Enhancing outcomes in extensive-stage small cell lung cancer brain metastases: a retrospective study on the synergistic effects of immune checkpoint inhibitor, brain radiotherapy, and chemotherapy
Highlight box
Key findings
• For extensive-stage small cell lung cancer (ES-SCLC) patients with synchronous brain metastases, the combination of immune checkpoint inhibitor (ICI) and brain radiotherapy (BRT), alongside chemotherapy, surpasses the efficacy of either treatment alone, with manageable treatment-related adverse events (TRAEs).
• Multivariate analysis identified that ICIs in combination with various radiotherapy regimens [whole BRT (WBRT), WBRT with boost] effectively influenced overall survival (OS).
What is known, and what is new?
• Combining chemotherapy with immunotherapy for ES-SCLC has substantial benefits, leading the National Comprehensive Cancer Network (NCCN) to recommend this strategy as the first-line treatment. However, the optimal approach for those with brain metastases remains ambiguous. This study suggests that combined ICI, BRT, and chemotherapy should be used as first-line treatment for ES-SCLC patients with synchronous brain metastases. The recommended radiotherapy regimens are WBRT and WBRT with boost.
What is the implication, and what should change now?
• This study suggests that combined ICI, BRT, and chemotherapy can significantly improve the prognosis of patients. Our findings also suggest that WBRT and WBRT + boost are independent prognostic factors of small cell lung cancer patients with brain metastases. An ongoing “single-arm, single-center phase II clinical study evaluating the efficacy of WBRT combined with tislelizumab and chemotherapy in patients with small-cell lung cancer harboring brain metastases”, is currently in the enrollment phase.
Introduction
Lung cancer continues to be a major health challenge globally, maintaining high incidence and mortality rates. Among its types, small cell lung cancer (SCLC) represents approximately 15% of cases (1), distinguished by its aggressive nature and swift progression. At initial diagnosis, a significant portion of SCLC patients (67.4%) are found to be in the extensive stage (ES-SCLC) (2), which is associated with a median overall survival (mOS) of around ten months (3). Brain metastases are a critical concern for these patients, with about 20% developing brain metastases during their illness (4). At diagnosis, around 10% of patients already exhibit brain metastases (5), significantly worsening their prognosis, with the survival span of untreated individuals dropping to under three months (4).
Studies (6-8) have highlighted the substantial benefits of combining chemotherapy with immunotherapy for ES-SCLC, leading the National Comprehensive Cancer Network (NCCN) to recommend this strategy as the first-line treatment. Nonetheless, the optimal approach for those with brain metastases remains ambiguous. While KEYNOTE-158 and KEYNOTE-028 have shown some success in treating recurrent or metastatic SCLC with pembrolizumab, including a 15.4% objective response rate (ORR) in brain metastases patients (9), other major studies such as KEYNOTE-604 and Impower133 did not demonstrate significant benefits for brain metastases patients (3,10). These mixed outcomes underscore the ongoing debate over the effectiveness of integrating chemotherapy with immunotherapy in ES-SCLC patients with brain metastases.
Brain radiotherapy (BRT), encompassing whole BRT (WBRT), WBRT with a simultaneous integrated boost (WBRT + boost), and stereotactic radiotherapy (SRT), is a foundational approach in managing brain metastases. Historically, the amalgamation of BRT and chemotherapy has been the conventional regimen for treating SCLC brain metastases, achieving a mOS of roughly seven months but also encountering certain limitations (11).
Although integrating BRT and chemotherapy with immunotherapy has become widespread in treating NSCLC brain metastases (12-14), the effectiveness and safety of such therapeutic combinations for SCLC brain metastases remain contested. This study seeks to evaluate the comparative effectiveness and side effects of BRT alone, immunotherapy alone, and their conjunction with chemotherapy in treating patients with extensive-stage SCLC (ES-SCLC) who have synchronous brain metastases. It also aims to investigate prognostic factors within this patient cohort. We present this article in accordance with the STROBE reporting checklist (available at https://jtd.amegroups.com/article/view/10.21037/jtd-24-654/rc).
Methods
Patient selection
This retrospective analysis reviewed the clinical data of patients diagnosed with brain metastases from SCLC at Zhejiang Cancer Hospital between January 2017 and October 2023. The inclusion criteria were as follows: (I) pathologically confirmed diagnosis of SCLC; (II) presence of brain metastases verifiable by computed tomography (CT) or magnetic resonance imaging (MRI); (III) either symptomatic or asymptomatic brain metastases, with symptoms potentially including headache, vertigo, nausea, vomiting, and seizures; (IV) brain metastases identified at the time of initial diagnosis (i.e., synchronous brain metastases); (V) availability of complete clinical data; (VI) a Karnofsky Performance Status (KPS) score of 60 or above.
Patients were excluded based on the following criteria: (I) any treatment for SCLC prior to the diagnosis of brain metastases; (II) diagnosis with other types of malignant tumors alongside SCLC at the time of initial diagnosis; (III) presence of active or uncontrollable serious infections; (IV) previous immunotherapy treatments; (V) incomplete clinical data.
Data collection
The study compiled extensive patient information covering demographic characteristics, clinical history, and responses to treatment. This data encompassed age, gender, smoking status, and the presence of comorbid conditions such as diabetes and hypertension. Initial evaluations included the KPS score and the detection of extracranial distant metastases, specifically in the liver, bone, and adrenal glands. The number of brain metastases, associated symptoms, and whether the first-line treatment included extracranial palliative radiotherapy were documented. Further data collection entailed any history of neurosurgical interventions, the application of anti-angiogenic targeted therapies, and specifics of chemotherapy and immunotherapy regimens, encompassing the number of cycles administered during first-line treatment. Additionally, the study investigated the use of combined immunotherapy and targeted therapy from the second line of treatment onward.
Documented adverse events during treatment included hematotoxicity, hepatotoxicity, nephrotoxicity, thyroid dysfunction, abnormal cardiac markers, and gastrointestinal side effects. Information regarding the specific regimen and dosage of BRT used in first-line treatment, the number of brain metastases targeted, and the grading of acute radiation injury according to the Radiation Therapy Oncology Group (RTOG) criteria for the central nervous system was also gathered.
The study was conducted per the Declaration of Helsinki (revised in 2013). The study was approved by the Medical Ethics Committee of Zhejiang Cancer Hospital [No. IRB-2024-324 (IIT)], and individual consent for this retrospective analysis was waived.
Treatment modalities
The study categorized 187 patients with ES-SCLC brain metastases into three groups based on their initial treatment regimens: the BRT group (n=83), the immune checkpoint inhibitor (ICI) group (n=56), and the combined ICI + BRT group (n=48). The BRT group received a combination of BRT and chemotherapy as their first-line treatment. The ICI group underwent a chemotherapy regimen paired with ICIs, which aligned with the current National Comprehensive Cancer Network (NCCN) Guidelines for ES-SCLC. The combined treatment group (ICI + BRT) was administered a triple regimen of chemotherapy, ICIs, and BRT, encompassing WBRT, WBRT + boost, and SRT, with a standard BRT dose of 30 Gy across ten fractions. This dosage could be adjusted based on the patient’s health status and the specific needs of the disease.
The chemotherapy protocol primarily involved etoposide (100 mg/m2 administered intravenously on days 1 to 3, every 3 weeks) combined with either cisplatin (75 mg/m2 administered intravenously on day 1) or carboplatin [area under the curve (AUC) 4–5 administered intravenously on day 1, every 3 weeks]. The ICIs employed in both the ICI and ICI + BRT groups fell into four main categories: durvalumab (1,500 mg, every three weeks), atezolizumab (200 mg, every three weeks), tislelizumab (200 mg, every three weeks), serplulimab (300 mg, every three weeks), along with other programmed cell death-1 (PD-1) inhibitors. Modifications to the type and dosage of these drugs were made as necessary based on the progression of the disease and the patient’s tolerance.
Furthermore, the ICI + BRT group was stratified into those receiving synchronized treatment (n=27), where the interval between ICI and BRT was four weeks or less, and the non-synchronized treatment group (n=21), with an interval exceeding four weeks.
Study endpoints
The primary endpoint of this study was overall survival (OS), with progression-free survival (PFS) and intracranial PFS (iPFS) as secondary endpoints. OS is defined by the time from initial diagnosis to death from any cause or the date of last known follow-up. PFS measures the time from the start of treatment to tumor progression, death from any cause, or the last recorded follow-up, assessing the treatment’s efficacy in delaying disease progression. Conversely, iPFS is determined from when brain metastasis is diagnosed to the first instance of intracranial disease progression, death for any reason, or the last follow-up, specifically evaluating control over the intracranial disease. The study’s data were concluded on February 20, 2024, establishing the cut-off date for analysis.
