 4 weeks | 6MWD; QF |
CG | 36 (22/14) | 56 [7] | 20/16 | (I) Cycling; (II) breath exercise; (III) strength training; (IV) education | |||||
Fuller, 2017, Australia | RCT | EG | 32 (17/15) | 51.7 [13.4] | 28/4 | 0.62 [0.25] m | First 7 weeks (I) treadmill and cycling; (II) upper and lower limb strength training; (III) core stability training; (IV) functional exercise training. After that, participants were encouraged to continue at home | 7 weeks | 6MWD; QF |
CG | 34 (14/20) | 49.8 [14] | 29/5 | (I) Treadmill and cycling; (II) upper and lower limb strength training; (III) core stability training; (IV) functional exercise training | 14weeks | ||||
Fuller, 2018, Australia | RCT | EG | 37 (20/17) | 55.9 [10.6] | NA | 0.6 m | (I) Range of joint movement; (II) shoulder strength training; (III) treadmill and cycling; (IV) lower limb strengthening. | 6 weeks | 6MWD; QF |
CG | 43 (17/26) | 56.12 [12.2) | (I) Treadmill and cycling; (II) lower limb strengthening | ||||||
Ulvestad, 2020, Norway | RCT | EG | 25 (11/14) | 52.3 [11.9] | NA | 2.52 [1.38] y | (I) Treadmill walking; (II) upper and lower limbs strength training; (III) back extension | 20 weeks | VO2max; QF |
Wu, 2022, China | RCT | EG | 48 (42/6) | 56.0 [11.5] | 16/32 | – | (I) Position management; (II) passive or active bedside leg cycling training; (III) neuromuscular electrical stimulation; (IV) lower and upper limbs training; (V) walking (assisted) | 4 weeks | 6MWD |
CG | 48 (40/8) | 58.1 [10.4] | 20/28 | (I) Early tracheal extubation combined with early exercise | |||||
Nissan, 2022, Israel | RCT | EG1 | 6 (4/2) | 57.3 [11.5] | 3/3 | 13.6 [4.4] m | (I) Treadmill and cycling; (II) upper and lower strength training; (III) stretch and range of motion | 16 weeks | 6MWD; VO2max |
EG2 | 6 (3/3) | 59.8 [4.4] | 5/1 | 13.6 [5.3] m | (I) Treadmill and cycling; (II) upper and lower strength training; (III) stretch and range of motion; (IV) inspiratory muscle training | ||||
EG3 | 5 (4/1) | 39.4 [17.7] | 2/3 | 15 [3.5] m | (I) Inspiratory muscle training | ||||
Tarrant, 2023, Australia | RCT | EG | 21 (11/10) | 64 | 21/0 | 4 d | Twice-daily physiotherapy, including (I) early mobility; (II) endurance exercise; (III) upper and lower strength; (IV) flexibility and trunk mobility; (V) education; (VI) psychosocial support | 10 weeks | 6MWD; HGF |
CG | 19 (13/6) | 60 | 19/0 | Once-daily physiotherapy, including (I) early mobility; (II) endurance exercise; (III) upper and lower strength; (IV) flexibility and trunk mobility; (V) education; (VI) psychosocial support | |||||
Emsley, 2022, Australia | Quasi-experimental studies | EG | 15 (11/4) | 67.9 | 9/6 | 14 d | (I) Physiotherapist-supervised group cardiovascular and strength training; (II) group education; (III) dietary management | 12 weeks | 6MWD |
CG | 14 (10/4) | 59.8 | 13/1 | (I) Physiotherapist-supervised group cardiovascular and strength training | |||||
Stiebellehner, 1998, Austria | Quasi-experimental studies | – | 9 (6/3) | 44 [6] | 7/2 | 1 [0.5] y | Cycling | 6 weeks | VO2max |
Maury, 2008, Belgium | Quasi-experimental studies | – | 36 (17/19) | 58 [4] | 32/4 | 1.25 m | (I) Cycling; (II) lower limb strength training | 12 weeks | 6MWD; QF; HGF |
Munro, 2009, Australia | Quasi-experimental studies | – | 36 (18/18) | 46 [14] | 29/7 | 1 m | (I) Stationary cycling and treadmill walking; (II) upper and lower limb strength training; (III) stretches of major muscle groups; (IV) range of motion of the major joints; (V) education | 12 weeks | 6MWD |
