Analysis of Mycoplasma pneumoniae pneumonia and viral coinfection in southern Guangzhou after the COVID-19 pandemic
Highlight box
Key findings
• We investigate the detection rate of Mycoplasma pneumoniae (MP) and clinical features of viral coinfection in Mycoplasma pneumoniae pneumonia (MPP) after the coronavirus disease 2019 (COVID-19) pandemic in southern Guangzhou.
What is known and what is new?
• Risk factors for MPP-viral coinfection include a longer fever process, higher leukocyte counts, higher lymphocyte counts and higher C-reactive protein.
• MPP-viral coinfection was identified in both children (27.27%) and adults (5.0%) in southern Guangzhou after COVID-19 pandemic. We further analyzed the characteristics of different types of MPP-viral coinfection and found that fever and elevated procalcitonin of greater than 5 ng/mL were the risk factors.
What is the implication, and what should change now?
• Increase in procalcitonin should be considered as a possibility of viral coinfection in children with MPP. This finding suggests the need for viral test in children with elevated procalcitonin in MPP, which could help clinicians identify viral coinfection at an early stage and provide timely antiviral intervention.
Introduction
Mycoplasma pneumoniae (MP) is a leading cause of community-acquired pneumonia (CAP) requiring hospitalization in both children and adults, accounting for approximately 10–40% of all CAP (1-4). Non-pharmaceutical interventions (NPIs) were introduced to reduce the spread of severe acute respiratory syndrome coronavirus 2 (SARS-CoV-2) during the coronavirus disease 2019 (COVID-19) pandemic, including the use of masks, respiratory etiquette, improved hygiene, physical distance measures, stay-at-home orders, etc. (5). Meanwhile, the implementation of NPIs also reduced the transmission of other respiratory infection such as MP, influenza virus (IFV) and respiratory syncytial virus (RSV) (6-9). There was a significant decline in MP incidence from 8.61% to 1.69% after implementation of NPIs according to results of a global survey (10). Since there was no COVID-19 restriction in 2023, there had been an epidemic of multiple respiratory infections in China. Sentinel hospitals have reported an increase in respiratory infections other than the novel coronavirus disease, including influenza, MP and RSV. An increase of MP was observed in CAP recently, and some patients were simultaneously infected with other respiratory pathogens at the same time. Three years of COVID-19 measures might be associated with this increasing population susceptibility to respiratory infections. The main objective of this study was to investigate the detection rate of MP and viral coinfection in southern Guangzhou after the COVID-19 epidemic, in hopes of discovering prevalence changes of the common respiratory pathogens following the loosening of pandemic measures. Further, since respiratory epidemic varied among regions, the pathogen results of this study would provide information on etiology of CAP in southern Guangzhou. We present this article in accordance with the STROBE reporting checklist (available at https://jtd.amegroups.com/article/view/10.21037/jtd-24-729/rc).
Methods
Study design and setting
This retrospective study was conducted at the Respiratory Medicine and Pediatrics Department of the Second Affiliated Hospital of Guangzhou Medical University Panyu Branch. We reviewed all patients who diagnosed with respiratory infection, and subsequently underwent MP and respiratory viral testing from October 2023 to January 2024. We excluded patients with compromised immune function, such as cancer patients, transplant patients, AIDS patients, hematologic malignancies, and rheumatic patients. Patients diagnosed with hospital-acquired pneumonia more than 48 hours after hospitalization were also excluded from this study. A total of 3,094 patients were included in this analysis, 886 patients were tested to be positive with MP, and among them 384 patients were diagnosed with Mycoplasma pneumoniae pneumonia (MPP) (Figure 1). The study was conducted in accordance with the Declaration of Helsinki (as revised in 2013). This study was approved by the Institution Ethics Board of the Second Affiliated Hospital of Guangzhou Medical University (No. 2024-hg-ks-30). All the patients provided their written informed consent for the retrospective evaluation of their data.
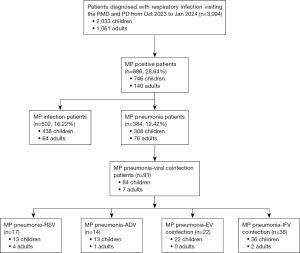
Data including basic patient information, duration of symptom onset, hospital admission, clinical symptoms and signs, laboratory findings, chest imaging feature were collected by reviewing medical records, and entering into a predesigned database by experienced clinicians. Nasopharyngeal or oral swabs samples were collected for viral pathogens testing.