Efficacy and toxicity evaluation criteria
In our study, the efficacy of systemic tumor treatments was assessed using the Response Evaluation Criteria in Solid Tumors (RECIST) version 1.1, whereas intracranial lesions were evaluated based on the Response Assessment in Neuro-Oncology (RANO) criteria. Following treatment initiation, evaluations were conducted routinely every 6–8 weeks, and subsequent assessments took place every 3–6 months post-treatment. The ORR was determined by the percentage of patients achieving either a complete response (CR) or a partial response (PR). Similarly, the disease control rate (DCR) included patients with CR, PR, and stable disease (SD).
For intracranial outcomes, intracranial ORR (iORR) and intracranial DCR (iDCR) were calculated analogously to ORR and DCR, focusing on the responses within the brain. The assessment of treatment-related adverse reactions (TRAEs) adhered to the National Cancer Institute Common Terminology Criteria for Adverse Events (NCI-CTC) version 5.0. The responsible physician immediately documented and graded TRAEs identified during hospital stays. In contrast, those TRAEs reported post-discharge were primarily collected through patient self-reports and telephone follow-ups.
Furthermore, the neurotoxic effects of BRT were evaluated per the RTOG Acute Radiation Injury Grading Criteria for the Central Nervous System (15). Comparative analyses involved the utilization of CT and MRI scans of the brain, as required, to precisely gauge the extent of neurotoxicity experienced by the patient.
Statistical analysis
This study employed SPSS statistical software, version 29.0, for all statistical analyses. Baseline demographics, treatment modalities, tumor responses, and adverse events across different groups were compared using the Chi-squared test, the corrected Chi-squared test, and Fisher’s exact test, as warranted. Kaplan-Meier survival plots were created to delineate OS, PFS, and iPFS among the groups. The log-rank test was utilized to assess differences in survival curves between these groups.
An initial univariate survival analysis using the Cox proportional hazards model was conducted to explore the association between OS and vital clinical factors. Factors that showed significance (P<0.05) in the univariate analysis were then included in a multivariate Cox proportional hazards model to identify significant predictors.
A two-tailed P value of <0.05 was considered indicative of statistical significance. In instances where significant differences emerged from the tri-group comparison, further analysis that contrasted the ICI + BRT group against the BRT and ICI groups individually was performed, applying an adjusted significance threshold of P≤0.025 to account for multiple comparisons (alpha splitting method).
Results
Patient demographics and characteristics
Of 3,674 lung cancer patients diagnosed with brain metastases at Zhejiang Cancer Hospital from January 2017 to October 2023, 187 qualified for inclusion based on our selection criteria. Patient exclusions are illustrated in Figure 1. These selected patients were divided into three treatment cohorts: 83 in the BRT group, 56 in the ICI group, and 48 in the combined ICI + BRT group. The median follow-up period was 13 months. Detailed baseline characteristics are summarized in Table 1, indicating an average age of 62.98±7.836 years at diagnosis, with a median age of 64. The cohort was predominantly male, making up over 90% of the participants. Most patients had a KPS score of 80 or above, and nearly half exhibited neurological symptoms at diagnosis. A significant number, 121 patients (64.7%), presented with brain metastases alongside extracranial metastases, with liver and bone metastases being particularly common. Over 80% of the patients had a GPA score of 2 or less.
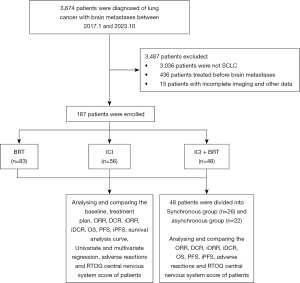
Table 1
Demographic and clinical characteristics | Total number of cases (n=187) | BRT group (n=83) |
ICI group (n=56) |
ICI + BRT group (n=48) |
χ2 value | P |
---|---|---|---|---|---|---|
Age (years) | 2.917 | 0.23 | ||||
Median (P25, P75) (range) | 64.0 (58.0, 69.0) (36.0–80.0) | 62.0 (54.0, 69.0) (45.0–80.0) | 66.5 (61.3, 70.0) (48.0–79.0) | 64.0 (59.0, 68.8) (36.0–74.0) | ||
≥60 | 131 (70.1) | 53 (63.9) | 43 (76.8) | 35 (72.9) | ||
<60 | 56 (29.9) | 30 (36.1) | 13 (23.2) | 13 (27.1) | ||
Gender | – | 0.90 | ||||
Male | 179 (95.7) | 80 (96.4) | 53 (94.6) | 46 (95.8) | ||
Female | 8 (4.3) | 3 (3.6) | 3 (5.4) | 2 (4.2) | ||
KPS score | 0.804 | 0.67 | ||||
≥80 | 111 (59.4) | 47 (56.6) | 33 (58.9) | 31 (64.6) | ||
<80 | 76 (40.6) | 36 (43.4) | 23 (41.1) | 17 (35.4) | ||
Smoking history | 0.853 | 0.65 | ||||
Ever | 130 (69.5) | 60 (72.3) | 39 (69.6) | 31 (64.6) | ||
Never | 57 (30.5) | 23 (27.7) | 17 (30.4) | 17 (35.4) | ||
Number of brain metastases | 1.724 | 0.42 | ||||
≥3 | 90 (48.1) | 38 (45.8) | 25 (44.6) | 27 (56.3) | ||
<3 | 97 (51.9) | 45 (54.2) | 31 (55.4) | 21 (43.7) | ||
Brain metastasis symptoms | 2.798 | 0.25 | ||||
Symptomatic | 92 (49.2) | 46 (55.4) | 23 (41.1) | 23 (47.9) | ||
Asymptomatic | 95 (50.8) | 37 (44.6) | 33 (58.9) | 25 (52.1) | ||
Underlying diseases | 3.114 | 0.21 | ||||
Yes | 86 (46.0) | 37 (44.6) | 22 (39.3) | 27 (56.3) | ||
No | 101 (54.0) | 46 (55.4) | 34 (60.7) | 21 (43.7) | ||
Extracranial distant metastases | 3.838 | 0.15 | ||||
Yes | 121 (64.7) | 51 (61.4) | 42 (75.0) | 28 (58.3) | ||
No | 66 (35.3) | 32 (38.6) | 14 (25.0) | 20 (41.7) | ||
Liver metastases | 2.709 | 0.26 | ||||
Yes | 60 (32.1) | 28 (33.7) | 21 (37.5) | 11 (22.9) | ||
No | 127 (67.9) | 55 (66.3) | 35 (62.5) | 37 (77.1) | ||
Bone metastases | 3.762 | 0.15 | ||||
Yes | 52 (27.8) | 20 (24.1) | 21 (37.5) | 11 (22.9) | ||
No | 135 (72.2) | 63 (75.9) | 35 (62.5) | 37 (77.1) | ||
Adrenal metastases | 0.292 | 0.86 | ||||
Yes | 44 (23.5) | 21 (25.3) | 12 (21.4) | 11 (22.9) | ||
No | 143 (76.5) | 62 (74.7) | 44 (78.6) | 37 (77.1) | ||
GPA score | 0.059 | 0.97 | ||||
>2 | 37 (19.8) | 17 (20.5) | 11 (19.6) | 9 (18.8) | ||
≤2 | 150 (80.2) | 66 (79.5) | 45 (80.4) | 39 (81.2) |
Statistical significance was set at P<0.05. For certain variables, Fisher’s exact probability method was applied in the absence of χ2 values. Data are presented as n (%) unless otherwise stated. BRT, brain radiotherapy; ICI, immune checkpoint inhibitor; KPS, Karnofsky Performance Status; GPA, Graded Prognostic Assessment score.
Treatment regimens and details
Table 2 presents the specifics of the treatment regimens. The chemotherapy combination of etoposide and carboplatin was administered to 99 patients (52.9%), cisplatin to 75 patients (40.2%), and other regimens to 13 patients (6.9%). A majority, 140 patients (74.9%), underwent four or more chemotherapy cycles. WBRT was the predominant BRT approach, typically delivered at a dosage of 30 Gy over 10 fractions. Among those receiving immunotherapy, 31 patients (29.9%) were treated with serplulimab, 28 (26.9%) with durvalumab, 17 (16.3%) with atezolizumab, and 15 (14.4%) with tislelizumab. Concurrent extracranial radiotherapy was administered as part of the first-line treatment to 44 patients (23.5%). Post-first-line progression, 23 patients (12.3%) received a combination of anti-angiogenic targeted therapy and immunotherapy. Neurosurgical resection of brain metastases at the initiation of the first-line therapy was performed in 6 patients (3.2%), and 8 patients (4.3%) underwent synchronized anti-angiogenic targeted therapy.