Choi, 2016, USA | Quasi-experimental studies | EG | 4 (3/1) | 52.5 [21.7] | 2/2 | 1.84 [0.8] m | (I) Education; (II) strengthening exercises; (III) walking | 8 weeks | 6MWD |
Schneeberger, 2017, Germany | Quasi-experimental studies | – | 722 (396/326) | 55.5 [7.5] | 458/264 | 2 [0.5] m | (I) Cycling; (II) lower limb strength training; (III) breath training; (IV) ADL training | 6 weeks | 6WMD |
Andrianopoulos, 2019, Germany | Quasi-experimental studies | – | 24 (14/10) | 58.2 [6.3] | 16/8 | 1 m | (I) Cycling and treadmill walking; (II) upper and lower strength training | 3 weeks | 6WMD |
Candemir, 2019, Turkey | Quasi-experimental studies | – | 17 (15/2) | 47 [10] | 17/0 | 2.5 [0.5] m | (I) Education; (II) cycling and treadmill; (III) upper and lower limb strength training | 8 weeks | ISWT; HGF; QF |
Kerti, 2021, Hungary | Quasi-experimental studies | – | 14 (11/3) | 52 [8.8] | NA | 2 m | (I) Controlled breathing technics, chest wall stretching and chest mobility improving technics; (II) bicycle or treadmill | 4 weeks | 6MWD |
Brunner, 2016, Germany | Quasi-experimental studies | – | 10 (5/5) | 49.8 [11.4] | 8/2 | 13 [10.4] d | Whole-body vibration therapy | 10 [2.0] d | 6MWD |
Smith, 2018, USA | Quasi-experimental studies | – | 10 (10/0) | 59 | 6/4 | 19.5 d | (I) Treadmill walking; (II) lower limbs strength training | 4 weeks | 6MWD; QF; HGF |
Byrd, 2024, Britain | Quasi-experimental studies | EG1 | 110 (63/47) | 60.5 | 103/7 | 19.0 d | Participants accepted group rehabilitation as followed: (I) stationary cycle; (II) upper and lower limbs progressive resistance exercise; (III) balance training; (IV) group exercise class; (V) education lectures (in person, daily) | 6–7 weeks | 6MWD |
EG2 | 105 (69/36) | 65.0 | 96/9 | 23.0 d | Participants accepted individual rehabilitation as followed: (I) stationary cycle; (II) upper and lower limbs progressive resistance exercise; (III) education lectures (on zoom, daily) |
Data are shown as n, mean [SD], or mean. RCT, randomized controlled trial; M, male; F, female; EG, experimental group; CG, control group; y, years; NA, not applicable; DLT, double lung transplantation; SLT, single lung transplantation; LTx, lung transplantation; PR, pulmonary rehabilitation; m, months; d, days; ADL, activity of daily life; 6MWD, 6-minute walking distance; VO2max, maximum oxygen consumption; QF, quadriceps force; HGF, handgrip force; ISWT, incremental shuttle walk test; SD, standard deviation.
Intervention characteristics
In this meta-analysis, we included 21 studies with 33 arms. The duration of PR ranged from 3 to 24 weeks. Among 9 RCTs included, participants from 7 of these RCTs accepted PR. Participants in 19 arms received endurance training (treadmill walking and stationary cycling). Participants from 18 studies received muscle strength training (lower with or without upper limb strength training) (see Table 1).
Risk of bias assessment
The PEDro scale was used to perform risk of bias assessment for RCTs (24-32). The total score ranged from 5 to 8 (see Table 2). As a result of variations in the study design, all the participants and researchers were not blinded to the study. The results of risk of bias assessment for quasi-experimental studies are detailed in Table 3 (15-23,33-35). All the included studies clearly stated the study question, listed pre-specified inclusion criteria, comprised participants who were representative of LTx recipients, and clearly defined the intervention and outcome variables. A noteworthy observation was that the percentage of participants who were lost to follow-up was low in all the included studies; this indicates good compliance with the PR regimen.
