Quantitative evaluation of radiation-induced heart disease in patients with lung cancer: a three-dimensional speckle tracking imaging study
Highlight box
Key findings
• Lung cancer patients receiving radiation therapy experienced a decline in left ventricular (LV) systolic function during radiotherapy.
What is known and what is new?
• Cardiovascular events due to radiation-induced heart disease (RIHD) have become one of the leading causes of death in cancer patients. There are still no clear guidelines for the diagnosis of RIHD, and there is still a gap in early and effective monitoring for the emergence of RIHD.
• In our study, lung cancer patients treated with radiation therapy showed a decrease in LV function at the time of treatment.
What is the implication, and what should change now?
• Further research with extended follow-up periods and larger sample sizes is necessary to fully understand the cardiovascular protective benefits of global longitudinal strain (GLS) monitoring.
Introduction
Radiotherapy is a fundamental treatment for cancer and has been used to treat a range of thoracic tumors such as breast cancer, lung cancer, esophageal cancer, mediastinal lymphoma, and thymoma (1,2). Radiation-induced heart disease (RIHD) represents a significant and severe complication arising from radiotherapy. This condition encompasses a spectrum of cardiac disorders, such as radiation-induced pericarditis, radiation-induced cardiomyopathy, radiation-induced valvular disease, radiation-induced coronary artery disease, and radiation-induced conduction system damage, which manifest following exposure of the heart to specific levels of radiation (3).
Cardiovascular mortality resulting from RIHD has emerged as a prominent cause of death among cancer survivors, thereby diminishing the overall efficacy of radiotherapy (4-6). Hence, it is recommended by oncologists and cardiologists to conduct regular screenings for RIHD in patients who have undergone chest radiotherapy. Based on contemporary guidelines and studies, alterations in global longitudinal strain (GLS) serve as initial indicators of cardiotoxicity, with three-dimensional speckle tracking echocardiography (3D-STE) assessments demonstrating greater alignment with the three-dimensional configuration of the cardiac muscle (7-9).
In this research, 3D-STE was employed for longitudinal monitoring to assess alterations in various parameters of left ventricular (LV) myocardial strain in a cohort of lung cancer patients undergoing radiotherapy for a 12-month period. This approach was complemented by the analysis of myocardial markers to offer insights into predicting RIHD. We present this article in accordance with the STROBE reporting checklist (available at https://jtd.amegroups.com/article/view/10.21037/jtd-24-587/rc).
Methods
Subjects
The observational study was conducted prospectively in The Second Affiliated Hospital of Nanchang University in Nanchang, Jiangxi Province, China from June 2017 to June 2023.
Inclusion criteria were as follows: (I) subjects: patients with pathologically confirmed lung cancer who came to the Oncology Department of The Second Affiliated Hospital of Nanchang University to receive radiotherapy (without chemotherapy during the period) for the first time; (II) irradiation field, dose and segmentation method: irradiation field involved heart, planning target volume prescription dose of 50–66 Gy, 1.8–2 Gy each time, 5 times a week for consecutive 5–6 weeks, irradiation dose of the left ventricle was 10–20 Gy; (III) conventional echocardiography showed no obvious abnormality of heart structure, hemodynamics and cardiac function and LV ejection fraction (LVEF) ≥53%; (IV) electrocardiogram (ECG) examination showed no myocardial ischemia and arrhythmia; (V) serum cardiac troponin I (cTnI) was normal; (VI) the quality of ultrasonic image is clear and meets the requirements of 3D-STE collection and analysis; (VII) patients with an expected survival time of >24 months, and can cooperate to complete relevant examinations.
Exclusion criteria were as follows: (I) organic heart disease and arrhythmia were found by echocardiography, ECG and clinical examination; (II) patients with extra-cardiac diseases such as hypertension, renal insufficiency, diabetes, rheumatoid disease and sleep apnea syndrome that may affect myocardial systolic function; (III) patients who already showed a significant decrease in GLS (defined as GLS <18%) at the pre-radiotherapy examination; (IV) patients failed to complete data collection for 6 times due to various reasons.
The study was conducted in accordance with the Declaration of Helsinki (as revised in 2013). and was approved by the Ethics Committee of The Second Affiliated Hospital of Nanchang University (No. 2023-075). All subjects have signed the informed consent form.
Clinical data acquisition
During the initial visit, clinical information for all participants was documented, encompassing details such as age, type of pathology, stage of tumor, prescribed dose and method of radiotherapy segmentation, average radiation dose to the left ventricle, and medical history. Blood pressure was assessed, standard electrocardiograms were conducted, and serum cTnI levels were analyzed prior to each ultrasound assessment for all participants. The detection of cTnI was carried out using the Beckman Access UniCel system, an automated magnetic microparticle chemiluminescence immunoassay analyzer, along with its corresponding kit.
Conventional echocardiography and 3D-STE data acquisition
According to the process of radiotherapy, conventional echocardiography and 3D-STE scan were performed at 1 day before radiotherapy, 2.5–3 week after beginning radiotherapy (irradiation dose: 26–33 Gy), 5–6 week after beginning radiotherapy (irradiation dose: 50–66 Gy), and 3-, 6- and 12-month after ending radiotherapy, respectively. Philips IE33 ultrasound system (Philips Medical System, Bothell, WA, USA), equipped with a 1–5 MHz phased array transducer (S5-1) and a 1–3 MHz 3D matrix phased array transducer (X3-1), was used for data collection.