Table 2
Treatment-related characteristics | Total cases, n (%) | BRT group (n=83) |
ICI group (n=56) |
ICI + BRT group (n=48) | χ2 value | P |
---|---|---|---|---|---|---|
BRT methods | 0.063 | 0.97 | ||||
WBRT | 105 (80.2) | 67 (80.8) | – | 38 (79.2) | ||
WBRT + boost | 16 (12.2) | 10 (12.0) | – | 6 (12.5) | ||
SRT | 10 (7.6) | 6 (7.2) | – | 4 (8.3) | ||
BRT dose (Gy) | 0.000 | >0.99 | ||||
≥30 | 119 (90.8) | 75 (90.4) | – | 44 (91.7) | ||
<30 | 12 (9.2) | 8 (9.6) | – | 4 (8.3) | ||
Chemotherapy regimen | – | 0.27 | ||||
Etoposide + cisplatin | 75 (40.2) | 36 (43.4) | 21 (37.5) | 18 (37.5) | ||
Etoposide + carboplatin | 99 (52.9) | 40 (48.2) | 34 (60.7) | 25 (52.1) | ||
Etoposide + nedaplatin | 3 (1.6) | 3 (3.6) | 0 (0.0) | 0 (0.0) | ||
Other | 10 (5.3) | 4 (4.8) | 1 (1.8) | 5 (10.4) | ||
Cycles of chemotherapy | 0.636 | 0.73 | ||||
≥4 | 140 (74.9) | 61 (73.5) | 41 (73.2) | 38 (79.2) | ||
<4 | 47 (25.1) | 22 (26.5) | 15 (26.8) | 10 (20.8) | ||
Brain surgery | – | 0.12 | ||||
Yes | 6 (3.2) | 5 (6.0) | 0 (0.0) | 1 (2.1) | ||
No | 181 (96.8) | 78 (94.0) | 56 (100.0) | 47 (97.9) | ||
First-line extracranial radiotherapy | 0.822 | 0.66 | ||||
Yes | 44 (23.5) | 20 (24.1) | 11 (19.6) | 13 (27.1) | ||
No | 143 (76.5) | 63 (75.9) | 45 (80.4) | 35 (72.9) | ||
ICI treatment cycles | 10.940 | <0.001* | ||||
≥6 | 60 (57.7) | – | 24 (42.9) | 36 (75.0) | ||
<6 | 44 (42.3) | – | 32 (57.1) | 12 (25.0) | ||
Specific types of ICI | 1.649 | 0.80 | ||||
Durvalumab | 28 (26.9) | – | 15 (26.8) | 13 (27.1) | ||
Atezolizumab | 17 (16.3) | – | 10 (17.8) | 7 (14.6) | ||
Tirelizumab | 15 (14.4) | – | 9 (16.1) | 6 (12.5) | ||
Serplulimab | 31 (29.9) | – | 17 (30.4) | 14 (29.1) | ||
Other PD-1 ICIs | 13 (12.5) | – | 5 (8.9) | 8 (16.7) | ||
First-line treatment antivascular targeted therapy | – | 0.33 | ||||
Yes | 8 (4.3) | 2 (2.4) | 2 (3.6) | 4 (8.3) | ||
No | 179 (95.7) | 81 (97.6) | 54 (96.4) | 44 (91.7) | ||
Second to multiple lines immunotherapy + antivascular targeted therapy | 0.975 | 0.61 | ||||
Yes | 23 (12.3) | 11 (13.3) | 8 (14.3) | 4 (8.3) | ||
No | 164 (87.7) | 72 (86.7) | 48 (85.7) | 44 (91.7) | ||
Second to multiple lines antivascular targeted therapy | 4.182 | 0.12 | ||||
Yes | 52 (27.8) | 18 (21.7) | 21 (37.5) | 13 (27.1) | ||
No | 135 (72.2) | 65 (78.3) | 35 (62.5) | 35 (72.9) |
Data are presented as n (%). *, statistical significance across the three groups (P<0.05). Fisher’s exact probability method was employed for specific variables due to the absence of χ2 values. BRT, brain radiotherapy; ICI, immune checkpoint inhibitor; PD-1, programmed death receptor 1; WBRT, whole brain radiotherapy; WBRT + boost, WBRT with simultaneous integrated boost; SRT, stereotactic radiotherapy.
Response and survival outcomes
In the analysis of survival outcomes, the mOS times for the BRT, ICI, and the combined ICI + BRT groups were 11.6, 11.6, and 20.9 months, respectively, demonstrating significant differences (P<0.001). Specifically, the OS for the BRT group versus the ICI + BRT group and the ICI group versus the ICI + BRT group showed marked improvements (both P<0.001), as depicted in the Kaplan-Meier (K-M) survival curves (Figure 2A).
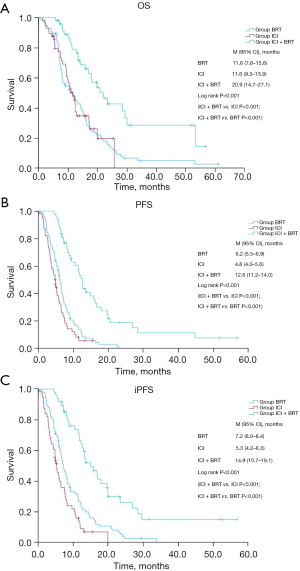
The PFS for these groups was observed at 6.2 months for BRT, 4.8 months for ICI, and 12.6 months for ICI + BRT, indicating significant enhancements in survival (P<0.001) with the combined treatment approach (both comparisons P<0.001), illustrated in Figure 2B.
Similarly, the median iPFS among the groups was 7.2, 5.3, and 14.9 months, respectively, with the ICI + BRT group showing superior outcomes (P<0.001 for all comparisons), as shown in Figure 2C.
The ORR across all 187 patients was 65.3%, with group-specific rates of 53.0% in BRT, 66.1% in ICI, and an impressive 85.4% in ICI + BRT (P<0.001), showcasing the enhanced efficacy of the combined treatment. Significant differences were also observed in the DCR, which was 82.9% overall but varied as 77.1% in BRT, 78.6% in ICI, and 97.9% in ICI + BRT, underlining the superiority of the combined regimen (P=0.006).
Intracranial outcomes reflected a total iORR of 66.3%, with the ICI + BRT group demonstrating a notable rate of 89.6% compared to 53.0% in BRT and 65.0% in ICI (P<0.001). The iDCR also highlighted the advantage of the ICI + BRT combination, reaching 97.9% compared to 83.1% in BRT and 76.8% in ICI (P=0.009), detailed in Tables 3,4.
Table 3
Healing effect | Total cases (n=187) | BRT group (n=83) | ICI group (n=56) | ICI + BRT group (n=48) | P |
---|---|---|---|---|---|
CR/PR (n, %) | 122 (65.3) | 44 (53.0) | 37 (66.1) | 41 (85.4) | – |
SD (n, %) | 33 (17.6) | 20 (24.1) | 7 (12.5) | 6 (12.5) | – |
PD (n, %) | 32 (17.1) | 19 (22.9) | 12 (21.4) | 1 (2.1) | – |
ORR (n, %) | 122 (65.3) | 44 (53.0) | 37 (66.1) | 41 (85.4) | <0.001* |
DCR (n, %) | 155 (82.9) | 64 (77.1) | 44 (78.6) | 47 (97.9) | 0.006* |
*, statistical significance, P<0.05 for comparisons across three groups and P≤0.025 for pairwise comparisons between two groups. ORR: BRT vs. ICI + BRT (P<0.001); ICI vs. ICI + BRT (P=0.02); DCR: BRT vs. ICI + BRT (P=0.001); ICI vs. ICI + BRT (P=0.003). BRT, brain radiotherapy; ICI, immune checkpoint inhibitor; CR, complete response; PR, partial response; SD, stable disease; PD, progressive disease; ORR, objective response rate; DCR, disease control rate.
Table 4
Healing effect | Total cases (n=187) | BRT group (n=83) | ICI group (n=56) | ICI + BRT group (n=48) | P |
---|---|---|---|---|---|
CR (n, %) | 28 (15.0) | 10 (12.0) | 5 (8.9) | 13 (27.1) | – |
PR (n, %) | 96 (51.3) | 44 (53.0) | 22 (39.3) | 30 (62.5) | – |
SD (n, %) | 35 (18.7) | 15 (18.1) | 16 (28.6) | 4 (8.3) | – |
PD (n, %) | 28 (15.0) | 14 (16.9) | 13 (23.2) | 1 (2.1) | – |
iORR (n, %) | 124 (66.3) | 54 (65.0) | 27 (48.2) | 43 (89.6) | <0.001* |
iDCR (n, %) | 159 (85.0) | 69 (83.1) | 43 (76.8) | 47 (97.9) | 0.009* |
*, statistical significance, P<0.05 for comparisons across three groups and P≤0.025 for pairwise comparisons between two groups. iORR: BRT vs. ICI + BRT (P=0.002); ICI vs. ICI + BRT (P<0.001); iDCR: BRT vs. ICI + BRT (P=0.01); ICI vs. ICI + BRT (P=0.002). iORR, intracranial objective response rate; iDCR, intracranial disease control rate; BRT, brain radiotherapy; ICI, immune checkpoint inhibitor; CR, complete response; PR, partial response; SD, stable disease; PD, progressive disease.