Table 2
Reference | Q1 | Q2 | Q3 | Q4 | Q5 | Q6 | Q7 | Q8 | Q9 | Q10 | Q11 | Total |
---|---|---|---|---|---|---|---|---|---|---|---|---|
Ihle [2011] | 1 | 1 | 0 | 1 | 0 | 0 | 0 | 0 | 0 | 1 | 1 | 5 |
Langer [2012] | 1 | 1 | 1 | 1 | 0 | 0 | 1 | 1 | 0 | 1 | 1 | 8 |
Gloeckl [2015] | 1 | 1 | 0 | 1 | 0 | 0 | 0 | 1 | 1 | 1 | 1 | 7 |
Fuller [2017] | 1 | 1 | 1 | 1 | 0 | 0 | 0 | 1 | 1 | 1 | 1 | 8 |
Fuller [2018] | 1 | 1 | 0 | 1 | 0 | 0 | 0 | 1 | 1 | 1 | 1 | 7 |
Ulvestad [2020] | 1 | 1 | 1 | 1 | 0 | 0 | 0 | 1 | 1 | 1 | 1 | 8 |
Wu [2022] | 1 | 1 | 0 | 1 | 0 | 0 | 0 | 0 | 1 | 1 | 1 | 6 |
Nissan [2022] | 1 | 1 | 0 | 1 | 0 | 0 | 0 | 0 | 0 | 1 | 1 | 5 |
Tarrant [2023] | 1 | 1 | 0 | 1 | 0 | 0 | 0 | 0 | 1 | 1 | 1 | 6 |
Q1, eligibility criteria and source; Q2, random allocation; Q3, concealed allocation; Q4, baseline comparability; Q5, blinding of participants; Q6, blinding of therapies; Q7, blinding of assessors; Q8, adequate follow-up; Q9, intention-to-treat analysis; Q10, between-group statistical comparisons; Q11, reporting of point measures and measures of variability.
Table 3
Reference | Q1 | Q2 | Q3 | Q4 | Q5 | Q6 | Q7 | Q8 | Q9 | Q10 | Q11 | Q12 |
---|---|---|---|---|---|---|---|---|---|---|---|---|
Stiebellehner [1998] | Y | Y | Y | Y | Y | Y | Y | N | Y | Y | N | NA |
Maury [2008] | Y | Y | Y | Y | Y | Y | Y | N | Y | Y | N | NA |
Munro [2009] | Y | Y | Y | Y | N | Y | Y | N | N | Y | N | NA |
Choi [2016] | Y | Y | Y | Y | N | Y | Y | N | Y | Y | N | NA |
Schneeberger [2017] | Y | Y | Y | Y | N | Y | Y | N | Y | Y | N | N |
Andrianopoulos [2019] | Y | Y | Y | Y | N | Y | Y | N | Y | Y | N | NA |
Candemir [2019] | Y | Y | Y | Y | N | Y | Y | N | Y | Y | N | N |
Kerti [2021] | Y | Y | Y | Y | N | N | Y | N | Y | Y | N | N |
Brunner [2016] | Y | Y | Y | Y | N | Y | Y | N | Y | Y | N | N |
Barbara [2018] | Y | Y | Y | Y | N | Y | Y | N | Y | Y | N | N |
Emsley [2022] | Y | Y | Y | Y | N | N | Y | N | Y | Y | N | N |
Rebecca [2024] | Y | Y | Y | Y | N | Y | Y | N | Y | Y | N | N |
Q1, Was the study question clearly stated? Q2, Were the eligibility/selection criteria for the study population pre-specified and clearly described? Q3, Were the participants in the study representative of those who would be eligible for the intervention in the general or clinical population of interest? Q4, Were all eligible participants that met the pre-specified entry criteria enrolled? Q5, Was the sample size large enough to provide confidence in the findings? Q6, Was the test/intervention clearly described and delivered consistently across the study population? Q7, Were the outcome measures pre-specified, clearly defined, valid, reliable, and assessed consistently across all the study participants? Q8, Were the people assessing the outcomes blinded to the participants’ exposures/interventions? Q9, Was the loss to follow-up after baseline 20% or less? Were those lost to follow-up accounted for in the analysis? Q10, Did the statistical methods examine changes in outcome measures from before to after the intervention? Were statistical tests done that provided P values for the pre-post changes? Q11, Were outcome measures of interest taken multiple times before the intervention and multiple times after the intervention (i.e., did they use an interrupted time-series design)? Q12, If the intervention was conducted at a group level, did the statistical analysis take into account the use of individual-level data to determine effects at the group level? Y, yes; N, no; NA, not applicable.
