Predictive model for intraoperative decision-making in video-assisted thoracoscopic lobectomy: optimizing chest drain placement for high-risk patients
Highlight box
Key findings
• In a retrospective multicenter study involving 418 patients who underwent thoracoscopic lobectomy, the rate of tension pneumothorax was found to be 4.53% (n=66) after the removal of a single chest tube. Several factors were analyzed to predict the need for a second intraoperative pleural drain. The most significant variables associated with this need were adhesions, intraoperative lung suturing, fused interlobar fissure, enlarged intrapulmonary lymph nodes, and chronic obstructive pulmonary disease (P<0.001). The model’s C-index was 0.957, with a mean absolute calibration error of 0.6% and a calibration curve slope of 0.959. A specific score indicated a 95% risk of postoperative tension pneumothorax.
What is known and what is new?
• The optimal method for draining the pleural cavity after completing the surgery has been a topic of ongoing debate. Traditionally, two pleural drains were installed following lung resection. However, recent randomized controlled trials and meta-analyses have shown that a single pleural drain is safe and effective following anatomical resection, in both open and thoracoscopic procedures. The use of a single pleural drain is associated with less pain, earlier patient mobilization, shorter duration of drainage, and potential for earlier discharge. Nonetheless, some patients may still require two pleural drain tubes based on their specific characteristics.
What is the implication, and what should change now?
• The predictive model and nomogram could assist in identifying patients who may benefit from a second intraoperative pleural drain placement. This approach allows for personalized care tailored to each patient’s needs.
Introduction
Background
In modern oncological thoracic surgery, predicting the likelihood of complications is a crucial and relevant task. Historically, after anatomic lung resection, the procedure ended with the placement of two pleural tubes—one for fluid drainage from the posterior-lateral surface of the lung and the other for air drainage from the anterior-medial surface (1). Recent advances in video-assisted thoracoscopic surgery (VATS) have demonstrated that the use of a single pleural drain is non-inferior to the use of two in terms of complication rates and provides the additional benefit of pain relief and improvement in cosmetic results (2).
Rationale and knowledge gap
However, there is a subgroup of the patients who develop postoperative pneumothorax or inadequate lung reexpansion and require additional drainage. This subgroup is not clearly defined in the literature.
Objective
The aim of this study was to develop and validate a prognostic model that evaluates the likelihood of developing tension pneumothorax and the need for intraoperative placement of an additional drain in patients undergoing VATS lobectomy. We present this article in accordance with the TRIPOD reporting checklist (available at https://jtd.amegroups.com/article/view/10.21037/jtd-24-617/rc).
Methods
A retrospective multicenter study was conducted at tertiary care facilities, including Thoracic Surgery Department of P. Hertsen Moscow Oncology Research Institute (MORI) and the Thoracic Department of the Regional Clinical Oncologic Dispensary in Ulyanovsk. The study was conducted in accordance with the Declaration of Helsinki (as revised in 2013). The study was approved by the P. Hertsen Moscow Oncology Research Institute Ethics Committee and holds Institutional Review Board approval (#062-A, 15.06.2022). Additionally, participating patients provided written informed consent, and all clinical data were anonymized. The study included 418 patients (their registry data was analyzed) who underwent VATS lobectomy for non-small cell lung cancer (NSCLC) or solitary pulmonary nodule between January 2014 and May 2022. The inclusion criteria for the study were: (I) age over 18 years; (II) thoracoscopic lobectomy; (III) solitary lesion; (IV) fitness for surgery. The exclusion criteria were: (I) thoracotomy, including conversion; (II) combined intervention; (III) incomplete clinical data. According to the additional procedure required, all patients were divided into three groups: (I) patients without postoperative pneumothorax; (II) patients with postoperative non-tension pneumothorax; and (III) patients with postoperative tension pneumothorax who required the placement of a second additional pleural drain (Figure 1).
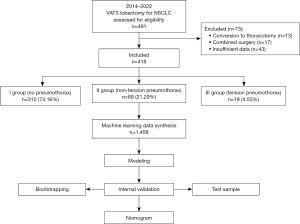
During the preoperative period, a comprehensive assessment of tumor spread was performed in all patients. This included computed tomography (CT) scans of the chest and abdomen, magnetic resonance imaging (MRI) of the brain and bone scintigraphy. Positron emission tomography (PET)-CT has been integrated into standard diagnostic algorithm since 2018. Functional tests such as blood and urine analysis, clotting tests, liver function tests, urea and electrolytes, electrocardiography (ECG), echocardiography (ECHO), ultrasonography of lower extremity veins and spirometry were also performed according to the department protocol. If the functional indicators were inconclusive, Holter monitoring of the ECG, exercise tests (ergometry), assessment of diffusing capacity of the lungs for carbon monoxide (DLCO) and maximal oxygen consumption (VO2max) were performed as necessary. The decision to undergo surgery was made by a multidisciplinary team. Each patient was assessed in an outpatient clinic by an anesthesiologist and a cardiologist.