Treatment-related adverse events
The incidence and severity of first-line TRAEs are detailed in Table 5 and Table S1. The occurrence of TRAEs was remarkably high across all treatment groups, collectively reaching 98.9%. Hematologic toxicities were the most prevalent, with 92.8% in the BRT group, 91.1% in the ICI group, and 95.8% in the ICI + BRT group, demonstrating no significant variance (P=0.66). Anemia emerged as a common complication, affecting over 80% of patients in each group during the first-line therapy.
Table 5
TRAEs | Total cases (n=187) | BRT group (n=83) | ICI group (n=56) | ICI + BRT group (n=48) | χ2 value | P |
---|---|---|---|---|---|---|
Any TRAEs | 185 (98.9) | 82 (98.8) | 56 (100.0) | 47 (97.9) | – | 0.73 |
TRAEs ≥10% | ||||||
Hematologic toxicity | 174 (93.0) | 77 (92.8) | 51 (91.1) | 46 (95.8) | – | 0.66 |
Anemic | 166 (88.8) | 74 (89.2) | 49 (87.5) | 43 (89.6) | 0.135 | 0.94 |
Leucopenia | 100 (53.5) | 51 (61.4) | 21 (37.5) | 28 (58.3) | 8.319 | 0.02* |
Neutropenia | 95 (50.8) | 48 (57.8) | 18 (32.1) | 29 (60.4) | 11.217 | 0.004* |
Thrombocytopenia | 76 (40.6) | 31 (37.3) | 19 (33.9) | 26 (54.2) | 5.059 | 0.08 |
Hypoalbuminemia | 130 (69.5) | 68 (81.9) | 33 (58.9) | 29 (60.4) | 10.872 | 0.004* |
Liver dysfunction | 113 (60.4) | 52 (62.7) | 32 (57.1) | 29 (60.4) | 0.424 | 0.81 |
Increased γ-GT | 81 (43.3) | 38 (45.8) | 25 (44.6) | 18 (37.5) | 0.907 | 0.64 |
Increased AST | 53 (28.3) | 23 (27.7) | 15 (26.8) | 15 (31.3) | 0.283 | 0.87 |
Increased ALT | 49 (26.2) | 22 (26.5) | 13 (23.2) | 14 (29.2) | 0.481 | 0.79 |
Increased blood bilirubin | 33 (17.6) | 17 (20.5) | 10 (17.9) | 6 (12.5) | 1.336 | 0.51 |
Nausea and vomiting | 103 (55.1) | 50 (60.2) | 24 (42.9) | 29 (60.4) | 4.827 | 0.09 |
Fatigue | 103 (55.1) | 53 (63.9) | 29 (51.8) | 21 (43.8) | 5.319 | 0.07 |
Weight loss | 66 (35.3) | 30 (36.1) | 22 (39.3) | 14 (29.2) | 1.206 | 0.55 |
Hematuria, proteinuria | 60 (32.1) | 20 (24.1) | 24 (42.9) | 16 (33.3) | 5.447 | 0.07 |
High blood fat disease | 48 (25.7) | 23 (27.7) | 13 (23.2) | 12 (25.0) | 0.369 | 0.83 |
Abnormal thyroid function | 47 (25.1) | 8 (9.6) | 18 (32.1) | 21 (43.8) | 20.894 | <0.001* |
Myocardial enzyme abnormalities | 45 (24.1) | 15 (18.1) | 13 (23.2) | 17 (35.4) | 5.038 | 0.08 |
Creatine kinase and isoenzyme abnormalities | 26 (13.9) | 10 (12.0) | 10 (17.9) | 6 (12.5) | 1.049 | 0.59 |
Increased troponin | 20 (10.7) | 5 (6.0) | 5 (8.9) | 10 (20.8) | 7.244 | 0.03* |
Renal impairment | 27 (14.4) | 17 (20.5) | 5 (8.9) | 5 (10.4) | 4.458 | 0.11 |
Insomnia | 24 (12.8) | 14 (16.9) | 4 (7.1) | 6 (12.5) | 2.833 | 0.24 |
Abdominal pain, diarrhea, bloating | 24 (12.8) | 11 (13.3) | 6 (10.7) | 7 (14.6) | 0.369 | 0.83 |
Rash | 20 (10.7) | 5 (6.0) | 8 (14.3) | 7 (14.6) | 3.412 | 0.18 |
≥3 TRAEs ≥10% | ||||||
≥3 TRAEs | 71 (38.0) | 35 (42.2) | 13 (23.2) | 23 (47.9) | 7.815 | 0.02* |
Hematologic toxicity | 62 (33.2) | 32 (38.6) | 11 (19.6) | 19 (39.6) | 6.600 | 0.04* |
Neutropenia | 49 (26.2) | 27 (32.5) | 8 (14.3) | 14 (29.2) | 6.049 | 0.049* |
Leucopenia | 29 (15.5) | 15 (18.1) | 6 (10.7) | 8 (16.7) | 1.448 | 0.49 |
Anemic | 19 (10.2) | 10 (12.0) | 5 (8.9) | 4 (8.3) | 0.593 | 0.74 |
Data are presented as n (%). *, statistical significance (P<0.05) in three-group comparisons (P<0.05) and two-group multiple comparisons (P≤0.025). Some variables were analyzed using Fisher’s exact probability method, with no χ2 values. TRAEs, treatment-related adverse reactions; BRT, brain radiotherapy; ICI, immune checkpoint inhibitor; γ-GT, γ-glutamyl transferase; AST, aspartate aminotransferase; ALT, alanine aminotransferase.
Twenty-one patients experienced Grade 4 TRAEs, with no significant disparity observed across the three groups (P=0.17), and no cases of Grade 5 TRAEs were reported. Immunotherapy-specific TRAEs included one instance of immune ophthalmia in the ICI group, two cases of immune enteritis (one in each of two groups), and two cases of immune pneumonitis (one in each of two groups).
Notably, neutropenia of any grade occurred in 57.8% of patients in the BRT group, 32.1% in the ICI group, and 60.4% in the ICI + BRT group, with the difference being statistically significant between the ICI and ICI + BRT groups (P=0.004). Thyroid function abnormalities were reported at varying rates: 9.6% in the BRT group, 32.1% in the ICI group, and 43.8% in the ICI + BRT group, highlighting a significant increase in the combined therapy group compared to BRT alone (P<0.001).
The incidence of troponin increase was significantly higher in the ICI + BRT group compared to the BRT group (P=0.01), which conversely showed a higher probability of hypoalbuminemia (P=0.007). The probability of experiencing grade ≥3 TRAEs was 42.2% in the BRT group, 23.2% in the ICI group, and 47.9% in the ICI + BRT group (P=0.02), with a significant distinction found between the ICI and ICI + BRT groups for grade ≥3 hematologic TRAEs (P=0.025).
The evaluation of RTOG acute radiation injury to the central nervous system revealed no significant differences between the BRT and ICI + BRT groups, as indicated in Tables 6,7. Among the 131 patients receiving BRT, three were graded with an RTOG score 3, and one patient reached grade 4, underscoring the relatively consistent neurotoxic risk between the BRT and combined treatment groups.
Table 6
RTOG score | Total cases (n=131) | BRT group (n=83) | ICI + BRT group (n=48) | χ2 value | P |
---|---|---|---|---|---|
≥2 | 31 (23.7) | 22 (26.5) | 9 (18.8) | 1.013 | 0.31 |
<2 | 100 (76.3) | 61 (73.5) | 39 (81.2) |
Data are presented as n (%). P<0.05 is considered statistically significant. RTOG, Radiation Therapy Oncology Group; BRT, brain radiotherapy; ICI, immune checkpoint inhibitor.
Table 7
Grade | Explanation |
---|---|
0 | No change |
1 | Fully functional status (i.e., able to work) with minor neurological findings, no medication needed |
2 | Neurological findings present sufficient to require home care/nursing assistance may be required/medications including steroids/antiseizure agents may be required |
3 | Neurological findings requiring hospitalization for initial management |
4 | Serious neurological impairment that includes paralysis, coma, or seizures >3 per week despite medication/hospitalization required |
RTOG, Radiation Therapy Oncology Group.