Effects of PR on exercise capacity
Of the selected studies, 18 studies (15,16,18-22,24-26,28-35) with 33 arms (1,305 participants) reported 6MWD at the baseline and after PR. The mean 6MWD at the baseline in the participants undergoing exercise training in 14 studies was 339.6 m (range, 149.4–540.4 m), as one reported the percentage of 6MWD (31). Pooled analysis of these 18 studies revealed a significant degree of heterogeneity (I2=85.5%, P<0.001). Based on the heterogeneity results, random-effects analysis was performed, and it showed that PR had a strong correlation with improvement in 6MWD (SMD =1.28, 95% CI: 1.05–1.50, P<0.001) (Figure 2). Besides, the observed pooled effect size remained consistent according to the sensitivity analyses (Figure 3). Meta-regression analysis showed that both durations of PR (P<0.001) and intervals between LTx and PR (P<0.001) were responsible for the heterogeneity.
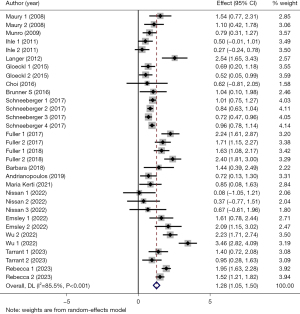
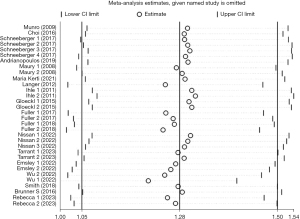
Then, we conducted subgroup analyses based on intervention duration (subgroup 1: duration <4 weeks, subgroup 2: 4≤ duration <8 weeks, subgroup 3: 8≤ duration <12 weeks and subgroup 4: duration ≥12 weeks), interval between LTx and PR (subgroup 1: interval <1 month, subgroup 2: 1≤ interval <12 months and subgroup 3: interval ≥12 months). The results of the former subgroup analyses showed a larger positive effect on 6MWD in subgroup 2 (SMD =1.46, 95% CI: 1.14–1.79, P<0.001), subgroup 3 (SMD =1.12, 95% CI: 0.67–1.57, P<0.001) and subgroup 4 (SMD =1.30, 95% CI: 0.87–1.73, P<0.001), while subgroup 1 presented small effect (SMD =0.53, 95% CI: 0.24–0.82, P=0.002) (Figure 4). In terms of heterogeneity, both subgroup 1 and 3 showed a considerable level of homogeneity (subgroup 1: I2=0.0%, P=0.46; subgroup 3: I2=0.0%, P=0.50), and between-studies heterogeneity in subgroup 4 also decreased to some extend (subgroup 4: I2=65.2%, P=0.002), whereas, subgroup 2 showed a considerable level of heterogeneity (subgroup 2: I2=91.6%, P<0.001). Besides, the latter subgroup analyses revealed that there was greater positive effect on 6MWD in subgroup 1 (SMD =1.71, 95% CI: 1.38–2.03, P<0.001) and subgroup 2 (SMD =0.93, 95% CI: 0.74–1.11, P<0.001), and small effect in subgroup 3 (SMD =0.38, 95% CI: 0.06–0.70, P=0.02) (Figure 5). The between-studies heterogeneity in three subgroups showed varying degrees of decrease (subgroup 1: I2=80.9%, P<0.001; subgroup 2: I2=56.6%, P=0.01; subgroup 3: I2=0.0%, P=0.93).
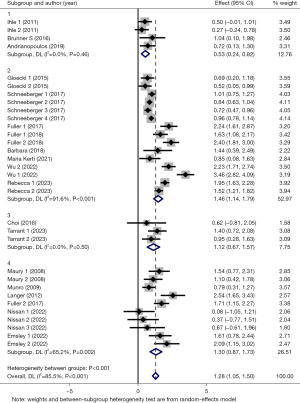
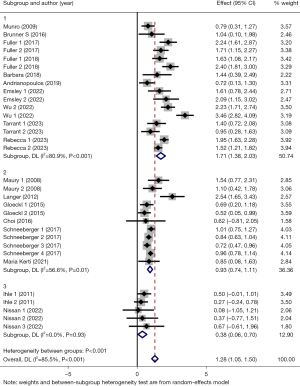
Four studies (23,27,31,32) with 5 arms (110 participants) reported VO2max at the baseline and after PR. The pooled analysis of these 4 studies showed that PR may had small effect on VO2max in adult LTx recipients (SMD =0.42, 95% CI: 0.15–0.68, P=0.002) (Figure 6). There was no significant between-studies heterogeneity (I2=0.0%, P=0.85).