Subjects were placed in left lateral position and connected to limb 3-lead electrocardiogram. The studies of conventional echocardiography were performed with the Philips IE33 system and S5-1 phased-array transducer. Measurements of interventricular septal end-diastolic dimension (IVSD), LV posterior wall end-diastolic dimension (LVPWD), LV end-diastolic dimension (LVEDD) and LV end-systolic dimension (LVESD) were acquired in the parasternal long-axis view and obtained perpendicular to the long axis of LV. IVSD and LVPWD were measured in the basal ventricular segment of the respective myocardial wall at the end of diastole. LVEDD and LVESD were measured at the level of the mitral valve leaflet tips at the LV end-diastole and end-systole, respectively. Early (E) and late (A) transmitral inflow velocities were measured in the apical 4-chamber view using conventional pulsed-wave spectral doppler echocardiography. LV end-diastolic volume (LVEDV), LV end-systolic volume (LVESV), LVEF were acquired by two-plane Simpson method, and LV endocardium were traced in the apical four-chamber view and the apical two-chamber view at the end of LV diastole and systole, respectively. Early (e’) and late (a’) diastolic mitral annular peak velocities were measured on the septal side of the mitral annulus in the same view by tissue doppler imaging. LV diastolic function was assessed in terms of the early diastolic mitral inflow velocity-to-early diastolic mitral annular velocity (E/e’) ratio. All the measurements were repeated three times, and the average was calculated.
All 3D data were acquired with the Philips IE33 system and X3-1 matrix phased array transducer. A wide angle acquisition of “full volume” mode was used with four consecutive cardiac cycles from the LV apical four-chamber view during an end-expiration breath hold. The depth and the sector width were adjusted to ensure that the entire LV cavity was included within the pyramidal volume, and the frame rate was optimized to be ≥20 frames/sec. Offline analysis of 3D data was performed using Tom-Tec analysis software (Tom Tec Imaging System GmbH, Unterschleissheim, Bayern, Germany). The analysis software could automatically trace the LV epicardium and endocardium frame by frame from the apical four-chamber view, the apical three-chamber view, and the apical two-chamber view (Figure 1). The tracing could be refined manually. Then, the software automatically divided the LV myocardium into 16 segments, tracked and displayed the strain curves and bull’s eye plots, and obtained the GLS, global radial strain (GRS), global circumferential strain (GCS) and global strain (GS) of the LV myocardium. Proportion of patients with asymptomatic cancer therapy-related cardiac dysfunction (CTRCD) was recorded at each time point according to guidelines (defined as a relative worsening in GLS >15% compared with baseline with LVEF remaining ≥50%) (10).
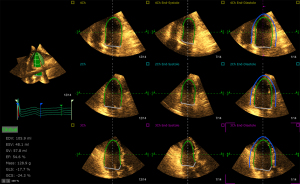
3D-STE repeatability test
The 3D data from 30 randomly selected subjects were analyzed twice at 1-week interval by one investigator for the intra-observer repeatability. The data were analyzed by two independent investigators for the inter-observer repeatability. The investigators were blinded to the other measurements.
Statistical analysis
The SPSS26.0 software package was used for statistical analysis. Descriptive statistical results of continuous variables were expressed as mean ± standard deviation. Differences of continuous variables of multiple groups were tested using the ANOVA. Bland-Atlman analysis was used to evaluate the intra-observer and inter-observer consistency. Inter- and intra-observer repeatability values were evaluated using the intra-class correlation coefficient (ICC). Statistical significance was accepted at a P level of less than 0.05.
Results
Clinical data
The baseline characteristics for all participants are presented in Table 1. A total of 119 patients met the inclusion criteria, of whom 14 (11.7%) were excluded from enrollment for substandard image quality. Finally, total of 105 participants were selected for the study, comprising 90 males and 15 females with an age range of 31 to 69 years and a median age of 58 years. The distribution of lung cancer cases among the subjects included 60 instances (57%) of left lung cancer and 45 instances (43%) of right lung cancer. Furthermore, the cases were categorized into 55 instances of central lung cancer (52%) and 50 instances of peripheral lung cancer (48%). The types of lung cancer observed in the study population consisted of 18 cases of small cell lung cancer (17%), 26 cases of squamous cell carcinoma (25%), and 61 cases of adenocarcinoma (58%).
Table 1
Clinical characteristics | All patients (N=105) |
---|---|
Male, n (%) | 90 (85.7%) |
Age (years), mean ± SD | 58.43±7.00 |
Left lung cancer, n (%) | 60 (57%) |
Type of cancer, n (%) | |
Centralized lung cancer | 55 (52%) |
Peripheral lung cancer | 50 (48%) |
Pathological type, n (%) | |
Adenocarcinoma | 61 (58%) |
Squamous cell carcinoma | 26 (25%) |
Small cell lung cancer | 18 (17%) |
Therapy drugs, n (%) | |
Pemetrexed disodium + carboplatin | 30 (29%) |
Paclitaxel + carboplatin | 12 (11%) |
Etoposide + carboplatin | 13 (12%) |
SD, standard deviation.