Perioperative management
All patients underwent thoracoscopic lobectomy using the multiport approach developed by D. Gossot (3). After completion of the surgical procedure, a single 24 Fr pleural drain was inserted on the posterior surface of the operated lung before ventilation was started. A follow-up chest examination was performed two hours after surgery and the following day. In the postoperative period, the principles of accelerated rehabilitation adopted by the department were applied, which included preoperative patient education, thoracic epidural anesthesia, early extubation, and the encouragement of early mobilization. All patients were attached to a pleural suction of −10 to −20 cmH2O on the first postoperative day. If there was no or minimal air leak, patients were transitioned to passive suction. The pleural drain was removed if there was no air leak, and the drainage volume was less than 300 mL/day. In patients with air leak, passive suction was preferred unless respiratory insufficiency was present. In such cases, active pleural suction was used instead. In cases of persistent fluid accumulation after a chest tube removal, a series of repeat thoracenteses were performed. A follow-up examination was performed the day after drain removal to assess lung tissue expansion. For pneumothorax, the height of the air strip was measured from the tip of the pleural cavity in apical pneumothorax or from the lateral edge of the chest wall in extensive pneumothorax. A limited pneumothorax was defined as an accumulation of air in the lower and medial part of the pleural cavity with expanded apex and lateral parts of the lung. An extended pneumothorax was defined as a collection of the air, collapsing the lateral side of the lung in paracostal space. Tension pneumothorax was defined as the presence of free air in the pleural cavity, detected by an X-ray and accompanied by dyspnea and cardiorespiratory failure. In this case an additional chest tube in the second intercostal space along the midclavicular line was placed.
Predicted outcome
The prognostic model predicts the risk of tension pneumothorax development after VATS lobectomy.
Analyzed variables
The present study conducted an analysis of various demographic indicators, including age, gender, smoking history, body mass index (BMI), comorbidities, forced expiratory volume in 1 second (FEV1, %), previous chest surgeries, and tumor-related parameters, such as tumor location, stage of disease according to the 8th revision of the tumour, node, metastasis (TNM) staging system, histological structure, and neoadjuvant therapy. Perioperative data were also collected, including operation duration, amount of blood loss, albumin level on the 1st postoperative day, operation side, presence of adhesion process, lung suturing, fusion of the interlobar fissure, number of staplers applied to the lung parenchyma, hormonal therapy in the postoperative period and its duration. In addition, data on the postoperative lung tissue condition were collected, such as presence of pneumothorax, type of pneumothorax (apical, extended, localized), size of air strip measured by X-ray, information on additional pleural drainage insertion, duration of drainage, presence of prolonged air leak for >5 days, information on lung expansion after drainage removal, and volume of air strip if lung tissue is not expanded.
The severity of postoperative complications was assessed using the Clavien-Dindo classification (4). Primary data collection was performed using a proprietary information system, which is built on open technologies ASP.Net Core, C#, PostgreSQL. One of the significant features of the system, in the context of this study, is its ability to record primary medical parameters (such as physical measurements and degree of invasion) and calculate secondary parameters based on them (such as TNM 8th ed.). Patient data were collected in the system in two stages: automated import of raw data from existing medical information systems and data enrichment through a user interface to enhance their analytical power. Data extraction from the system for subsequent statistical analysis was performed in Excel format.
Data preparation
In this study, missing values were eliminated by multiple imputation of data using the Multiple Imputation by Chained Equations (MICE) method. To determine indicators between groups, univariate analysis was performed and a number of covariates with prognostic value were identified. Factors with variance close to zero and collinear covariates were excluded. Synthetic data were generated with an a priori distribution consistent with the primary data to increase the power of the test using machine learning methods, and the sample size calculation suggested by Riley was used to determine the required sample size (5). The synthesized dataset was used as the training set, while the original dataset was used as the test set and was not included in the model training.
Development of prognostic model
Binary (binomial) multivariate logistic regression with backward stepwise predictor selection by the Akaike Information Criterion was used for modeling. The model was evaluated during internal validation using the bootstrap method (400 iterations) and validation using the testing set. Validation indicators were analyzed, and calibration curves were generated for internal and external validation. An ordinal correlation was found between prognostic indicators and the risk of developing tension pneumothorax, and a nomogram was constructed using the “rms” package in the R program. The score characteristics, levels of the linear predictor, and risk of complication development were reflected.
Statistical analysis
Quantitative indicators were assessed for normality using the Shapiro-Wilk test (for a sample size less than 50) or the Kolmogorov-Smirnov test (for a sample size more than 50). For normally distributed data, mean arithmetic values (M), standard deviations (SDs), and the 95% confidence interval (95% CI) were calculated. Collections of non-normally distributed data were described using the median (Me) and lower and upper quartiles (Q1–Q3). Nominal data were described with absolute values and percentages. Statistical significance was set at a P value of less than 0.05. Student’s t-test was used to compare means in normally distributed populations of quantitative data, while the Mann-Whitney U test or Kruskal-Wallis test was used to compare independent populations of non-normally distributed data. Pearson’s Chi-squared test was used to compare nominal data. The R program (version 4.2.2) was used for all statistical calculations.