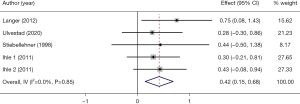
In addition to the studies mentioned above, we still included 1 study (17 participants) which evaluated the effect of PR on exercise capacity by incremental shuttle walk test (ISWT) (17), and the result also showed a positive effect. We did not perform pooled analyses because of the small number of studies.
Effects of PR on muscle strength
The effect of PR on HGF was assessed in detail in 6 studies with 7 arms (148 participants) (16,17,22,24,27,31), which showed good homogeneity (I2=0.0%, P=0.86). Based on the homogeneity, pooled fixed-effects analysis was conducted on the 6 studies, and the results demonstrated a small effect in handgrip (SMD =0.49, 95% CI: 0.26–0.73, P<0.001) (Figure 7).
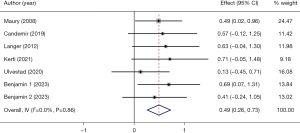
Seven studies with 10 arms (238 participants) reported the effect of PR on QF (17,22,27,29-31,34), which showed good homogeneity (I2=0.0%, P=0.88). Based on the homogeneity, pooled fixed-effects analysis was conducted on the 7 studies, and the results demonstrated a moderate effect in QF (SMD =0.63, 95% CI: 0.45–0.82, P<0.001) (Figure 8).
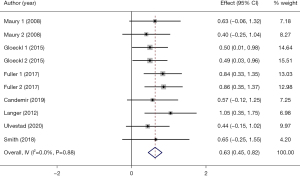
Publication bias
In terms of publication bias, the Egger’s test did not show any significant publication bias in 6MWD (P=0.14), VO2max (P=0.50), QF (P=0.76) and HGF (P=0.38).
Discussion
The principal finding of this single-arm meta-analysis is that PR is closely related with a significant improvement in exercise capacity (based on improvements in 6MWD, VO2max) and muscle strength (based on HGF, QF) from baseline to follow-up in LTx recipients. Although more than half of included studies were quasi-experimental design, the greatest between-studies heterogeneity may be associated with the difference in duration of PR and interval between LTx and PR. This meta-analysis makes an important contribution to the literature on this topic and has strong clinical implications, as the findings show that there is evidence of the efficacy of PR in LTx recipients and highlight its potential role as an adjunct to clinical approaches designed to improve survival.
6MWD and VO2max are the main indicators of exercise capacity, which showed significant improvements with PR. Previous study has established an association between 6MWD and survival in patients after LTx (36). Moreover, improvement in physical capacity is known to have a positive correlation with better prognosis (36,37). As a result, improvement in 6MWD and VO2max has been used as a surrogate endpoint in LTx clinical trials. However, most of the included studies that assessed exercise capacity were quasi-experimental studies, so it is difficult to draw a definitive conclusion on whether PR can induce a clinically viable increase in exercise capacity that can be distinguished from natural recovery. More notably, subgroup analyses on 6MWD seemed to suggest that the earlier LTx recipients undertake adequate PR, the better the exercise capacity will be. In the future, more studies are needed to confirm the effect of PR on exercise capacity in LTx recipients.
Our study also reported an improvement in HGF and QF, which is consistent with the findings of a previous study (5). Delayed recovery of exercise capacity is related to the slow recovery of muscle (38), and LTx recipients who do not receive PR are expected to experience a reduction in muscle strength due to peripheral myopathy caused by long-term drug treatment (39). Despite these optimistic findings, the clinical relevance needs to be confirmed through high-quality RCTs in the future.