There were no notable variances in heart rate, systolic blood pressure, and diastolic blood pressure at each measurement time (Table 2). A total of 35 instances of ECG abnormalities were detected during and up to 12 months after radiotherapy. These included 18 cases of sinus tachycardia, 11 cases of premature contractions in the atria or ventricles, 5 cases of ST-T segment changes, and 1 case of low voltage in the QRS complex. Seven cases of ECG abnormalities were observed 2.5–3 weeks after starting radiotherapy, 25 cases at 5–6 weeks after starting radiotherapy, and 3 cases at 3 months after completing radiotherapy. Out of the 32 instances of ECG abnormalities detected during radiotherapy, 22 cases normalized three months after completing treatment, while 7 cases normalized after six months, and 3 cases remained abnormal even after 12 months. The three abnormalities that emerged three months post-radiotherapy resolved by the six-month mark.
Table 2
Parameters | 1 day before radiotherapy | 2.5–3 weeks after beginning radiotherapy | 5–6 weeks after beginning radiotherapy | 3 months after ending radiotherapy | 6 months after ending radiotherapy | 12 months after ending radiotherapy | P |
---|---|---|---|---|---|---|---|
Heart rate (times/min) | 76.76±7.76 | 77.29±8.33 | 79.85±7.61 | 77.65±5.09 | 77.22±5.68 | 77.56±5.30 | 0.057 |
Systolic blood pressure (mmHg) | 107.84±5.41 | 107.47±4.19 | 108.74±4.70 | 108.78±3.93 | 108.77±5.49 | 108.84±4.27 | 0.10 |
Diastolic blood pressure (mmHg) | 73.99±5.85 | 74.02±4.43 | 75.14±5.14 | 74.90±4.72 | 74.06±5.18 | 74.87±5.15 | 0.34 |
cTnI (ng/mL) | 0.022±0.004 | 0.045±0.081 | 0.054±0.095# | 0.023±0.041 | 0.024±0.069 | 0.023±0.004 | <0.001 |
Data are presented as mean ± standard deviation. #, represent comparison with before radiotherapy, P<0.05. cTnI, cardiac troponin I.
During radiotherapy, the serum cTnI levels of 19 patients rose above the normal range but returned to normal three months after treatment. At 5–6 weeks into radiotherapy, the cTnI levels were significantly higher compared to before treatment (P=0.01). The concentration of serum cTnI at 2.5–3 weeks after beginning radiotherapy and 3-, 6- and 12-month after ending radiotherapy were slightly higher than that before radiotherapy, but the differences were not statistically significant (P=0.06, 0.37, 0.16 and 0.69, respectively) (Table 2).
Conventional echocardiographic results
Before starting radiotherapy, conventional echocardiography did not reveal any clear signs of pericardial effusion or pericarditis in all participants. However, after 5–6 weeks of radiotherapy, 8 subjects developed a small amount of pericardial effusion, with the fluid dark area around the left ventricle measuring approximately 3–6 mm. By three months after completing radiotherapy, the pericardial effusion had resolved.
Univariate ANOVA result showed that there were no significant changes at each time point in IVSD, LVPWD, LVEDD, LVESD, LVEDV, LVESV and LVEF measured by conventional two-dimensional echocardiography and in E, A, and E/A measured by conventional pulsed-wave spectral doppler echocardiography and in a′ measured by TDI (P=0.20, 0.42, 0.82, 0.30, 0.10, 0.15 and 0.18, respectively). Compared with before radiotherapy, e′ (P6<0.001, P12<0.001) and e′/a′ (P6<0.001, P12<0.001) measured by TDI were significantly decreased and E/e′ were significantly increased at 6 and 12 month after ending radiotherapy (P6<0.001, P12<0.001) (Table 3).
Table 3
Parameters | 1 day before radiotherapy | 2.5–3 weeks after beginning radiotherapy | 5–6 weeks after beginning radiotherapy | 3 months after ending radiotherapy | 6 months after ending radiotherapy | 12 months after ending radiotherapy | P |
---|---|---|---|---|---|---|---|
IVSD (mm) | 9.37±1.02 | 9.18±0.89 | 9.52±1.11 | 9.33±1.11 | 9.48±0.98 | 9.33±1.03 | 0.20 |
LVPWD (mm) | 9.23±1.01 | 9.31±1.12 | 9.50±1.19 | 9.37±1.08 | 9.35±1.02 | 9.21±0.91 | 0.42 |
LVEDD (mm) | 47.87±3.27 | 48.22±3.40 | 48.19±3.33 | 47.76±3.11 | 48.30±3.30 | 48.07±3.28 | 0.82 |