Results
In the analysis of the initial data (n=418), the frequency of postoperative pneumothorax was 21.29% (n=89), and tension pneumothorax was observed in 4.55% of cases (n=19). The general characteristics of the studied groups are presented in Table 1.
Table 1
Characteristic | I group (N=310) | II group (N=89) | III group (N=19) | P |
---|---|---|---|---|
Clinical characteristics | ||||
Age, years | 0.86 | |||
Mean ± SD | 62.11±10.88 | 61.18±11.42 | 63.11±6.4 | |
Min; max | 31.0; 84.0 | 25.0; 84.0 | 46.0; 70.0 | |
T criteria (TNM 8th ed.), n (%) | 0.008* | |||
T1a | 16 (5.16) | 4 (4.49) | 1 (5.26) | |
T1b | 100 (32.26) | 21 (23.6) | 4 (21.05) | |
T1c | 104 (33.55) | 20 (22.47) | 6 (31.58) | |
T2a | 58 (18.71) | 27 (30.34) | 2 (10.53) | |
T2b | 18 (5.81) | 9 (10.11) | 5 (26.32) | |
T3 | 14 (4.52) | 8 (8.99) | 1 (5.26) | |
N criteria (TNM 8th ed.), n (%) | 0.004* | |||
N0 | 251 (80.97) | 78 (87.64) | 11 (57.89) | |
N1 | 36 (11.61) | 9 (10.11) | 8 (42.11) | |
N2 | 23 (7.42) | 2 (2.25) | 0 (0.0) | |
Stage (TNM 8th ed.), n (%) | 0.31 | |||
IA1 | 13 (4.19) | 4 (4.49) | 1 (5.26) | |
IA2 | 74 (23.87) | 15 (16.85) | 5 (26.32) | |
IA3 | 69 (22.26) | 18 (20.22) | 6 (31.58) | |
IB | 60 (19.35) | 27 (30.34) | 2 (10.53) | |
IIA | 14 (4.52) | 7 (7.87) | 1 (5.26) | |
IIB | 48 (15.48) | 15 (16.85) | 4 (21.05) | |
IIIA | 26 (8.39) | 1 (1.12) | 0 (0.0) | |
IIIB | 1 (0.32) | 2 (2.25) | 0 (0.0) | |
IVA | 4 (1.29) | 0 (0.0) | 0 (0.0) | |
IVB | 1 (0.32) | 0 (0.0) | 0 (0.0) | |
Histology, n (%) | <0.001* | |||
Adenocarcinoma | 251 (80.97) | 56 (62.92) | 10 (52.63) | |
Squamous cell carcinoma | 36 (11.61) | 13 (14.61) | 9 (47.37) | |
Neuroendocrine tumour | 13 (4.19) | 5 (5.62) | 0 (0.0) | |
Benign | 3 (0.97) | 5 (5.62) | 0 (0.0) | |
Inflammatory disease | 2 (0.65) | 7 (7.87) | 0 (0.0) | |
Other | 5 (1.61) | 3 (3.37) | 0 (0.0) | |
COPD, n (%) | <0.001* | |||
Yes | 40 (12.9) | 17 (19.1) | 11 (57.89) | |
No | 270 (87.1) | 72 (80.9) | 8 (42.11) | |
FEV1, % | 0.056 | |||
Mean ± SD | 91.47±21.37 | 87.87±21.41 | 79.89±20.34 | |
95% CI | 88.21; 94.74 | 83.35; 92.38 | 70.09; 89.7 | |
Min; max | 42.0; 152.0 | 37.0; 145.0 | 51.0; 111.0 | |
BMI, kg/m2 | 0.69 | |||
Mean ± SD | 27.71±4.75 | 27.96±5.28 | 27.07±4.12 | |
Min; max | 20.0; 42.8 | 16.98; 43.11 | 23.0; 37.8 | |
Smoking, n (%) | 0.59 | |||
Yes | 126 (40.65) | 30 (33.71) | 6 (31.58) | |
No | 184 (59.35) | 59 (66.29) | 13 (68.42) | |
Neoadjuvant therapy, n (%) | 0.59 | |||
Yes | 2 (0.65) | 1 (1.12) | 0 (0.0) | |
No | 308 (99.35) | 88 (98.88) | 19 (100.0) | |
Previous lung surgery, n (%) | 0.11 | |||
No | 309 (99.68) | 86 (96.63) | 19 (100.0) | |
Ipsilateral lung | 0 (0.0) | 1 (1.12) | 0 (0.0) | |
Contralateral lung | 1 (0.32) | 2 (2.25) | 0 (0.0) | |
Surgical characteristics | ||||
Duration, min | 0.24 | |||
Mean ± SD | 186.3±59.88 | 176.3±41.12 | 211.0±89.61 | |
Min; max | 30.0; 460.0 | 100.0; 290.0 | 110.0; 450.0 | |
Blood loss, mL | 0.16 | |||
Mean ± SD | 114.4±104.5 | 91.69±59.36 | 98.42±83.62 | |
Min; max | 10.0; 1,100.0 | 30.0; 300.0 | 0.0; 300.0 | |
Albumin, g/L | 0.02* | |||
Mean ± SD | 38.13±5.38 | 35.99±4.01 | 34.52±4.14 | |
Min; max | 29.9; 56.8 | 24.8; 45.6 | 24.5; 41.2 | |
Side, n (%) | 0.44 | |||
Left | 134 (43.23) | 35 (39.33) | 10 (52.63) | |
Right | 176 (56.77) | 54 (60.67) | 9 (47.37) | |
Lobe, n (%) | 0.30 | |||