Detection of MP and other pathogens
MP was confirmed by MP serologic tests (SERODIA-MYCO II, FUJIREBIO, FuJi, Japan) as well as polymerase chain reaction (PCR) of sputum or nasopharyngeal swab samples using a MP PCR fluorescence diagnostic kit (TIANLONG, Suzhou, China). The specific immunoglobulin M (IgM) antibodies against MP, RSV, adenovirus (ADV) and enterovirus (EV) were also detected using a commercial enzyme-linked immunosorbent assay (ELISA) kit (PNEUMOSLIDE IgM, VIRCELL, Spain). A positive IgM antibody reaction was defined as >1.1 S/CO. Nasopharyngeal or oral swab samples were obtained from each patient after admission to detect influenza A (IV-A) and influenza B (IV-B) (without subtype information). Common viruses including RSV, ADV and EV were detected by immunofluorescence tests or PCR assay. Each positive result was reviewed by at least one physician. Viral coinfection in MPP was determined if test results were positive for more than one of the respiratory viruses previously mentioned.
Statistical analysis
Data were entered into Microsoft Excel and analyzed using SPSS v.23.0 (IBM Corp., NY, USA). Values were presented as frequencies with proportions for categorical variables and median with interquartile ranges (IQRs) for continuous variables. The Fisher’s exact test was performed to compare differences between categorical variables. The Mann-Whitney U-test was used to compare the continuous variables. P<0.05 was considered as a significant difference.
Results
Study population
A total of 3,094 patients were included in this analysis, 886 (28.64%) patients were tested positive with MP, and among them 384 (12.42%) patients were diagnosed with MPP. MP infection was identified in 746 (36.69%) children and 140 (13.20%) adults. Among them, there were 308 (41.29%) children and 76 (54.29%) adults with MPP. Tests for common respiratory viruses including RSV, ADV, EV and IFV were performed to detect viral coinfection in MPP. 84 children (27.27%) and 7 adults (5%) with MPP were found to be coinfected with viral pathogens.
Age distribution of MP and IFV infection in autumn and winter after COVID-19 pandemic
Among the patients with respiratory infection in autumn and winter in southern Guangzhou, MP was identified highest in all age groups, especially in preschool children. The MP detection rate of preschool children aged 0–3 and 4–6 years was 41.78% and 37.46%, respectively. The MP infection rate of patients aged 41–60 years was the lowest, and it was slightly increased to 12.09% in patients over 60 years. We routinely tested for IFV as it was also high season for influenza. Influenza was identified in 22.2% of children, which was significantly higher than that in adults of 3.77% (Figure 2).
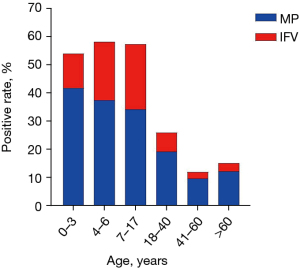
Temporal distribution of MP and IFV infection in autumn and winter after COVID-19 pandemic
MP infection was found in 40.09% of children in October 2023, and then gradually declined but remained at a high level in the following months. While it was observed in 18.25% of adults in November 2023, which was significantly lower than that of children. In December, Influenza was identified in 27.62% of children, while it was significantly lower in adults with a positive rate of 4.47% (Figure 3).
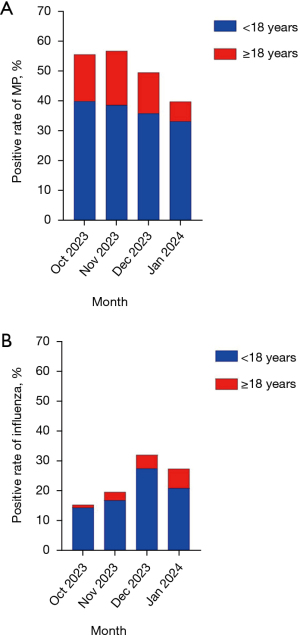
Distinction between MPP with and without viral coinfection in children
The most common identified virus in children with MPP was IFV (11.68%), follow by EV (7.14%), RSV (4.22%) and ADV (4.22%) (Figure 4). Significant differences were observed in fever (P=0.03), white blood cell count (P=0.03), C-reactive protein (P=0.02), procalcitonin (P=0.02), lactate dehydrogenase (LDH) (>250 U/L) (P=0.04) between single MPP and viral coinfections. There was no statistically significant difference in age (P=0.13), gender (P=0.20), hospital stay (P=0.44), pulse rate (P=0.64), respiratory rate (P=0.30), lymphocyte count (P=0.22), red blood cell count (P=0.41), platelet count (P=0.53), hemoglobin (P=0.47), serum amyloid (P=0.62), serum LDH (P=0.33), alanine aminotransferase (ALT) (P=0.40), aspartate aminotransferase (AST) (P=0.28) and creatine (P=0.46) between patients with single infections and those with viral coinfection (Table 1).