LVESD (mm) | 31.31±2.73 | 30.80±2.66 | 31.60±2.58 | 31.47±2.67 | 31.51±2.68 | 31.24±2.70 | 0.30 |
LVEDV (mL) | 111.04±7.49 | 109.97±5.89 | 110.60±5.50 | 110.52±5.71 | 109.58±5.34 | 108.90±5.32 | 0.10 |
LVESV (mL) | 40.26±4.25 | 40.04±3.91 | 39.71±3.86 | 40.67±3.93 | 41.07±4.27 | 40.76±3.97 | 0.15 |
LVEF (%) | 62.68±3.02 | 62.84±3.43 | 62.81±3.71 | 62.09±3.34 | 61.99±3.30 | 62.03±3.70 | 0.18 |
E (cm/s) | 101.30±7.77 | 102.71±7.76 | 103.45±6.76 | 103.17±6.60 | 102.81±7.73 | 103.86±5.64 | 0.15 |
A (cm/s) | 61.95±5.55 | 61.85±5.65 | 60.99±5.78 | 62.54±5.32 | 62.39±5.80 | 62.90±5.49 | 0.23 |
E/A | 1.65±0.21 | 1.68±0.22 | 1.71±0.22 | 1.66±0.19 | 1.66±0.22 | 1.67±0.19 | 0.34 |
e′ (cm/s) | 12.31±1.14 | 12.28±1.07 | 12.20±1.23 | 12.16±1.18 | 11.41±1.08* | 11.09±1.23* | <0.001 |
a′ (cm/s) | 7.09±0.57 | 7.10±0.61 | 7.06±0.56 | 7.07±0.63 | 7.16±0.55 | 7.23±0.57 | 0.25 |
e′/a′ | 1.75±0.23 | 1.74±0.22 | 1.74±0.22 | 1.73±0.22 | 1.60±0.18* | 1.54±0.18* | <0.001 |
E/e′ | 8.29±0.96 | 8.43±0.94 | 8.57±1.04 | 8.57±1.05 | 9.09±1.11* | 9.46±1.09* | <0.001 |
Data are presented as mean ± standard deviation. *, represent comparison with before radiotherapy, P<0.001. IVSD, interventricular septal end-diastolic dimension; LVPWD, left ventricular posterior wall end-diastolic dimension; LVEDD, left ventricular end-diastolic dimension; LVESD, left ventricular end-systolic dimension; LVEDV, left ventricular end-diastolic volume; LVESV, left ventricular end-systolic volume; LVEF, left ventricular ejection fraction; E and A, early and late transmitral inflow velocities; e’ and a’, early and late diastolic mitral annular peak velocities.
Strain changes of left ventricle in 3D-STE
Univariate ANOVA result showed that there were no significant changes in LV mass (LVM) at each time point (P=0.73), but the absolute values of GLS, GRS, GCS and GS were gradually decreased with time at each time point (P<0.001).
In comparison to pre-radiotherapy levels, the GLS, GRS, GCS, and GS values showed a slight decrease at 2.5–3 weeks after starting radiotherapy, but these differences were not considered statistically significant (P=0.16, 0.27, 0.20 and 0.35, respectively). At 5–6 weeks after beginning radiotherapy, the absolute values of GLS and GS were significantly decreased (PGLS<0.001, PGS=0.002), the absolute values of GRS and GCS were slightly decreased. The magnitudes of GLS, GRS, GCS, and GS continued to decrease over time at 3, 6, and 12 months post-radiotherapy cessation (P<0.001) (Table 4 and Figures 2-4). These findings suggest that STE can detect the decline in LV systolic function as soon as during radiotherapy. After 3-month of radiotherapy, the proportion of patients who progressed to CTRCD increased significantly (23, 21.9%). This proportion increased further after 6- and 12-month of radiotherapy.
Table 4
Parameters | 1 day before radiotherapy | 2.5–3 weeks after beginning radiotherapy | 5–6 weeks after beginning radiotherapy | 3 months after ending radiotherapy | 6 months after ending radiotherapy | 12 months after ending radiotherapy | P |
---|---|---|---|---|---|---|---|
GLS (%) | 20.39±1.21 | 19.97±1.18 | 19.20±1.55* | 18.10±1.39* | 17.28±1.29* | 15.96±0.95* | <0.001 |
GRS (%) | 41.60±1.14 | 41.19±1.34 | 41.13±1.19 | 38.90±1.35* | 35.45±2.51* | 31.38±3.32* | <0.001 |
GCS (%) | 32.27±1.44 | 31.97±1.54 | 31.88±1.78 | 30.05±1.99* | 28.81±1.63* | 26.73±1.93* | <0.001 |
GS (%) | 33.12±1.45 | 32.67±1.44 | 32.28±1.66# | 31.21±1.35* | 30.28±1.20* | 29.13±1.18* | <0.001 |
LVM (g) | 161.01±9.39 | 159.51±7.86 | 161.10±8.17 | 160.60±8.39 | 161.13±7.83 | 160.55±8.49 | 0.73 |
CTRCD | – | 0 (0%) | 1 (1%) | 23 (21.9%)* | 59 (56.2%)* | 97 (92.4%)* | <0.001 |
Data are presented as mean ± standard deviation or n (%). #, represent comparison with before radiotherapy, P<0.05; * represent P<0.001. 3D-STE, three-dimensional speckle tracking echocardiography; GLS, global longitudinal strain; GRS, global radial strain; GCS, global circumferential strain; GS, global strain; LVM, left ventricular mass; CTRCD, cancer therapy-related cardiac dysfunction.