LTx recipients exhibit deficiencies in muscle mitochondrial function and peripheral circulatory factors as a result of deconditioning and the impact of immunosuppressive therapy (40). Although the mechanisms underlying improvement in exercise capacity with PR among patients after LTx are not well understood. The benefits of PR are likely associated with improving these deficiencies. With regard to the influential factors, peripheral muscle abnormalities have been shown to be the predominant limiting factor to exercise capacity in LTx recipients; in addition, the original lung disease and pathophysiology may also influence an individual’s exercise capacity and physical activity (39). Recent studies have shown that exercise contributes to significant improvements in central pulmonary perfusion and peripheral skeletal muscle function that are beneficial for improvement in exercise capacity. This is probably because improved pulmonary perfusion is likely to lead to improved oxygenation and cardiac output and, thus, improvement in exercise tolerance and cardiorespiratory fitness. Moreover, Memme et al. (41) reported that exercise could help adjust the volume, structure, and capacity of mitochondria; thus, PR may help alleviate the muscle atrophy and damage to the respiratory function of mitochondria resulting from long-term immobilization before and after LTx and the use of immunosuppressive drugs. To summarize, the mechanisms underlying the beneficial effects of PR may include improvement in pulmonary effusion, peripheral skeletal muscle function, and mitochondrial structure and function. In the future, these mechanisms need to be investigated in detail in order to gain a better understanding of the effects and treatment targets.
Study limitations
There are several limitations in this study which should be noted. First, we only included studies that were published in English, which may cause language bias. Besides, we cannot eliminate a selection bias because the studies included were only retrieved from published trials. Second, significant heterogeneity may be associated with original disease, simple size, the features of the PR regimen (such as frequency, intensity, and duration of exercise), type of LTx. Although the results of this meta-analysis suggested that different PR durations and different intervals between LTx and PR may have distinct effects on 6MWD in LTx recipients, in the future, if necessary, network meta-analysis is still needed to explore for more optimized PR programs.
Conclusions
The current evidence from the literature indicates that PR is associated with significant improvement in exercise capacity among adult patients with LTx. Future high-quality studies are needed to determine whether exercise training can safely and effectively improve long-term clinical outcomes among these patients in the real-world setting.
Acknowledgments
We thank the staff of the Department of Rehabilitation Medicine and Department of Lung Transplantation in China-Japan Friendship Hospital for their assistance.
Funding: This work was supported by
Footnote
Reporting Checklist: The authors have completed the PRISMA reporting checklist. Available at https://jtd.amegroups.com/article/view/10.21037/jtd-24-568/rc
Peer Review File: Available at https://jtd.amegroups.com/article/view/10.21037/jtd-24-568/prf
Conflicts of Interest: All authors have completed the ICMJE uniform disclosure form (available at https://jtd.amegroups.com/article/view/10.21037/jtd-24-568/coif). S.J. reports funding from the China’s National Key R&D Programmes (2022YFC2009700). W.C. reports funding from the Chinese Academy of Medical Sciences (CAMS) Innovation Fund for Medical Sciences (2021-12M-1-049). The other authors have no conflicts of interest to declare.
Ethical Statement: The authors are accountable for all aspects of the work in ensuring that questions related to the accuracy or integrity of any part of the work are appropriately investigated and resolved.
Open Access Statement: This is an Open Access article distributed in accordance with the Creative Commons Attribution-NonCommercial-NoDerivs 4.0 International License (CC BY-NC-ND 4.0), which permits the non-commercial replication and distribution of the article with the strict proviso that no changes or edits are made and the original work is properly cited (including links to both the formal publication through the relevant DOI and the license). See: https://creativecommons.org/licenses/by-nc-nd/4.0/.