Upper | 159 (51.29) | 53 (59.55) | 10 (52.63) | |
Middle | 28 (9.03) | 4 (4.49) | 0 (0.0) | |
Lower | 123 (39.68) | 32 (35.96) | 9 (47.37) | |
Intraoperative adhesions, n (%) | <0.001* | |||
Yes | 7 (2.26) | 16 (17.98) | 11 (57.89) | |
No | 303 (97.74) | 73 (82.02) | 8 (42.11) | |
Intraoperative lung suturing, n (%) | <0.001* | |||
Yes | 8 (2.58) | 10 (11.24) | 8 (42.11) | |
No | 302 (97.42) | 79 (88.76) | 11 (57.89) | |
Interlobar fissure, n (%) | <0.001* | |||
Fused | 99 (31.94) | 43 (48.31) | 12 (63.16) | |
Non-fused | 211 (68.06) | 46 (51.69) | 7 (36.84) | |
Number of staplers used | 0.001* | |||
Mean ± SD | 1.79±1.06 | 2.12±1.46 | 3.0±1.7 | |
Min; max | 0.0; 4.0 | 0.0; 6.0 | 0.0; 6.0 | |
Postoperative corticosteroids, n (%) | 0.01* | |||
Yes | 20 (6.45) | 10 (11.24) | 3 (15.79) | |
No | 290 (93.55) | 79 (88.76) | 16 (84.21) | |
Duration of postoperative corticosteroids, days | <0.001* | |||
Mean ± SD | 0.112±0.529 | 2.33±1.0 | 0.765±1.25 | |
Min; max | 0.0; 4.0 | 1.0; 4.0 | 0.0; 3.0 | |
Length of hospital stay, days | <0.001* | |||
Mean ± SD | 7.44±2.42 | 9.03±4.26 | 18.74±3.09 | |
Min; max | 2.0; 14.0 | 4.0; 23.0 | 12.0; 24.0 |
I group: patients without postoperative pneumothorax. II group: patients with postoperative non-tension pneumothorax. III group: patients with postoperative tension pneumothorax who required the placement of a second additional pleural drain. *, P<0.05. SD, standard deviation; TNM 8th ed., 8th revision of the tumour, node, metastasis staging system; COPD, chronic obstructive pulmonary disease; FEV1, forced expiratory volume in 1 second; CI, confidence interval; BMI, body mass index.
Information on postoperative observations is presented in Table 2. The overall complication rate, including non-tension and tension pneumothorax (not shown in this table as they are grouping factors), was 28.71% (n=120). In the III group, an additional pleural drain was installed in all patients, which led to the complications being classified as IIIa according to the Clavien-Dindo scale.
Table 2
Complication | I group (N=310) | II group (N=89) | III group (N=19) | P |
---|---|---|---|---|
Arrhythmia | 7 (58.4%) | 4 (80%) | 4 (50%) | 0.19 |
Hydrothorax | 3 (25%) | NA | NA | |
COVID-19 | 1 (8.3%) | NA | NA | |
Haemothorax | 1 (8.3%) | NA | NA | |
Pneumonia | NA | NA | 4 (50%) | |
Chylothorax | NA | 1 (20%) | NA |
I group: patients without postoperative pneumothorax. II group: patients with postoperative non-tension pneumothorax. III group: patients with postoperative tension pneumothorax who required the placement of a second additional pleural drain. COVID-19, coronavirus disease 2019.
The total duration of drainage was significantly higher in the III group compared to the II group (12.53 vs. 5.33 days, P<0.001). In addition, extended pneumothorax was significantly more common in group III (84.21% vs. 28.09% in group II), often requiring additional drainage (see Table 3). Incomplete lung re-expansion was more common in the group requiring additional pleural drainage (47.37% vs. 58.43% in group II), but the data were not significantly different (P=0.38). Nevertheless, the size of the air strip in patients with residual pneumothorax after drain removal was reduced in all patients in the III group and did not differ from this parameter in the II group (P=0.81).