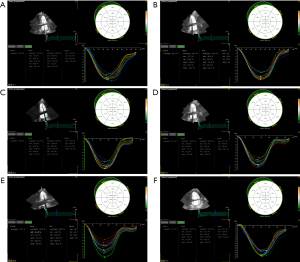
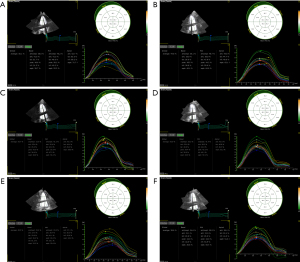
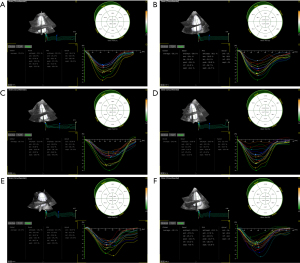
The relationship between cTnI levels, strain parameters and CTRCD
The multivariate linear regression analysis results are presented in Table 5. In linear regression, cTnI levels were significantly correlated with GS (P=0.03) and GLS (P=0.04).
Table 5
Parameters | β | SE (β) | P |
---|---|---|---|
GLS (%) | −0.003 | −0.131 | 0.04 |
GRS (%) | 0.001 | 0.077 | 0.27 |
GCS (%) | 0.000 | 0.010 | 0.86 |
GS (%) | 0.003 | 0.120 | 0.03 |
CTRCD | 0.008 | 0.067 | 0.28 |
cTnI, cardiac troponin I; CTRCD, cancer therapy-related cardiac dysfunction; SE, standard error; GLS, global longitudinal strain; GRS, global radial strain; GCS, global circumferential strain; GS, global strain.
Inter-observer and intra-observer repeatability
The inter-observer reliability measured by the ICC was 0.80, 0.88, 0.84, and 0.82 for GLS, GRS, GCS, and GS, respectively. Intra-observer reliability ICC values were 0.85, 0.90, 0.86, and 0.83 for GLS, GRS, GCS, and GS (Table 6).
Table 6
Parameters | Inter-observer | Intra-observer | |||||
---|---|---|---|---|---|---|---|
Observer1 | Observer2 | ICC | Observer1 | Observer2 | ICC | ||
GLS (%) | 18.26±1.78 | 18.23±1.85 | 0.80 | 18.26±1.78 | 18.3±1.82 | 0.85 | |
GRS (%) | 39.15±4.03 | 38.92±3.95 | 0.88 | 39.15±4.03 | 39.28±4.88 | 0.90 | |
GCS (%) | 30.69±3.55 | 30.60±3.77 | 0.84 | 30.69±3.55 | 30.75±3.53 | 0.86 | |
GS (%) | 32.04±2.85 | 31.99±3.69 | 0.82 | 32.04±2.85 | 32.15±3.62 | 0.83 |
Data are presented as mean ± standard deviation. GLS, global longitudinal strain; GRS, global radial strain; GCS, global circumferential strain; GS, global strain; ICC, intra-class correlation coefficient.
Discussion
The advancement of precision radiotherapy has led to a decrease in the amount of heart radiation through techniques such as intensity-modulated radiation therapy and image-guided radiation therapy (11,12). However, it is still unavoidable that a small portion of heart tissues will receive high doses of radiation. The heart is seen as a critical organ in chest radiotherapy, with even small doses of radiation posing a risk of RIHD (13-15). In this research, patients with lung cancer receiving radiotherapy were prospectively followed up, and 3D-STE and cTnI were employed to assess the alterations in LV systolic and diastolic function caused by early radiotherapy.
As an important marker of myocardial injury, cTnI is only present in cardiomyocytes (16). According to the position statement from the Cardio-Oncology Study Group of the Heart Failure Association (17), cTnI is recommended for early monitoring and identification of heart damage in patients undergoing cancer treatment. In our study, Serum cTnI of 19 patients increased and exceeded the upper limit of normal during radiotherapy, and returned to normal at 3-month after ending radiotherapy. Eight instances of pericardial effusion were identified five to six weeks after beginning radiotherapy, with a notable difference in cTnI levels compared to pre-radiotherapy values. Pericardial effusion and elevated levels of myocardial injury markers may result from reaching the maximum radiotherapy dose at 5–6 weeks. The heart is particularly vulnerable to radiation and at higher risk of acute heart damage. Compared with before radiotherapy, the concentration of serum cTnI were not statistically significant at 3-, 6- and 12-month after ending radiotherapy (P=0.37, 0.16 and 0.69, respectively). This also shows that although cTNI has a certain sensitivity to early cardiac injury monitoring, it cannot reflect the long-term cardiac injury in real time.
The Practical Guidelines for Cancer Treatment and Cardiovascular Toxicity published by the European Society of Cardiology (ESC) in 2016 recommend echocardiography as an important mean for the cardiovascular evaluation of cancer patients (18). In our research, we noted notable variations in e', e'/a', and E/e' at 6 and 12 months post-radiotherapy compared to pre-radiotherapy levels (all P<0.001), indicating a decline in LV diastolic function. There were no significant differences in LVEF at these time points, indicating that the decrease in LV diastolic function occurred before any changes in LVEF, aligning with previous research findings (18,19).
Expert consensus of the European Association of Cardiovascular Imaging and the American Society of Echocardiography in 2013 lists speckle tracking echocardiography as an ultrasonic method for diagnosing early myocardial function impairment associated with cancer treatment (20). Reducing GLS levels can be used to anticipate LV dysfunction, and utilizing GLS-guided cardiac protection during potentially cardiotoxic cancer treatments may help lower cancer therapy-related cardiovascular events (21-23). Prior research has shown a notable decrease in GLS among breast cancer patients following radiotherapy (24-27). And the decrease of GLS was also found in patients with lung cancer after radiotherapy (28). There is a lack of extensive research on assessing the impact of radiotherapy on lung cancer patients’ strain, with short follow-up periods. More studies are required. To minimize the impact of radiation dose variations on the heart, we selected lung cancer patients who received consistent radiation treatment and total dose. We conducted a 12-month follow-up using 3D-STE and compared parameter changes at various intervals.