References
- Iguidbashian J, Cotton J, King RW, et al. Survival following lung transplantation: A population-based nested case-control study. J Card Surg 2022;37:1153-60. [Crossref] [PubMed]
- Bos S, Vos R, Van Raemdonck DE, et al. Survival in adult lung transplantation: where are we in 2020? Curr Opin Organ Transplant 2020;25:268-73. [Crossref] [PubMed]
- Bourgeois N, Shallwani SM, Al-Huda FS, et al. Relationship of Exercise Capacity, Physical Function, and Frailty Measures With Clinical Outcomes and Healthcare Utilization in Lung Transplantation: A Scoping Review. Transplant Direct 2022;8:e1385. [Crossref] [PubMed]
- Bobos P, Nazari G, Lu Z, et al. Measurement Properties of the Hand Grip Strength Assessment: A Systematic Review With Meta-analysis. Arch Phys Med Rehabil 2020;101:553-65. [Crossref] [PubMed]
- Polastri M, Dell'Amore A, Reed RM, et al. Handgrip Strength in Lung Transplant Candidates and Recipients. Exp Clin Transplant 2023;21:547-55. [PubMed]
- Rochester CL, Vogiatzis I, Holland AE, et al. An Official American Thoracic Society/European Respiratory Society Policy Statement: Enhancing Implementation, Use, and Delivery of Pulmonary Rehabilitation. Am J Respir Crit Care Med 2015;192:1373-86. [Crossref] [PubMed]
- Schneeberger T, Abdullayev G, Koczulla AR. Pulmonary Rehabilitation. Rehabilitation (Stuttg) 2023;62:232-47. [Crossref] [PubMed]
- Squires RW, Bonikowske AR. Cardiac rehabilitation for heart transplant patients: Considerations for exercise training. Prog Cardiovasc Dis 2022;70:40-8. [Crossref] [PubMed]
- Wilkinson TJ, McAdams-DeMarco M, Bennett PN, et al. Advances in exercise therapy in predialysis chronic kidney disease, hemodialysis, peritoneal dialysis, and kidney transplantation. Curr Opin Nephrol Hypertens 2020;29:471-9. [Crossref] [PubMed]
- Abidi Y, Kovats Z, Bohacs A, et al. Lung Transplant Rehabilitation-A Review. Life (Basel) 2023;13:506. [Crossref] [PubMed]
- Hume E, Ward L, Wilkinson M, et al. Exercise training for lung transplant candidates and recipients: a systematic review. Eur Respir Rev 2020;29:200053. [Crossref] [PubMed]
- Gutierrez-Arias R, Martinez-Zapata MJ, Gaete-Mahn MC, et al. Exercise training for adult lung transplant recipients. Cochrane Database Syst Rev 2021;7:CD012307. [PubMed]
- Moher D, Liberati A, Tetzlaff J, et al. Preferred reporting items for systematic reviews and meta-analyses: the PRISMA Statement. Open Med 2009;3:e123-30. [PubMed]
- Lin L, Aloe AM. Evaluation of various estimators for standardized mean difference in meta-analysis. Stat Med 2021;40:403-26. [Crossref] [PubMed]
- Emsley C, Snell G, Paul E, et al. Can we HALT obesity following lung transplant? A Dietitian- and Physiotherapy-directed pilot intervention. Clin Transplant 2022;36:e14763. [Crossref] [PubMed]
- Kerti M, Bohacs A, Madurka I, et al. The effectiveness of pulmonary rehabilitation in connection with lung transplantation in Hungary. Ann Palliat Med 2021;10:3906-15. [Crossref] [PubMed]
- Candemir I, Ergun P, Kaymaz D, et al. The Efficacy of Outpatient Pulmonary Rehabilitation After Bilateral Lung Transplantation. J Cardiopulm Rehabil Prev 2019;39:E7-E12. [Crossref] [PubMed]
- Andrianopoulos V, Gloeckl R, Boensch M, et al. Improvements in functional and cognitive status following short-term pulmonary rehabilitation in COPD lung transplant recipients: a pilot study. ERJ Open Res 2019;5:00060-2019. [Crossref] [PubMed]
- Schneeberger T, Gloeckl R, Welte T, et al. Pulmonary Rehabilitation Outcomes after Single or Double Lung Transplantation in Patients with Chronic Obstructive Pulmonary Disease or Interstitial Lung Disease. Respiration 2017;94:178-85. [Crossref] [PubMed]
- Choi J, Hergenroeder AL, Burke L, et al. Delivering an in-Home Exercise Program via Telerehabilitation: A Pilot Study of Lung Transplant Go (LTGO). Int J Telerehabil 2016;8:15-26. [Crossref] [PubMed]
- Munro PE, Holland AE, Bailey M, et al. Pulmonary rehabilitation following lung transplantation. Transplant Proc 2009;41:292-5. [Crossref] [PubMed]