Prognostic factors influencing OS
The objective of this study was to delineate the impact of various clinical parameters on the OS of patients with brain metastases from SCLC. Initially, a univariate Cox regression analysis identified potential factors affecting patient OS, summarized in Table 8. This analysis indicated that several factors, including the number of brain metastases (≥3), a KPS of ≥80, presence of extracranial distant metastases, liver and bone metastases, a GPA score of >2, specific BRT regimens, the inclusion of first-line extracranial radiotherapy, more than six cycles of immunotherapy, use of serplulimab, completion of at least four chemotherapy cycles, and the choice of first-line treatment regimen, could be associated with variations in OS among patients.
Table 8
Influencing factors (independent variables) | P | HR value | 95% CI for HR |
---|---|---|---|
Number of brain metastases (≥3/<3) | 0.007* | 1.607 | 1.140, 2.267 |
Sex (M/F) | 0.71 | 1.156 | 0.539, 2.478 |
Smoking (yes/no) | 0.44 | 1.161 | 0.795, 1.696 |
Underlying diseases (yes/no) | 0.50 | 1.124 | 0.800, 1.578 |
KPS (≥80/<80) | 0.02* | 0.661 | 0.468, 0.935 |
Age (≥60/<60 years) | 0.40 | 1.171 | 0.813, 1.687 |
Brain symptoms at diagnosis (yes/no) | 0.51 | 1.120 | 0.798, 1.571 |
Extracranial distant metastases (yes/no) | <0.001* | 1.919 | 1.329, 2.773 |
Liver metastases (yes/no) | 0.003* | 1.738 | 1.214, 2.489 |
Bone metastases (yes/no) | 0.004* | 1.704 | 1.180, 2.460 |
Adrenal metastases (yes/no) | 0.18 | 1.314 | 0.886, 1.949 |
GPA grading (>2/≤2) | <0.001* | 0.419 | 0.266, 0.662 |
Intracranial surgical treatment (yes/no) | 0.36 | 0.655 | 0.267, 1.608 |
BRT methods | |||
No BRT, dummy variable | 0.22 | ||
WBRT | 0.16 | 0.743 | 0.494, 1.118 |
WBRT + boost | 0.04* | 0.503 | 0.258, 0.981 |
SRT | 0.57 | 0.787 | 0.347, 1.784 |
First-line extracranial radiotherapy (yes/no) | 0.01* | 0.596 | 0.398, 0.894 |
First-line targeted therapy (yes/no) | 0.61 | 1.261 | 0.515, 3.090 |
Immunotherapy cycle (≥6/<6) | <0.001* | 0.422 | 0.282, 0.632 |
Immunotherapy | |||
No ICI, dummy variable | 0.11 | ||
Durvalumab | 0.07 | 0.647 | 0.402, 1.041 |
Atezolizumab | 0.08 | 0.558 | 0.288, 1.080 |
Tislelizumab | 0.23 | 0.639 | 0.307, 1.330 |
Serplulimab | 0.043* | 0.517 | 0.273, 0.980 |
Other PD-1 ICIs | 0.20 | 0.637 | 0.319, 1.274 |
First-line chemotherapy | |||
Etoposide + cisplatin, dummy variable | 0.11 | ||
Etoposide + carboplatin | 0.051 | 1.431 | 0.999, 2.050 |
Etoposide + nedaplatin | 0.44 | 0.732 | 0.332, 1.613 |
Other | 0.61 | 0.691 | 0.168, 2.851 |
Cycles of chemotherapy (≥4/<4) | <0.001* | 0.483 | 0.330, 0.707 |
Second to multiple lines immunotherapy + anti-vascular targeted therapy (yes/no) | 0.06 | 0.615 | 0.368, 1.028 |
Second to multiple lines anti-vascular targeted therapy (yes/no) | 0.009* | 0.601 | 0.411, 0.878 |
First-line regimen | |||
ICI + BRT, dummy variables | <0.001 | ||
ICI | <0.001* | 2.695 | 1.588, 4.572 |
BRT | <0.001* | 2.621 | 1.664, 4.127 |
*, statistical significance (P<0.05) included in multifactor Cox regression analysis. HR, hazard ratio; CI, confidence interval; BRT, brain radiotherapy; ICI, immune checkpoint inhibitor; KPS, Karnofsky Performance Status; GPA, Graded Prognostic Assessment score; PD-1, programmed death receptor 1; WBRT, whole brain radiotherapy; WBRT + boost, WBRT with simultaneous integrated boost; SRT, stereotactic radiotherapy.
To minimize covariance and concentrate on statistically significant variables (P<0.05), these factors underwent further analysis using multivariate Cox regression, as outlined in Table 9 (Model 1). This analysis identified several factors significantly associated with OS, including the choice of first-line treatment regimen (with ICI and ICI + BRT as dummy variables), completion of more than four cycles of chemotherapy, the presence of bone metastases, having more than three brain metastases, undergoing more than six cycles of immunotherapy, the administration of second to multiple lines of targeted therapy, and the inclusion of first-line extracranial radiotherapy.
Table 9
Factor | P | HR value | 95% CI for HR |
---|---|---|---|
Number of brain metastases (≥3/<3) | <0.001* | 1.897 | 1.329, 2.708 |
KPS (≥80/<80) | 0.29 | 0.825 | 0.578, 1.179 |
Liver metastases (yes/no) | 0.13 | 1.342 | 0.916, 1.968 |
Bone metastases (yes/no) | 0.009* | 1.744 | 1.152, 2.641 |
First-line extracranial radiotherapy (yes/no) | 0.009* | 0.549 | 0.350, 0.861 |
Immunotherapy cycles (≥6/<6) | 0.03* | 0.536 | 0.302, 0.950 |
Second to multiple lines anti-vascular targeted therapy (yes/no) | <0.001* | 0.388 | 0.253, 0.595 |
Cycles of chemotherapy (≥4/<4) | <0.001* | 0.399 | 0.258, 0.616 |
First-line programs | |||
ICI + BRT, dummy variables | 0.001 | ||
ICI | <0.001* | 2.838 | 1.612, 4.997 |
BRT | 0.03* | 1.957 | 1.083, 3.539 |
*, statistical significance (P<0.05). HR, hazard ratio; CI, confidence interval; KPS, Karnofsky Performance Status; ICI, immune checkpoint inhibitor; BRT, brain radiotherapy.
A more detailed analysis of grouping variables, dividing the first-line regimen into specific immunotherapy and radiotherapy regimens, is presented in Table 10 (Model 2). This refined approach demonstrated that specific immunotherapies (atezolizumab and serplulimab, compared with no ICI) and radiotherapy regimens (WBRT and WBRT + boost compared with no BRT) significantly impacted OS. Additionally, the presence of liver metastasis emerged as a significant factor. The completion of more than four chemotherapy cycles, the presence of bone metastases, having more than three brain metastases, undergoing more than six cycles of immunotherapy, receiving second to multiple lines of targeted therapy, and the application of first-line extracranial radiotherapy retained their significance in this more detailed analysis.
Table 10
Factor | P value | HR value | 95% CI for HR |
---|---|---|---|
Number of brain metastases (≥3/<3) | <0.001* | 1.977 | 1.359, 2.878 |
KPS (≥80/<80) | 0.33 | 0.822 | 0.555, 1.216 |
Liver metastases (yes/no) | 0.04* | 1.521 | 1.024, 2.261 |
Bone metastases (yes/no) | 0.02* | 1.644 | 1.072, 2.521 |
First-line extracranial radiotherapy (yes/no) | 0.004* | 0.495 | 0.307, 0.799 |
Immunotherapy cycle (≥6/<6) | 0.048* | 0.553 | 0.307, 0.996 |
Second to multiple lines targeted therapy (yes/no) | <0.001* | 0.356 | 0.226, 0.559 |
Cycles of chemotherapy (≥4/<4) | <0.001* | 0.388 | 0.245, 0.614 |
BRT methods | |||
No BRT, dummy variable | <0.001* | ||
WBRT | <0.001* | 0.298 | 0.163, 0.545 |
WBRT + boost | <0.001* | 0.232 | 0.099, 0.545 |
SRT | 0.10 | 0.456 | 0.181, 1.150 |
Immunotherapy | |||
No ICI, dummy variable | 0.04 | ||
Durvalumab | 0.14 | 0.582 | 0.283, 1.199 |
Atezolizumab | 0.01* | 0.341 | 0.147, 0.794 |
Tislelizumab | 0.12 | 0.456 | 0.168, 1.237 |
Serplulimab | 0.003* | 0.272 | 0.116, 0.636 |
Other PD-1 ICIs | 0.70 | 0.852 | 0.377, 1.923 |
*, statistical significance (P<0.05). HR, hazard ratio; CI, confidence interval; BRT, brain radiotherapy; ICI, immune checkpoint inhibitor; KPS, Karnofsky Performance Status; PD-1, programmed death receptor 1; WBRT, whole brain radiotherapy; WBRT + boost, WBRT with simultaneous integrated boost; SRT, stereotactic radiotherapy.