In this study, we used different strain parameters of 3D-STE to observe the changes of LV systolic function at each time point before and after radiotherapy in patients with lung cancer. We found that the absolute values of GLS and GS began to decrease significantly at 5–6 weeks after the start of radiotherapy (PGLS<0.001, PGS=0.002). At 3, 6 and 12 months after radiotherapy, the absolute values of GRS and GCS gradually showed significant statistical differences (P<0.001). The changes of GLS and GS were earlier than those of conventional echocardiographic parameters, and the changes of GLS had more obvious statistical differences. This proves that GLS has higher sensitivity among different STE parameters. These results suggest that changes in LV systolic function have occurred immediately after radiotherapy, and the application of cTnI and STE monitoring can detect radiation-induced myocardial injury in the early stage. In the 12-month follow-up after radiotherapy, we observed an average decrease of approximately 5% in GLS, which aligns with findings from earlier studies (25,26,29). Previous research has defined a relative decrease in GLS of more than 15% compared with baseline as a form of CTRCD (10,30,31). The proportion of CTRCD increased significantly after 3 months of radiotherapy (P<0.001). And the proportion of CTRCD increased further with longer follow-up. This likewise confirms the sensitivity of using GLS as a test for cardiac dysfunction. Our regression analysis showed that cTnI levels were significantly correlated with GS and GLS. Although a significant increase in cTnI was only seen at 5–6 weeks after the start of radiotherapy, there was still a correlation between strain parameters and cTnI levels.
Damage to the conduction system in patients with RIHD typically presents as arrhythmias. Previous research has shown that ECG abnormalities resulting from radiotherapy typically appear in the second week of treatment and resolve quickly. However, delayed ECG abnormalities tend to develop months to years after completing radiotherapy (32,33). The incidence varies greatly among different studies maybe due to the different lengths of follow-up. In our study, 7 cases (7/105) of ECG abnormality appeared at 2.5–3 weeks after beginning radiotherapy, 25 cases (25/105) appeared at 5–6 weeks after beginning radiotherapy, 3 cases (3/105) at 3-month after ending radiotherapy, and mostly (32/35) returned to normal within 6 months after ending radiotherapy. The result is consistent with previous reports.
In cancer patients receiving thoracic radiotherapy, there is a notable connection between the amount of radiation the heart received and the resulting cardiovascular side effects (34). Our study’s methodology allowed us to decrease radiation exposure dose bias in radiotherapy for the patients we included, making it more dependable to monitor early changes in LV function in lung cancer patients post-radiotherapy. The noticeable reduction in strain parameters after 5–6 weeks of radiotherapy indicates a potential increase in radiation-induced heart damage during treatment. However, further research is needed to determine how to define radiation-induced heart damage based on GLS and the connection between GLS decline and long-term outcomes and cardiovascular events in radiotherapy patients.
Limitations
There are still some limitations in our research. First of all, the number of samples included is small, because long-term follow-up leads to some patients lost to follow-up, and some patients failed to tolerate the termination of radiotherapy plan on the way. Despite taking into account the dose-induced bias, non-radiative factors such as endocrine therapy need to be considered in our study. And the proportion of male patients was significantly higher in this study. On the one hand, this is inherent in the incidence of the disease, and on the other hand men have more exposure factors (smoking or exposure to dusty environments). We will pay attention to these biases in subsequent studies to make the statistical results more representative of the population. Secondly, the follow-up time is limited. Longer follow-up is still needed to monitor the changes of myocardial strain and the occurrence of cardiovascular events in patients undergoing radiotherapy. Finally, although the baseline cardiovascular risk factors were screened, there may still be confounding factors such as family history of cardiovascular disease, diet and transportation, which may affect the accuracy of the final results.
Conclusions
Lung cancer patients receiving radiation therapy experience a decline in LV systolic function during radiotherapy. Following the completion of radiation therapy, patients continue to experience a gradual decline in LV function over time, potentially leading to damage in cardiac conduction function. GLS is more sensitive than other STE parameters, and the evaluation of cTnI combined with GLS can identify radiation-induced heart injury more sensitively in the early stage. By combining the evaluation of cTnI with GLS, radiation-induced heart damage can be identified more sensitively in the initial stages. Providing cardiovascular protection when GLS starts to decline in the early asymptomatic phase could enhance the long-term prognosis for cancer patients and reduce the risk of cardiovascular events. Further research with extended follow-up periods and larger sample sizes is necessary to fully understand the cardiovascular protective benefits of GLS monitoring.
Acknowledgments
Funding: This work was supported by
Footnote
Reporting Checklist: The authors have completed the STROBE reporting checklist. Available at https://jtd.amegroups.com/article/view/10.21037/jtd-24-587/rc
Data Sharing Statement: Available at https://jtd.amegroups.com/article/view/10.21037/jtd-24-587/dss
Peer Review File: Available at https://jtd.amegroups.com/article/view/10.21037/jtd-24-587/prf
Conflicts of Interest: All authors have completed the ICMJE uniform disclosure form (available at https://jtd.amegroups.com/article/view/10.21037/jtd-24-587/coif). The authors have no conflicts of interest to declare.