- Maury G, Langer D, Verleden G, et al. Skeletal muscle force and functional exercise tolerance before and after lung transplantation: a cohort study. Am J Transplant 2008;8:1275-81. [Crossref] [PubMed]
- Stiebellehner L, Quittan M, End A, et al. Aerobic endurance training program improves exercise performance in lung transplant recipients. Chest 1998;113:906-12. [Crossref] [PubMed]
- Tarrant BJ, Quinn E, Robinson R, et al. Post-operative, inpatient rehabilitation after lung transplant evaluation (PIRATE): A feasibility randomized controlled trial. Physiother Theory Pract 2023;39:1406-16. [Crossref] [PubMed]
- Wu T, Zhou S, Wu B, et al. The effect of early tracheal extubation combined with physical training on pulmonary rehabilitation of patients after lung transplantation: a randomized controlled trial. J Thorac Dis 2022;14:1120-9. [Crossref] [PubMed]
- Nissan G, Ruth D, Paltiel W, et al. Pulmonary rehabilitation and inspiratory muscle training for patients following lung transplantation: A pilot study. Physiotherapy Practice and Research 2022;43:27-35. [Crossref]
- Ulvestad M, Durheim MT, Kongerud JS, et al. Effect of high-intensity training on peak oxygen uptake and muscular strength after lung transplantation: A randomized controlled trial. J Heart Lung Transplant 2020;39:859-67. [Crossref] [PubMed]
- Fuller LM, El-Ansary D, Button BM, et al. Effect of Upper Limb Rehabilitation Compared to No Upper Limb Rehabilitation in Lung Transplant Recipients: A Randomized Controlled Trial. Arch Phys Med Rehabil 2018;99:1257-1264.e2. [Crossref] [PubMed]
- Fuller LM, Button B, Tarrant B, et al. Longer Versus Shorter Duration of Supervised Rehabilitation After Lung Transplantation: A Randomized Trial. Arch Phys Med Rehabil 2017;98:220-226.e3. [Crossref] [PubMed]
- Gloeckl R, Heinzelmann I, Seeberg S, et al. Effects of complementary whole-body vibration training in patients after lung transplantation: A randomized, controlled trial. J Heart Lung Transplant 2015;34:1455-61. [Crossref] [PubMed]
- Langer D, Burtin C, Schepers L, et al. Exercise training after lung transplantation improves participation in daily activity: a randomized controlled trial. Am J Transplant 2012;12:1584-92. [Crossref] [PubMed]
- Ihle F, Neurohr C, Huppmann P, et al. Effect of inpatient rehabilitation on quality of life and exercise capacity in long-term lung transplant survivors: a prospective, randomized study. J Heart Lung Transplant 2011;30:912-9. [Crossref] [PubMed]
- Brunner S, Brunner D, Winter H, et al. Feasibility of whole-body vibration as an early inpatient rehabilitation tool after lung transplantation--a pilot study. Clin Transplant 2016;30:93-8. [Crossref] [PubMed]
- Smith BK, Carter N, Arteaga A, et al. Acute and 6-Month Outcomes in Recipients of Lung Transplantation Following Lower Extremity Strength Training: Pilot Study. Journal of Acute Care Physical Therapy 2018;9:145-54. [Crossref]
- Byrd R, Breslin R, Wang P, et al. Group Versus Individual Rehabilitation in Lung Transplantation: A Retrospective Noninferiority Assessment. Cardiopulmonary Physical Therapy Journal 2024;35:28-36.
- Castleberry AW, Englum BR, Snyder LD, et al. The utility of preoperative six-minute-walk distance in lung transplantation. Am J Respir Crit Care Med 2015;192:843-52. [Crossref] [PubMed]
- Saez-Gimenez B, Barrecheguren M, Ramon MA, et al. Near-normal aerobic capacity in long-term survivors after lung transplantation. ERJ Open Res 2021;7:00381-2020. [Crossref] [PubMed]
- Troosters T, Janssens W, Demeyer H, et al. Pulmonary rehabilitation and physical interventions. Eur Respir Rev 2023;32:220222. [Crossref] [PubMed]
- Hatt K, Kinback NC, Shah A, et al. A Review of Lung Transplantation and Its Implications for the Acute Inpatient Rehabilitation Team. PM R 2017;9:294-305. [Crossref] [PubMed]
- Thaniyavarn T, Grewal HS, Goldberg HJ, et al. Nonallograft Complications of Lung Transplantation. Thorac Surg Clin 2022;32:243-58. [Crossref] [PubMed]
- Memme JM, Erlich AT, Phukan G, et al. Exercise and mitochondrial health. J Physiol 2021;599:803-17. [Crossref] [PubMed]