Table 3
Characteristic | I group (N=310) | II group (N=89) | III group (N=19) | P |
---|---|---|---|---|
Prolonged air leak, n (%) | <0.001* | |||
Yes | 0 (0.0) | 19 (21.35) | 19 (100.0) | |
No | 310 (100.0) | 70 (78.65) | 0 (0.0) | |
Duration of drainage, days | <0.001* | |||
Mean ± SD | 2.75±1.27 | 5.33±4.16 | 12.53±5.12 | |
Min; max | 1.0; 13.0 | 2.0; 20.0 | 8.0; 28.0 | |
Pneumothorax type, n (%) | <0.001* | |||
Apical | Not applicable | 50 (56.18) | 3 (15.79) | |
Extended | 25 (28.09) | 16 (84.21) | ||
Localized | 14 (15.73) | 0 (0.0) | ||
Size of the air strip, mm | 0.02* (II vs. III) | |||
Mean ± SD | 0.0±0.0 | 23.13±21.58 | 40.11±28.51 | |
Min; max | 0.0; 0.0 | 0.0; 100.0 | 7.0; 112.0 | |
Full lung expansion, n (%) | 0.38 (II vs. III) | |||
Yes | 310 (100.0) | 37 (41.57) | 10 (52.63) | |
No | 0 (0.0) | 52 (58.43) | 9 (47.37) | |
Complications, n (%) | <0.001* | |||
Yes | 12 (3.87) | 89 (100.0) | 19 (100.0) | |
No | 298 (96.13) | 0 (0.0) | 0 (0.0) | |
Size of the air strip after drain removal, mm | 0.81 (II vs. III) | |||
Mean ± SD | 18.60±15.11 | 19.83±17.99 | ||
Min; max | 0; 75 | 0; 47 | ||
Complication grade, n (%) | <0.001* | |||
I | 0 (0.0) | 0 (0.0) | 0 (0.0) | |
II | 11 (91.67) | 89 (100.0) | 0 (0.0) | |
IIIa | 1 (8.33) | 0 (0.0) | 19 (100.0) |
I group: patients without postoperative pneumothorax. II group: patients with postoperative non-tension pneumothorax. III group: patients with postoperative tension pneumothorax who required the placement of a second additional pleural drain. *, P<0.05. SD, standard deviation.
The analysis identified the main prognostic factors for the development of tension pneumothorax after surgery, including the presence of adhesions, intraoperative lung sutures due to multiple defects in the lung tissue, the severity of the interlobar fissure, the presence of chronic obstructive pulmonary disease (COPD) (P<0.001), and involvement of intrapulmonary lymph nodes (P=0.002). After applying machine learning methods and data synthesis for model validation, the total sample size for prognostically significant covariates was set at 1,458 patients. A likelihood ratio test was performed to evaluate the contribution of each factor to the prognostic effectiveness of the model (see Figure 2).
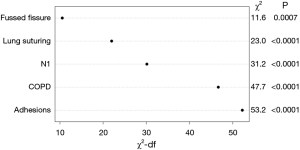
Following a rigorous internal validation, the following adjusted model characteristics were obtained (see Table 4).
Table 4
Characteristic | Value |
---|---|
Coefficient of determination | 0.566 |
C-index | 0.957 |
Mean absolute calibration error | 0.6% |
Calibration slope | 0.959 |
Calibration intercept | −0.049 |
During internal validation using bootstrapping and the test data set, a calibration curve was created. The mean calibration error for the test data set was 1.9% and the C-index was 0.958. Through the analysis of the prognostic model, a nomogram was created that determined the risk contribution of each variable to the development of tension pneumothorax after thoracoscopic lobectomy, which may require additional pleural drainage (see Figure 3).
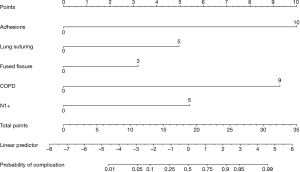
A total score of 7 points corresponds to a 1% risk of a complication occurring. With a value of 11 points, the risk increases to 5%. A score of 13 is associated with a 10 percent risk of complications. If a patient’s score reaches 16 points, the risk increases significantly to 25%. A total score of 19 points indicates a 50% risk of complications. At 22 points, the risk increases to 75%. With a value of 24 points, there is a 90% risk of complications. In patients with a score of 26 points, the risk is particularly high at 95%. Finally, a total score of 31 points is associated with a complication risk of 99%, which means a high probability of tension pneumothorax occurring in the postoperative period.
Discussion
Lung cancer continues to maintain its prominent position in the prevalence and mortality rates among oncological diseases (6). Anatomical lung resections of varying extent have retained their relevance as a radical treatment method not only for localized forms of the disease, but also for locally advanced forms of tumors as part of multimodal therapy. Despite advances in surgical technology, popularization and widespread adoption of thoracoscopic techniques, there has been no decline in the problem of surgical complications after lung resections.
According to the European Society of Thoracic Surgeons’ database, from 2012 to 2020, the frequency of cardiorespiratory complications decreased from 16.8% to 11.6%, in contrast to the frequency of the development of prolonged air leak, which remain constant and was 9.4% and 11.1% during the periods 2007–2014 and 2015–2022, respectively (7).
According to various authors, the frequency of prolonged air leak (>5 days) after elective anatomical lung resections varies widely, ranging from 5% to 60%, and depends on numerous factors related to both the patient’s constitutional and somatic characteristics and the nuances of the surgical procedure (8). Prolonged air leak serves as an independent predictor for longer length of hospital stay, the risk of development of infectious complications (pneumonia, pleuritis, and pleural empyema), exacerbation of COPD, and progression of respiratory failure in the postoperative period (9). Prolonged pleural cavity drainage for postoperative air leak significantly (up to 8 times) increases the risk of infection and the development of pleural empyema (10). In addition to the risk of various clinical complications, prolonged air leak is associated with documented economic costs, making the study of the causes and prevention of air leak insufficiency an ongoing and relevant issue, particularly in the context of planning the operations of thoracic surgery departments (11).