Ethical Statement: The authors are accountable for all aspects of the work in ensuring that questions related to the accuracy or integrity of any part of the work are appropriately investigated and resolved. The study was conducted in accordance with the Declaration of Helsinki (as revised in 2013). The study was approved by Ethics Committee of The Second Affiliated Hospital of Nanchang University (No. 2023-075) and all subjects have signed the informed consent form.
Open Access Statement: This is an Open Access article distributed in accordance with the Creative Commons Attribution-NonCommercial-NoDerivs 4.0 International License (CC BY-NC-ND 4.0), which permits the non-commercial replication and distribution of the article with the strict proviso that no changes or edits are made and the original work is properly cited (including links to both the formal publication through the relevant DOI and the license). See: https://creativecommons.org/licenses/by-nc-nd/4.0/.
References
- Sárközy M, Varga Z, Gáspár R, et al. Pathomechanisms and therapeutic opportunities in radiation-induced heart disease: from bench to bedside. Clin Res Cardiol 2021;110:507-31. [Crossref] [PubMed]
- Wang KX, Ye C, Yang X, et al. New Insights into the Understanding of Mechanisms of Radiation-Induced Heart Disease. Curr Treat Options Oncol 2023;24:12-29. [Crossref] [PubMed]
- Wei T, Cheng Y. The cardiac toxicity of radiotherapy - a review of characteristics, mechanisms, diagnosis, and prevention. Int J Radiat Biol 2021;97:1333-40. [Crossref] [PubMed]
- Wang H, Wei J, Zheng Q, et al. Radiation-induced heart disease: a review of classification, mechanism and prevention. Int J Biol Sci 2019;15:2128-38. [Crossref] [PubMed]
- Yu Z, Xu C, Song B, et al. Tissue fibrosis induced by radiotherapy: current understanding of the molecular mechanisms, diagnosis and therapeutic advances. J Transl Med 2023;21:708. [Crossref] [PubMed]
- Lancellotti P, Nkomo VT, Badano LP, et al. Expert consensus for multi-modality imaging evaluation of cardiovascular complications of radiotherapy in adults: a report from the European Association of Cardiovascular Imaging and the American Society of Echocardiography. Eur Heart J Cardiovasc Imaging 2013;14:721-40. [Crossref] [PubMed]
- Čelutkienė J, Pudil R, López-Fernández T, et al. Role of cardiovascular imaging in cancer patients receiving cardiotoxic therapies: a position statement on behalf of the Heart Failure Association (HFA), the European Association of Cardiovascular Imaging (EACVI) and the Cardio-Oncology Council of the European Society of Cardiology (ESC). Eur J Heart Fail 2020;22:1504-24. [Crossref] [PubMed]
- Liu JE, Barac A, Thavendiranathan P, et al. Strain Imaging in Cardio-Oncology. JACC CardioOncol 2020;2:677-89. [Crossref] [PubMed]
- Zamorano JL, Lancellotti P, Rodriguez Muñoz D, et al. 2016 ESC Position Paper on cancer treatments and cardiovascular toxicity developed under the auspices of the ESC Committee for Practice Guidelines: The Task Force for cancer treatments and cardiovascular toxicity of the European Society of Cardiology (ESC). Eur Heart J 2016;37:2768-801. [Crossref] [PubMed]
- Lyon AR, López-Fernández T, Couch LS, et al. 2022 ESC Guidelines on cardio-oncology developed in collaboration with the European Hematology Association (EHA), the European Society for Therapeutic Radiology and Oncology (ESTRO) and the International Cardio-Oncology Society (IC-OS). Eur Heart J 2022;43:4229-361. [Crossref] [PubMed]
- Buglione M, Guerini AE, Filippi AR, et al. A Systematic Review on Intensity Modulated Radiation Therapy for Mediastinal Hodgkin's Lymphoma. Crit Rev Oncol Hematol 2021;167:103437. [Crossref] [PubMed]
- Schlaak RA. Advances in Preclinical Research Models of Radiation-Induced Cardiac Toxicity. Cancers (Basel) 2020;12:415. [Crossref] [PubMed]
- Kearney M, Keys M, Faivre-Finn C, et al. Exposure of the heart in lung cancer radiation therapy: A systematic review of heart doses published during 2013 to 2020. Radiother Oncol 2022;172:118-25. [Crossref] [PubMed]
- Podlesnikar T, Berlot B, Dolenc J, et al. Radiotherapy-Induced Cardiotoxicity: The Role of Multimodality Cardiovascular Imaging. Front Cardiovasc Med 2022;9:887705. [Crossref] [PubMed]
- van den Bogaard VAB, Spoor DS, van der Schaaf A, et al. The Importance of Radiation Dose to the Atherosclerotic Plaque in the Left Anterior Descending Coronary Artery for Radiation-Induced Cardiac Toxicity of Breast Cancer Patients? Int J Radiat Oncol Biol Phys 2021;110:1350-9. [Crossref] [PubMed]