Comparison of synchronized and asynchronized ICI + BRT groups
The study distinguished patients receiving ICI and BRT within four weeks as the synchronized group (n=27) and those with intervals greater than four weeks as the asynchronized group (n=21). Baseline characteristics such as the number of brain metastases exceeding three, gender, smoking status, presence of underlying diseases, extracranial distant metastasis, a KPS of 80 or above, age over 60, brain-related symptoms, and a GPA score above 2 showed no significant differences between the groups.
Upon comparison, the median OS was 18.1 months for the synchronized group versus 29.4 months for the asynchronized group, although this difference did not reach statistical significance (P=0.10). However, significant differences emerged in terms of median PFS and median iPFS, which were 11.4 versus 16.7 months (P=0.01) and 13.2 months versus 19.9 months (P=0.03), respectively, favoring the asynchronized group.
ORR, DCR, iORR, and iDCR did not significantly differ between the synchronized and asynchronized groups. Likewise, the incidence of TRAEs and the proportion of patients with RTOG scores of 2 or higher (indicative of acute radiation injury) were comparable between the groups, with no statistically significant differences observed (25.9% in the synchronized group versus 9.5% in the asynchronized group, P=0.28).
Discussion
Our research scrutinized the outcomes of individuals with ES-SCLC brain metastases treated at Zhejiang Cancer Hospital from January 2017 to October 2023. In an era dominated by chemotherapy, our investigation focused on the outcomes and TRAEs among patients administered three distinct first-line therapies: BRT, ICI, and a combination of both (ICI + BRT), without any baseline discrepancies among these groups. Our analysis demonstrated that the combination therapy of ICI + BRT significantly surpassed the efficacy of either treatment alone in median oOS, median PFS, median iPFS, ORR, DCR, iORR, and iDCR.
The ICI + BRT groups demonstrate significant advantages in therapeutic efficacy
Notable differences emerged between the BRT and ICI + BRT groups, with the combination therapy showing superior median OS, PFS, and iPFS. These outcomes mirror trends observed in comparable studies that did not distinguish between primary and secondary brain metastases, wherein immunotherapy markedly enhanced median OS and iPFS compared to standard treatments. For instance, one study documented an increase in median OS from 13.3 to 33.4 months and in iPFS from 6.93 to 10.73 months, consistent with our findings (16). Moreover, a smaller study involving 46 cases observed a non-significant trend towards improved PFS in the ICI + BRT group compared to the BRT group alone, hinting that the sample size may affect the ability to detect statistical differences (17). The ORR, DCR, iORR, and iDCR were notably better in our study and were consistent with prior research. Notably, the iORR for the immunotherapy group was significantly higher than that for the standard treatment group, although iDCR did not differ significantly between the two groups (16). This might be due to the lack of distinction between patients with primary versus secondary metastases in our analysis, highlighting the influence of previous brain-targeted treatments on the efficacy of subsequent ICI therapy. Moreover, this study revealed that first-line ICI therapy has a pronounced benefit on PFS compared to second-line or later ICI therapy in multivariate regression analysis (18), underscoring the potential of early immunotherapy intervention. While the ICI + BRT combination exhibits certain efficacy advantages over BRT alone, the necessity for a more extensive, prospective study to further validate these findings remains clear.
The comparison between the ICI group and the combined ICI and BRT group highlighted significant differences in efficacy, with the ICI + BRT group demonstrating superior outcomes across all metrics: median OS, median PFS, median iPFS, ORR, DCR, iORR, and iDCR. These findings suggest a synergistic effect between radiotherapy and immunotherapy, potentially enhancing the overall anti-cancer response (19). Ionizing radiation facilitates the dendritic cell-mediated cross-presentation of tumor antigens to T cells, fostering a potent anti-tumor immune response, particularly by activating cytotoxic CD8+ T lymphocytes (20). The mobilization and activation of T cells towards the tumor site are pivotal mechanisms in effective cancer immunotherapy (21,22). Moreover, applying higher BRT doses may temporarily breach the blood-brain barrier, enhancing the central nervous system’s exposure to systemic therapeutic agents and promoting the infiltration of immune cells. Conversely, immunotherapy may also alter the tumor microenvironment, contributing to the normalization of tumor vasculature, the reduction of intra-tumor hypoxia, and increased radiosensitivity (20). Such effects suggest that immunotherapy boosts the body’s innate ability to fight cancer and enhances the tumor’s vulnerability to radiation. Supporting evidence shows that patients with brain metastases receiving BRT alongside immunotherapy exhibit greater PFS compared to those receiving chemotherapy alone, especially highlighting the benefits in patients treated with both BRT and immunotherapy (11.4 vs. 5.0 months), as opposed to those without BRT (6.9 vs. 3.4 months) (23). This synergy underscores the recommendation to incorporate BRT into the first-line treatment regimen of chemotherapy combined with immunotherapy for patients with ES-SCLC brain metastases, aiming to maximize therapeutic efficacy.
Incidence of TRAEs remains within acceptable limits
In our study, no significant differences were observed across the treatment groups regarding the level of hematologic toxicity, aligning with findings from previous studies (17). However, the ICI + BRT group demonstrated a notably higher occurrence of severe neutropenia and grade ≥3 hematologic toxicity than the ICI group. This underscores the importance of rigorous monitoring of hematologic parameters, particularly neutrophil counts, in patients undergoing combined immunotherapy and radiation treatment. Interestingly, hypoalbuminemia was significantly less common in the ICI + BRT group than in the BRT group, potentially reflecting the improved tumor response and subsequent alleviation of tumor-associated malnutrition, which in turn may enhance nutritional status and decrease the incidence of hypoalbuminemia. On the other hand, thyroid function abnormalities and troponin elevations are more frequently observed in the ICI + BRT group than the BRT group, suggesting that adding immunotherapy to standard treatment could amplify thyroid- and cardiac-related adverse effects. The proposed mechanism involves activating T cells, previously inhibited by PD-1 and PD-L1 (programmed cell death-ligand 1) interactions, which may recognize cancer cells and normal body antigens, leading to various immune-related TRAEs (24).
No significant difference was noted in the RTOG Acute Radiation Injury Grading Criteria scores between the BRT and ICI + BRT groups, indicating that combining ICI with BRT does not exacerbate radiologic injury. Similar findings have been reported in other studies, which showed no significant increase in radiation leukoencephalopathy rates between combined treatment and radiation alone (17). Therefore, while the combination of ICI and BRT may increase the risk of certain adverse events, particularly regarding thyroid function and neutropenia, the overall incidence of severe TRAEs remains within acceptable limits. This equilibrium of efficacy and safety supports the inclusion of ICI with BRT in managing ES-SCLC brain metastases, although further investigation is needed to fully understand the mechanisms behind the observed adverse effects.
Model 1: ICI + BRT serves as an independent prognostic factor
Model 1 determined that several variables significantly impacted OS, including the choice of first-line treatment (with ICI and ICI + BRT modeled as dummy variables), demonstrating a substantial improvement in OS for the combination therapy group. The findings underscore the superiority of ICI + BRT over using either ICI or BRT alone, reinforcing the value of an integrated treatment approach amid the widespread application of chemotherapy. Other significant predictors included the presence of bone metastases and a higher count of brain metastases (≥3), both of which were associated with poorer OS. Conversely, extensive application of first-line extracranial radiotherapy and engagement in second to multiple lines of targeted therapy was associated with enhanced survival, pointing towards the benefits of aggressive and comprehensive treatment strategies. Thus, this study reaffirms the efficacy of combining ICI with BRT as a first-line treatment modality, suggesting a paradigm that integrates chemotherapy, immunotherapy, and radiation for maximized therapeutic benefit.
Model 2: discussion on ICIs, BRT, and vascular targeted therapy
Further analysis within our study delved into the nuances of first-line treatment strategies by distinguishing between specific radiation and immunotherapy regimens through multivariate Cox regression. This approach unveiled that particular immunotherapies significantly enhanced patient OS, notably atezolizumab and serplulimab (considered without using any ICI as a baseline). Atezolizumab exhibited an hazard ratio (HR) of 0.341, while serplulimab showed an HR of 0.272, indicating robust contributions to prolonging OS in patients with ES-SCLC brain metastases. In the context of broader research, the Impower133 trial (3) assessed the benefit of adding atezolizumab to a first-line platinum and etoposide regimen in 403 ES-SCLC patients, noting marked improvements in median OS (12.3 vs. 10.3 months) and PFS (5.2 vs. 4.3 months). Despite these general findings, the specific impact of atezolizumab on patients with brain metastases remained uncertain. Our findings suggest a potential for atezolizumab to extend OS in this patient subgroup, offering a new perspective on its utility.