Among the risk factors for air leak, the most significant are the presence of lung emphysema, male gender, repeated surgeries on the chest organs, and dense adhesions in the pleural cavity, as well as a decrease in the lung function’s flow rate indicators (12). However, from a clinical perspective, the most critical aspect in the postoperative period is not just the fact of the air leak itself, but also the volume and rate of air entry through the pleural drains.
An increase in the rate of air entry during air leak insufficiency can lead to tension pneumothorax and the need for additional pleural drainage. This, especially in the presence of pronounced emphysema of the soft tissues of the chest wall, can be associated with additional difficulties and risks of complications.
The search for methods to prevent air leak insufficiency following lung resection over the last 15 years has led to the intensive development of digital pleural cavity drainage systems. This has also given rise to new adhesive and applicative reinforcement systems for mechanical and manual sutures, as well as the development of a consensus regarding pleural cavity drainage with the use of active and passive aspiration (13,14).
Despite all of these measures, each of which deserves individual attention, their popularization has not yet had an impact on the frequency of air leak insufficiency. The results of numerous studies in this field continue to be contradictory with respect to their overall effectiveness (15).
The selection of the optimal method for pleural cavity drainage after completing the primary surgical phase has remained a subject of ongoing debate. For a long time, the traditional approach following anatomical lung resection was the installation of two pleural drains. However, currently, the results of randomized controlled trials (RCTs) and subsequent meta-analyses have demonstrated the safety of placing a single pleural drain following anatomical resection, both in open and thoracoscopic procedures (1).
The installation of a single pleural drain is associated with less pain, earlier patient mobilization, a shorter duration of pleural cavity drainage, and, consequently, the potential for earlier hospital discharge. The advantages of placing a single pleural drain after standard lung resections have been endorsed in a consensus by the European Society of Thoracic Surgeons (15). Authors have noted that in some clinical observations, additional pleural cavity drainage may be necessary if air evacuation is inadequate with a single drain. According to Pawelczyk and colleagues, the frequency of re-drainage of the pleural cavity in the postoperative period can reach 11–20% (16). In clinical guidelines from the Chinese Society of Thoracic Surgeons regarding postoperative patient management, a differentiated approach to pleural cavity drainage is established for cases at high risk of massive air leak (17).
In the present study, a predictive model for prolonged air leak following thoracoscopic lobectomy has been developed using a nomogram, enabling intraoperative decision-making regarding the necessity of additional pleural cavity drainage. It is important to emphasize that the idea of predicting air leak after surgery is not new, and several scales have been proposed to assess the risk of air leak insufficiency (18,19). However, each of these models has its own specific features and cannot be directly translated into routine clinical practice without reservations. Additionally, these scales do not offer a correction in the decision-making strategy for intraoperative pleural cavity drainage, which could be valuable in preventing postoperative complications in cases of prolonged air leak and tension pneumothorax. For example, in the study by Orsini and colleagues, only 7.9% of patients underwent video-assisted surgery, and caution should be exercised when extrapolating these data to the group of patients after video-assisted procedures (19).
Among the numerous risk factors for air leak insufficiency in the presented study, the most significant factors that increase the likelihood of tension pneumothorax, requiring additional drainage, included the presence of adhesions in the pleural cavity, attempts at additional manual suturing of lung tissue, the absence of a pronounced interlobar fissure, the presence of COPD, and intrapulmonary lymphadenopathy. According to Li et al., adhesions in the pleural cavity significantly extend the duration of pleural cavity drainage and hospital stay, primarily due to the necessity of performing pneumolysis, during which the risk of lung parenchymal injury is greatly increased (20). Naturally, there is no objective classification of adhesions in the pleural cavity, but some authors have proposed analogous methods for assessing the extent of adhesions in the pleural cavity (21). Therefore, the assessment of the severity of adhesions remains a subjective criterion, as does the possibility of trauma-free pneumolysis. This largely depends on the specific surgeon’s experience and, consequently, can have a different impact on the risk of postoperative complications. During thoracoscopic procedures, it can be quite challenging to examine the entire surface of the remaining lung tissue and perform a thorough water test. The posterior surface of the lung is often inaccessible for inspection.
It is essential to highlight the influence of lung emphysema, as reflected in the parameter “COPD”, however, the flow rate indicators of lung function did not have a significant impact on the risk of developing tension pneumothorax. This underscores the role of the structural, rather than functional, condition of the lung parenchyma in the risk of air leak. This factor likely had particular significance for patients with intrapulmonary lymphadenopathy, where there was an additional need for dissection in the lung hilum. It is important to use digital draining systems in COPD patients and to quantify the air leak. This may allow for more precise establishment of indications for an additional chest drain, especially when a bedside X-ray is not available.