- Empana JP, Lerner I, Perier MC, et al. Ultrasensitive Troponin I and Incident Cardiovascular Disease. Arterioscler Thromb Vasc Biol 2022;42:1471-81. [Crossref] [PubMed]
- Pudil R, Mueller C, Čelutkienė J, et al. Role of serum biomarkers in cancer patients receiving cardiotoxic cancer therapies: a position statement from the Cardio-Oncology Study Group of the Heart Failure Association and the Cardio-Oncology Council of the European Society of Cardiology. Eur J Heart Fail 2020;22:1966-83. [Crossref] [PubMed]
- Zamorano JL, Lancellotti P, Rodriguez Muñoz D, et al. 2016 ESC Position Paper on cancer treatments and cardiovascular toxicity developed under the auspices of the ESC Committee for Practice Guidelines: The Task Force for cancer treatments and cardiovascular toxicity of the European Society of Cardiology (ESC). Eur J Heart Fail 2017;19:9-42. [Crossref] [PubMed]
- Di Lisi D, Moreo A, Casavecchia G, et al. Atrial Strain Assessment for the Early Detection of Cancer Therapy-Related Cardiac Dysfunction in Breast Cancer Women (The STRANO STUDY: Atrial Strain in Cardio-Oncology). J Clin Med 2023;12:7127. [Crossref] [PubMed]
- Schiffer W, Pedersen LN, Lui M, et al. Advances in Screening for Radiation-Associated Cardiotoxicity in Cancer Patients. Curr Cardiol Rep 2023;25:1589-600. [Crossref] [PubMed]
- Thavendiranathan P, Negishi T, Somerset E, et al. Strain-Guided Management of Potentially Cardiotoxic Cancer Therapy. J Am Coll Cardiol 2021;77:392-401. [Crossref] [PubMed]
- Negishi T, Thavendiranathan P, Penicka M, et al. Cardioprotection Using Strain-Guided Management of Potentially Cardiotoxic Cancer Therapy: 3-Year Results of the SUCCOUR Trial. JACC Cardiovasc Imaging 2023;16:269-78. [Crossref] [PubMed]
- Yu AF, Ho AY, Braunstein LZ, et al. Assessment of Early Radiation-Induced Changes in Left Ventricular Function by Myocardial Strain Imaging After Breast Radiation Therapy. J Am Soc Echocardiogr 2019;32:521-8. [Crossref] [PubMed]
- Locquet M, Spoor D, Crijns A, et al. Subclinical Left Ventricular Dysfunction Detected by Speckle-Tracking Echocardiography in Breast Cancer Patients Treated With Radiation Therapy: A Six-Month Follow-Up Analysis (MEDIRAD EARLY-HEART study). Front Oncol 2022;12:883679. [Crossref] [PubMed]
- Walker V, Lairez O, Fondard O, et al. Early detection of subclinical left ventricular dysfunction after breast cancer radiation therapy using speckle-tracking echocardiography: association between cardiac exposure and longitudinal strain reduction (BACCARAT study). Radiat Oncol 2019;14:204. [Crossref] [PubMed]
- Erven K, Florian A, Slagmolen P, et al. Subclinical cardiotoxicity detected by strain rate imaging up to 14 months after breast radiation therapy. Int J Radiat Oncol Biol Phys 2013;85:1172-8. [Crossref] [PubMed]
- Trivedi SJ, Choudhary P, Lo Q, et al. Persistent reduction in global longitudinal strain in the longer term after radiation therapy in patients with breast cancer. Radiother Oncol 2019;132:148-54. [Crossref] [PubMed]
- Chen L, Ta S, Wu W, et al. Prognostic and Added Value of Echocardiographic Strain for Prediction of Adverse Outcomes in Patients with Locally Advanced Non-Small Cell Lung Cancer after Radiotherapy. Ultrasound Med Biol 2019;45:98-107. [Crossref] [PubMed]
- Erven K, Jurcut R, Weltens C, et al. Acute radiation effects on cardiac function detected by strain rate imaging in breast cancer patients. Int J Radiat Oncol Biol Phys 2011;79:1444-51. [Crossref] [PubMed]
- Sritharan HP, Delaney GP, Lo Q, et al. Evaluation of traditional and novel echocardiographic methods of cardiac diastolic dysfunction post radiotherapy in breast cancer. Int J Cardiol 2017;243:204-8. [Crossref] [PubMed]
- Thavendiranathan P, Poulin F, Lim KD, et al. Use of myocardial strain imaging by echocardiography for the early detection of cardiotoxicity in patients during and after cancer chemotherapy: a systematic review. J Am Coll Cardiol 2014;63:2751-68. [Crossref] [PubMed]
- Heidenreich PA, Kapoor JR. Radiation induced heart disease: systemic disorders in heart disease. Heart 2009;95:252-8. [Crossref] [PubMed]
- Tao Y, Lu J, Deng W, et al. Correlation of Mean Heart Dose and Cardiac Biomarkers with Electrocardiographic Changes in Patients Receiving Thoracic Radiation Therapy. Radiat Res 2023;199:336-45. [Crossref] [PubMed]
- van den Bogaard VA, Ta BD, van der Schaaf A, et al. Validation and Modification of a Prediction Model for Acute Cardiac Events in Patients With Breast Cancer Treated With Radiotherapy Based on Three-Dimensional Dose Distributions to Cardiac Substructures. J Clin Oncol 2017;35:1171-8. [Crossref] [PubMed]