Similarly, a subgroup analysis within the ASTRUM-005 trial indicated a slight improvement (although without statistical significance) in survival for patients with brain metastases treated with the PD-1 inhibitor serplulimab (HR =0.61) (8), aligning with our study’s conclusions. These observations underscore the therapeutic promise of these immunotherapies in managing ES-SCLC brain metastases, albeit with the recognition that the optimal dosing and treatment regimen requires further investigation. The demonstrated effectiveness of atezolizumab and serplulimab in improving OS among ES-SCLC patients with brain metastases highlights the potential of targeted immunotherapies in this challenging clinical scenario. However, exploring the most effective treatment modalities and dosing strategies continues, emphasizing the need for ongoing research to refine and validate these findings.
In our analysis, distinct BRT regimens, specifically WBRT without BRT as a reference and WBRT with a boost (WBRT + boost, no BRT as a reference variable), significantly influenced patient OS, establishing them as independent prognostic factors. WBRT remains a cornerstone for achieving local control in patients with SCLC brain metastases, with evidence suggesting that WBRT + boost may offer additional survival benefits. A comparative study (25) has demonstrated that patients receiving WBRT + boost experienced extended median OS compared to those undergoing SRT alone (21.8 vs. 12.9 months). Furthermore, regarding iPFS, the WBRT + boost cohort surpassed both the WBRT-only group (10.8 vs. 6.5 months) and the SRT group (10.8 vs. 7.5 months), findings that resonate with our study’s outcomes. However, our study indicated that the radiotherapy regimen, particularly SRT (considered with no radiation as a dummy variable), did not significantly impact OS. This lack of significance could stem from the intrinsic propensity of SCLC for widespread dissemination, suggesting that even patients presenting with localized brain metastases at diagnosis might harbor undetected micrometastases across the brain, thereby diminishing the isolated impact of SRT on OS (26). Supporting this perspective, other research (27) employing multivariate Cox regression analysis found similar results, where SRT did not confer a significant survival advantage. Consequently, our findings suggest that WBRT and WBRT + boost are independent prognostic factors of SCLC patients with brain metastases. We plan to expand the sample size in future studies to further investigate the efficacy and safety of SRT.
Our findings also highlight the crucial contribution of second-line and subsequent targeted therapies, especially anti-angiogenic agents, in improving patient prognosis. Anlotinib, chosen by a vast majority (92.3%) of our study participants, stood out due to its capacity to cross the blood-brain barrier. This characteristic offers a significant therapeutic benefit for treating brain metastases, which are often challenging to manage because of their seclusion by this barrier (28). A notable phase 2 randomized, double-blind study underscored anlotinib’s effectiveness beyond second-line therapy, demonstrating marked improvements in PFS and OS compared to a placebo. The study also reported significant enhancements in iPFS and iDCR (29), leading to anlotinib receiving approval in 2019 from the Chinese State Food and Drug Administration as a standard third-line treatment for ES-SCLC patients. Following progression from initial therapies, our research endorses the inclusion of anlotinib for patients with brain metastases from SCLC, aiming to better patient outcomes. Moreover, subgroup analyses from the ALTER 1202 trial (30) have demonstrated the advantages of combining anlotinib with ICIs in patients with brain metastases, showing extensions in PFS by three months and OS by 3.7 months, signifying a substantial leap in treatment efficacy.
However, our study did not demonstrate statistically significant differences in integrating immunotherapy with targeted therapy after first-line treatment. This could be attributed to the limited sample size and the inherent variability in the range of immunotherapies and targeted drugs used, potentially introducing confounding factors. This highlights the need for more extensive, focused studies to determine the most effective therapeutic strategies, including the potential benefits of combining anlotinib with ICIs, to improve patients’ survival and quality of life with ES-SCLC brain metastases.
Synchronized group vs. asynchronized group
The integration of BRT with ICI in treating brain metastases from SCLC remains underexplored, with the temporal sequencing of these modalities (synchronized vs. asynchronized) scarcely addressed in the existing literature. Conversely, in NSCLC brain metastasis management, substantial research has established a four-week temporal threshold to differentiate between synchronous and asynchronous combinations of ICI and BRT (31-34). Accordingly, our study segmented the BRT + ICI cohort into synchronized (n=27) and asynchronized groups (n=21) for a more detailed subgroup analysis. This subgroup evaluation revealed no statistically significant differences in outcomes such as median OS, ORR, DCR, and TRAEs between the synchronized and asynchronized groups, apart from PFS and iPFS, indicating a trend towards better efficacy in the asynchronized group. Furthermore, most TRAE incidences, including any grade of hematotoxicity, liver function abnormalities, and other side effects, exhibited a lower tendency in the asynchronized group, suggesting potential advantages in delaying the combination of ICI with BRT. Contrastingly, numerous studies on NSCLC brain metastases treatment suggest that synchronous ICI combined with BRT might offer more substantial benefits than their asynchronous counterparts (20). This discrepancy could stem from the intrinsic differences in malignancy and recurrence patterns between NSCLC and SCLC, highlighting the need for further research to optimize the combination strategy of ICI and BRT specifically for SCLC brain metastases. Determining the most effective integration of these treatments remains a critical area for future investigation, aiming to enhance patient outcomes while minimizing adverse effects.
Limitations
This research offers significant insights into treating ES-SCLC brain metastases yet encounters several limitations. As a single-center retrospective study, it covers a relatively small subset of patients (less than 10% of the total patient population), potentially introducing statistical biases and limiting the generalizability of the findings. In response, our team is dedicated to continuing patient recruitment and expanding the sample size in future studies to enhance the reliability of our conclusions. Furthermore, the study noted a disproportionately high number of male participants. Despite efforts to ensure balanced groupings, this gender disparity could potentially influence the results. Another consideration is the extended duration of the study period. With the relatively recent integration of immunotherapy into the treatment regime for brain metastases in SCLC, variations in treatment approaches over time could serve as confounding variables, impacting the comparability across the three patient groups.
We plan to conduct prospective studies to address these issues and refine our understanding of the most effective treatment strategies for ES-SCLC brain metastases. These future investigations aim to overcome confounding factors associated with the extended study period and the variety of drug types used. Among these is an ongoing “single-arm, single-center phase II clinical study evaluating the efficacy of WBRT combined with tislelizumab and chemotherapy in patients with SCLC harboring brain metastases”, currently in the enrollment phase. Through these endeavors, we aspire to provide a clearer, more comprehensive understanding of the optimal approaches for treating this challenging condition.
Conclusions
The management of ES-SCLC brain metastases benefits significantly from integrating BRT with ICI alongside standard chemotherapy, outperforming the use of ICI or BRT alone. Despite an increased incidence of certain TRAEs, this combination approach demonstrates enhanced efficacy, while the TRAEs remain within an acceptable range. Critical prognostic factors influencing OS in this patient population include the selection of first-line treatment regimens, completion of four or more chemotherapy cycles, presence of bone metastases, having three or more brain metastases, undergoing six or more cycles of immunotherapy, application of second-line or further targeted therapies, and the incorporation of first-line extracranial radiotherapy. Immunotherapy regimens, such as atezolizumab and serplulimab, combined with radiotherapy techniques like WBRT and WBRT with a boost, demonstrate significant potential efficacy.
Moreover, our findings indicate a trend towards better outcomes and potentially lower adverse event rates with asynchronous ICI and BRT treatments compared to synchronous approaches. Nevertheless, definitive conclusions regarding the most effective treatment modalities require further exploration through large-scale prospective clinical trials. With continuous research and development in this field, we aim to enhance treatment strategies, thereby improving prognosis and quality of life for patients afflicted with ES-SCLC and brain metastases.
Acknowledgments
Funding: This work was supported by
Footnote
Reporting Checklist: The authors have completed the STROBE reporting checklist. Available at https://jtd.amegroups.com/article/view/10.21037/jtd-24-654/rc
Data Sharing Statement: Available at https://jtd.amegroups.com/article/view/10.21037/jtd-24-654/dss
Peer Review File: Available at https://jtd.amegroups.com/article/view/10.21037/jtd-24-654/prf
Conflicts of Interest: All authors have completed the ICMJE uniform disclosure form (available at https://jtd.amegroups.com/article/view/10.21037/jtd-24-654/coif). The authors have no conflicts of interest to declare.
Ethical Statement:
Open Access Statement: This is an Open Access article distributed in accordance with the Creative Commons Attribution-NonCommercial-NoDerivs 4.0 International License (CC BY-NC-ND 4.0), which permits the non-commercial replication and distribution of the article with the strict proviso that no changes or edits are made and the original work is properly cited (including links to both the formal publication through the relevant DOI and the license). See: https://creativecommons.org/licenses/by-nc-nd/4.0/.