The obliteration of the interlobar fissure significantly complicates the identification of vascular structures. In this situation, surgical techniques such as “fissureless” and “one direction” lobectomy are gaining increasing popularity (22). Each technique has its advantages. However, in cases of anatomical variability of pulmonary vessels, the most comprehensive revision is performed through the interlobar approach, requiring precise dissection of lung tissue. In the model obtained in this study, the absence of a pronounced interlobar fissure made a minimal contribution to the development of air leak insufficiency. This may be associated with the active application of the aforementioned atraumatic methods for separating the interlobar fissure. Developing a classification system for the severity of interlobar fissure fusion could further enhance the study of lung surgery outcomes.
The placement of an additional drain in group III not only resolves acute respiratory insufficiency but also improves the immediate treatment outcomes and creates better conditions for lung expansion. In patients with a residual pleural space, the size of the air strip before discharge did not differ between groups II and III (P=0.81).
One of the important directions in improving the outcomes of surgical treatment for patients is the standardization of approaches and practices for various conditions. However, the vast majority of clinical guidelines are developed for standard situations, and deviations from the standard course may require decision-making not directly specified in the guidelines. In this study, an algorithm for decision-making regarding the nature of pleural cavity drainage in patients after thoracoscopic anatomical lung resection was developed, depending on factors that significantly influence the risk of postoperative air leak insufficiency and, separately, the need for additional drainage in the postoperative period. The decision-making algorithm is implemented through the construction of a nomogram, which may allow for the objective decision on additional drainage in the future. The results of the analysis are user-friendly and can be customized to include variables specific to individual patients. Our local policy was to insert an additional chest tube if a risk of postoperative complication was more or equal 90%.
Limitations of the study
This analysis was conducted on a retrospective cohort of patients collected over 7 years in a high-volume center primarily treating patients with lung tumor diseases. Groups were defined by the outcome and not randomized. Despite internal validation of the results, the practical application of the nomogram requires prospective evaluation regarding the reduction in the risk and duration of air leak insufficiency and the reduction in the need for additional drainage after implementing the proposed decision-making algorithm. It is also essential to consider that in this study, over 90% of patients were operated on by surgeons with more than 10 years of experience in performing thoracoscopic surgeries, which may influence the overall frequency of air leak insufficiency and requires special attention to the protocol during the learning phases of thoracoscopic technologies. The present nomogram requires separate validation for patients with chronic inflammatory lung diseases and tuberculosis, as well as for repeat operations on chest organs.
Conclusions
As a result of the analysis, a prognostic model for the development of tension pneumothorax after thoracoscopic lobectomy has been developed. Significant risk factors have been identified, including the presence of pleural adhesions, intraoperative suturing of lung tissue, obliterated interlobar fissure, a history of COPD, and enlarged intrapulmonary lymph nodes. The nomogram proposed in the study can serve as an additional tool for making objective decisions about pleural drainage methods following thoracoscopic anatomical lung resection. Referring a patient to the high-risk group allowed us to place a second drain intraoperatively, thus preventing possible episodes of acute respiratory failure and improving the expansion of the operated lung. Additional clinical prospective validation of the proposed algorithm is necessary to assess the impact of its routine use on reducing the frequency of postoperative complications.
Acknowledgments
Funding: None.
Footnote
Reporting Checklist: The authors have completed the TRIPOD reporting checklist. Available at https://jtd.amegroups.com/article/view/10.21037/jtd-24-617/rc
Data Sharing Statement: Available at https://jtd.amegroups.com/article/view/10.21037/jtd-24-617/dss
Peer Review File: Available at https://jtd.amegroups.com/article/view/10.21037/jtd-24-617/prf
Conflicts of Interest: All authors have completed the ICMJE uniform disclosure form (available at https://jtd.amegroups.com/article/view/10.21037/jtd-24-617/coif). The authors have no conflicts of interest to declare.
Ethical Statement: The authors are accountable for all aspects of the work in ensuring that questions related to the accuracy or integrity of any part of the work are appropriately investigated and resolved. The study was conducted in accordance with the Declaration of Helsinki (as revised in 2013). The study was approved by the P. Hertsen Moscow Oncology Research Institute Ethics Committee and holds Institutional Review Board approval (#062-A, 15.06.2022). Additionally, participating patients provided written informed consent, and all clinical data were anonymized.
Open Access Statement: This is an Open Access article distributed in accordance with the Creative Commons Attribution-NonCommercial-NoDerivs 4.0 International License (CC BY-NC-ND 4.0), which permits the non-commercial replication and distribution of the article with the strict proviso that no changes or edits are made and the original work is properly cited (including links to both the formal publication through the relevant DOI and the license). See: https://creativecommons.org/licenses/by-nc-nd/4.0/.