References
- Chen Y, Paz-Ares L, Reinmuth N, et al. Impact of Brain Metastases on Treatment Patterns and Outcomes With First-Line Durvalumab Plus Platinum-Etoposide in Extensive-Stage SCLC (CASPIAN): A Brief Report. JTO Clin Res Rep 2022;3:100330. [Crossref] [PubMed]
- Bryan S, Masoud H, Weir HK, et al. Cancer in Canada: Stage at diagnosis. Health Rep 2018;29:21-5. [PubMed]
- Horn L, Mansfield AS, Szczęsna A, et al. First-Line Atezolizumab plus Chemotherapy in Extensive-Stage Small-Cell Lung Cancer. N Engl J Med 2018;379:2220-9. [Crossref] [PubMed]
- Rittberg R, Banerji S, Kim JO, et al. Treatment and Prevention of Brain Metastases in Small Cell Lung Cancer. Am J Clin Oncol 2021;44:629-38. [Crossref] [PubMed]
- Seute T, Leffers P, ten Velde GP, et al. Neurologic disorders in 432 consecutive patients with small cell lung carcinoma. Cancer 2004;100:801-6. [Crossref] [PubMed]
- Paz-Ares L, Dvorkin M, Chen Y, et al. Durvalumab plus platinum-etoposide versus platinum-etoposide in first-line treatment of extensive-stage small-cell lung cancer (CASPIAN): a randomised, controlled, open-label, phase 3 trial. Lancet 2019;394:1929-39. [Crossref] [PubMed]
- Wang J, Zhou C, Yao W, et al. Adebrelimab or placebo plus carboplatin and etoposide as first-line treatment for extensive-stage small-cell lung cancer (CAPSTONE-1): a multicentre, randomised, double-blind, placebo-controlled, phase 3 trial. Lancet Oncol 2022;23:739-47. [Crossref] [PubMed]
- Cheng Y, Han L, Wu L, et al. Effect of First-Line Serplulimab vs Placebo Added to Chemotherapy on Survival in Patients With Extensive-Stage Small Cell Lung Cancer: The ASTRUM-005 Randomized Clinical Trial. JAMA 2022;328:1223-32. [Crossref] [PubMed]
- Chung HC, Piha-Paul SA, Lopez-Martin J, et al. Pembrolizumab After Two or More Lines of Previous Therapy in Patients With Recurrent or Metastatic SCLC: Results From the KEYNOTE-028 and KEYNOTE-158 Studies. J Thorac Oncol 2020;15:618-27. [Crossref] [PubMed]
- Rudin CM, Awad MM, Navarro A, et al. Pembrolizumab or Placebo Plus Etoposide and Platinum as First-Line Therapy for Extensive-Stage Small-Cell Lung Cancer: Randomized, Double-Blind, Phase III KEYNOTE-604 Study. J Clin Oncol 2020;38:2369-79. [Crossref] [PubMed]
- Stokes M, Berfeld N, Gayle A, et al. A systematic literature review of real-world treatment outcomes of small cell lung cancer. Medicine (Baltimore) 2022;101:e29783. [Crossref] [PubMed]
- Buriolla S, Pelizzari G, Corvaja C, et al. Immunotherapy in NSCLC Patients with Brain Metastases. Int J Mol Sci 2022;23:7068. [Crossref] [PubMed]
- Suwinski R. Combination of immunotherapy and radiotherapy in the treatment of brain metastases from non-small cell lung cancer. J Thorac Dis 2021;13:3315-22. [Crossref] [PubMed]
- Enright TL, Witt JS, Burr AR, et al. Combined Immunotherapy and Stereotactic Radiotherapy Improves Neurologic Outcomes in Patients with Non-small-cell Lung Cancer Brain Metastases. Clin Lung Cancer 2021;22:110-9. [Crossref] [PubMed]
- Cox JD, Stetz J, Pajak TF. Toxicity criteria of the Radiation Therapy Oncology Group (RTOG) and the European Organization for Research and Treatment of Cancer (EORTC). Int J Radiat Oncol Biol Phys 1995;31:1341-6. [Crossref] [PubMed]
- Chang J, Jing X, Hua Y, et al. Programmed cell death 1 pathway inhibitors improve the overall survival of small cell lung cancer patients with brain metastases. J Cancer Res Clin Oncol 2023;149:1825-33. [Crossref] [PubMed]
- Ma J, Tian Y, Hao S, et al. Outcomes of first-line anti-PD-L1 blockades combined with brain radiotherapy for extensive-stage small-cell lung cancer with brain metastasis. J Neurooncol 2022;159:685-93. [Crossref] [PubMed]
- Wang C, Mu S, Yang X, et al. Outcome of immune checkpoint inhibitors in patients with extensive-stage small-cell lung cancer and brain metastases. Front Oncol 2023;13:1110949. [Crossref] [PubMed]
- Wang Y, Liu ZG, Yuan H, et al. The Reciprocity between Radiotherapy and Cancer Immunotherapy. Clin Cancer Res 2019;25:1709-17. [Crossref] [PubMed]
- Scoccianti S, Olmetto E, Pinzi V, et al. Immunotherapy in association with stereotactic radiotherapy for non-small cell lung cancer brain metastases: results from a multicentric retrospective study on behalf of AIRO. Neuro Oncol 2021;23:1750-64. [Crossref] [PubMed]
- Tsiouprou I, Zaharias A, Spyratos D. The Role of Immunotherapy in Extensive Stage Small-Cell Lung Cancer: A Review of the Literature. Can Respir J 2019;2019:6860432. [Crossref] [PubMed]
- Zhu Y, Cui Y, Zheng X, et al. Small-cell lung cancer brain metastasis: From molecular mechanisms to diagnosis and treatment. Biochim Biophys Acta Mol Basis Dis 2022;1868:166557. [Crossref] [PubMed]
- Chen H, Ma X, Liu J, et al. Real-world evaluation of first-line treatment of extensive-stage small-cell lung cancer with atezolizumab plus platinum/etoposide: a focus on patients with brain metastasis. Clin Transl Oncol 2024;26:1664-73. [Crossref] [PubMed]
- Chalan P, Di Dalmazi G, Pani F, et al. Thyroid dysfunctions secondary to cancer immunotherapy. J Endocrinol Invest 2018;41:625-38. [Crossref] [PubMed]
- Ni M, Jiang A, Liu W, et al. Whole brain radiation therapy plus focal boost may be a suitable strategy for brain metastases in SCLC patients: a multi-center study. Radiat Oncol 2020;15:70. [Crossref] [PubMed]
- Zeng H, Li R, Hu C, et al. Association of Twice-Daily Radiotherapy With Subsequent Brain Metastases in Adults With Small Cell Lung Cancer. JAMA Netw Open 2019;2:e190103. [Crossref] [PubMed]
- Chiang CL, Yang HC, Liao YT, et al. Treatment and survival of patients with small cell lung cancer and brain metastasis. J Neurooncol 2023;165:343-51. [Crossref] [PubMed]
- Xu H, Cao D, Jie F, et al. The efficacy and safety of anlotinib for subsequent line treatment of small cell lung cancer: a systematic review and meta-analysis. Tumori 2023;109:203-14. [Crossref] [PubMed]
- Cheng Y, Wang Q, Li K, et al. Third-line or above anlotinib in relapsed and refractory small cell lung cancer patients with brain metastases: A post hoc analysis of ALTER1202, a randomized, double-blind phase 2 study. Cancer Innov 2023;2:181-90. [Crossref] [PubMed]
- Cheng Y, Wang Q, Li K, et al. Anlotinib vs placebo as third- or further-line treatment for patients with small cell lung cancer: a randomised, double-blind, placebo-controlled Phase 2 study. Br J Cancer 2021;125:366-71. [Crossref] [PubMed]
- Yang Y, Deng L, Yang Y, et al. Efficacy and Safety of Combined Brain Radiotherapy and Immunotherapy in Non-Small-Cell Lung Cancer With Brain Metastases: A Systematic Review and Meta-Analysis. Clin Lung Cancer 2022;23:95-107. [Crossref] [PubMed]
- Hubbeling HG, Schapira EF, Horick NK, et al. Safety of Combined PD-1 Pathway Inhibition and Intracranial Radiation Therapy in Non-Small Cell Lung Cancer. J Thorac Oncol 2018;13:550-8. [Crossref] [PubMed]
- Schapira E, Hubbeling H, Yeap BY, et al. Improved Overall Survival and Locoregional Disease Control With Concurrent PD-1 Pathway Inhibitors and Stereotactic Radiosurgery for Lung Cancer Patients With Brain Metastases. Int J Radiat Oncol Biol Phys 2018;101:624-9. [Crossref] [PubMed]
- Koenig JL, Shi S, Sborov K, et al. Adverse Radiation Effect and Disease Control in Patients Undergoing Stereotactic Radiosurgery and Immune Checkpoint Inhibitor Therapy for Brain Metastases. World Neurosurg 2019;126:e1399-411. [Crossref] [PubMed]