References
- Brunelli A, Beretta E, Cassivi SD, et al. Consensus definitions to promote an evidence-based approach to management of the pleural space. A collaborative proposal by ESTS, AATS, STS, and GTSC. Eur J Cardiothorac Surg 2011;40:291-7. [Crossref] [PubMed]
- Nachira D, Bertoglio P, Ismail M, et al. Are the Efficacy and Safety of Chest Tubes in Uniportal Video-Assisted Thoracic Surgery Related to the Level of Intercostal Space Insertion or to the Drain Type? A Prospective Multicenter Study. J Clin Med 2024;13:430. [Crossref] [PubMed]
- Gossot D. From uniportal to multiportal approach to major pulmonary resections: a non-evidence based debate. Video-assist Thorac Surg 2017;2:75.
- Clavien PA, Barkun J, de Oliveira ML, et al. The Clavien-Dindo classification of surgical complications: five-year experience. Ann Surg 2009;250:187-96. [Crossref] [PubMed]
- Riley RD, Snell KIE, Ensor J, et al. Minimum sample size for developing a multivariable prediction model: PART II - binary and time-to-event outcomes. Stat Med 2019;38:1276-96. [Crossref] [PubMed]
- Thandra KC, Barsouk A, Saginala K, et al. Epidemiology of lung cancer. Contemp Oncol (Pozn) 2021;25:45-52. [Crossref] [PubMed]
- ESTS Database Annual Report: Silver Book. 2022. Available online: https://www.ests.org/_userfiles/pages/files/database_reports/ests_2022_silver_book.pdf
- Zheng Q, Ge L, Zhou J, et al. Risk factors for prolonged air leak after pulmonary surgery: A systematic review and meta-analysis. Asian J Surg 2022;45:2159-67. [Crossref] [PubMed]
- Attaar A, Tam V, Nason KS. Risk Factors for Prolonged Air Leak After Pulmonary Resection: A Systematic Review and Meta-analysis. Ann Surg 2020;271:834-44. [Crossref] [PubMed]
- Gonzalez M, Karenovics W, Bédat B, et al. Performance of prolonged air leak scoring systems in patients undergoing video-assisted thoracoscopic surgery segmentectomy. Eur J Cardiothorac Surg 2022;62:ezac100. [Crossref] [PubMed]
- Johnson BH, Johnston SS, Tewari P, et al. Clinical and Economic Burden Associated with Prolonged Air Leaks Among Patients Undergoing Thoracic Resection: A Retrospective Database Analysis. Clinicoecon Outcomes Res 2023;15:269-80. [Crossref] [PubMed]
- Brunelli A, Varela G, Refai M, et al. A scoring system to predict the risk of prolonged air leak after lobectomy. Ann Thorac Surg 2010;90:204-9. [Crossref] [PubMed]
- Divisi D, Pipitone M, Perkmann R, et al. Prolonged air leak after video-assisted thoracic anatomical pulmonary resections: a clinical predicting model based on data from the Italian VATS group registry, a machine learning approach. J Thorac Dis 2023;15:849-57. [Crossref] [PubMed]
- Brunelli A, Cassivi SD, Salati M, et al. Digital measurements of air leak flow and intrapleural pressures in the immediate postoperative period predict risk of prolonged air leak after pulmonary lobectomy. Eur J Cardiothorac Surg 2011;39:584-8. [Crossref] [PubMed]
- Zaraca F, Brunelli A, Pipitone MD, et al. A Delphi Consensus report from the "Prolonged Air Leak: A Survey" study group on prevention and management of postoperative air leaks after minimally invasive anatomical resections. Eur J Cardiothorac Surg 2022;62:ezac211. [Crossref] [PubMed]
- Pawelczyk K, Marciniak M, Kacprzak G, et al. One or two drains after lobectomy? A comparison of both methods in the immediate postoperative period. Thorac Cardiovasc Surg 2007;55:313-6. [Crossref] [PubMed]
- Gao S, Zhang Z, Aragón J, et al. The Society for Translational Medicine: clinical practice guidelines for the postoperative management of chest tube for patients undergoing lobectomy. J Thorac Dis 2017;9:3255-64. [Crossref] [PubMed]
- Rivera C, Bernard A, Falcoz PE, et al. Characterization and prediction of prolonged air leak after pulmonary resection: a nationwide study setting up the index of prolonged air leak. Ann Thorac Surg 2011;92:1062-8; discussion 1068. [Crossref] [PubMed]
- Orsini B, Baste JM, Gossot D, et al. Index of prolonged air leak score validation in case of video-assisted thoracoscopic surgery anatomical lung resection: results of a nationwide study based on the French national thoracic database, EPITHOR. Eur J Cardiothorac Surg 2015;48:608-11. [Crossref] [PubMed]
- Li SJ, Zhou K, Wu YM, et al. Presence of pleural adhesions can predict conversion to thoracotomy and postoperative surgical complications in patients undergoing video-assisted thoracoscopic lung cancer lobectomy. J Thorac Dis 2018;10:416-31. [Crossref] [PubMed]
- Cangir AK, Yüksel C, Dakak M, et al. Use of intrapleural streptokinase in experimental minimal clotted hemothorax. Eur J Cardiothorac Surg 2005;27:667-70. [Crossref] [PubMed]
- Temes RT, Willms CD, Endara SA, et al. Fissureless lobectomy. Ann Thorac Surg 1998;65:282-4. [Crossref] [PubMed]