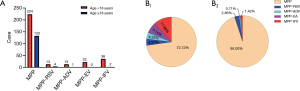
Table 1
Characteristics | MPP (n=224) | MPP-viral coinfection (n=84) | |||||||||
---|---|---|---|---|---|---|---|---|---|---|---|
Total (n=84) | P1 | RSV coinfection (n=13) | P2 | ADV coinfection (n=13) | P3 | EV coinfection (n=22) | P4 | IFV coinfection (n=36) | P5 | ||
Age (year) | 6 [3–7] | 4 [3–7] | 0.13 | 3 [2–5] | 0.02 | 4 [3–6] | 0.17 | 6 [3–9] | 0.40 | 4 [3–7] | 0.22 |
Male/female ratio | 108/116 | 48/36 | 0.20 | 6/7 | 1.00 | 6/7 | 1.00 | 15/7 | 0.12 | 21/15 | 0.37 |
Fever (%) | 197 (87.95) | 81 (96.43) | 0.03 | 13 (100.00) | 0.37 | 12 (92.31) | 1.00 | 21 (95.45) | 0.48 | 35 (97.22) | 0.14 |
Fever process (days) | 6 [5–8] | 6 [5–8] | 0.27 | 6 [5–8] | 0.57 | 7 [6–8] | 0.69 | 7 [6–9] | 0.26 | 7 [6–8] | 0.62 |
Peak temperature (℃) | 39.0 [38.9–39.8] | 39.0 [38.8–39.9] | 0.84 | 38.5 [38.1–39.8] | 0.12 | 39.8 [38.7–40.0] | <0.001 | 39.0 [39.0–39.8] | 0.67 | 39.0 [39.0–39.8] | 0.91 |
Hospital stay (day) | 7 [7–9] | 7 [6–8] | 0.44 | 8 [7–9] | 0.78 | 8 [7–8] | 0.03 | 7 [6–8] | 0.24 | 6 [5–8] | 0.10 |
Pulse rate (times/min) | 110 [102–115] | 110 [102–118] | 0.64 | 112 [102–118] | 0.44 | 110 [104–124] | 0.44 | 110 [102–120] | 0.57 | 110 [103–118] | 0.54 |
Respiratory rate (times/min) | 24 [22–28] | 24 [22–28] | 0.30 | 26 [23–29] | 0.01 | 24 [22–27] | 0.58 | 24 [21–30] | 0.36 | 25 [23–27] | 0.95 |
Blood oxygen saturation (%) | 98 [98–99] | 98 [98–99] | 0.07 | 98 [98–99] | 0.08 | 98 [98–99] | 0.37 | 98 [98–99] | 0.47 | 98 [98–99] | 0.17 |
Laboratory findings | |||||||||||
White blood cell (×109/L) | 7.91 [6.18–10.03] | 8.02 [5.73–11.34] | 0.03 | 9.10 [6.98–11.93] | 0.03 | 7.50 [6.12–21.73] | 0.002 | 7.42 [5.48–11.65] | 0.25 | 7.78 [5.05–10.61] | 0.91 |
<4×109/L (%) | 7 (3.13) | 6 (7.14) | 0.20 | 0 (0.00) | 1.00 | 0 (0.00) | 1.00 | 1 (4.55) | 1.00 | 5 (13.89) | 0.14 |
>10×109/L (%) | 53 (23.66) | 32 (38.10) | 0.02 | 5 (38.46) | 0.32 | 5 (38.46) | 0.32 | 9 (40.91) | 0.12 | 13 (36.11) | 0.15 |
Lymphocyte count (×109/L) | 2.19 [1.51–3.25] | 2.57 [1.74–3.39] | 0.22 | 3.98 [3.30–5.99] | <0.001 | 2.74 [1.68–3.37] | 0.79 | 1.89 [1.31–2.70] | 1.00 | 2.28 [1.61–3.24] | 0.93 |
<1.1×109/L (%) | 14 (6.25) | 6 (7.15) | 0.80 | 0 (0.00) | 1.00 | 0 (0.00) | 1.00 | 3 (13.64) | 0.19 | 3 (8.33) | 0.71 |
>3.2×109/L (%) | 55 (24.55) | 28 (33.33) | 0.15 | 11 (84.62) | <0.001 | 4 (30.77) | 0.74 | 4 (18.18) | 0.61 | 9 (25.00) | 1.00 |
Red blood cell (×109/L) | 4.45 [4.22–4.75] | 4.53 [4.26–4.89] | 0.41 | 4.49 [4.07–4.89] | 0.81 | 4.72 [4.40–5.11] | 0.21 | 4.48 [4.27–4.86] | 0.94 | 4.53 [4.25–4.88] | 0.56 |
Hemoglobin (g/L) | 122 [116–128] | 121 [113–131] | 0.47 | 119 [103–127] | 0.26 | 119 [107–130] | 0.69 | 119 [112–131] | 0.25 | 126 [113–133] | 0.26 |
Platelet (×1012/L) | 277 [219–364] | 279 [224–387] | 0.53 | 395 [321–455] | 0.01 | 250 [208–372] | 0.55 | 273 [225–401] | 0.64 | 260 [220–345] | 0.50 |
C-reactive protein (mg/L) | 8.71 [2.94–20.50] | 9.82 [6.44–28.07] | 0.02 | 8.27 [6.64–24.02] | 0.67 | 17.60 [11.02–29.95] | 0.01 | 14.32 [6.48–31.68] | 0.09 | 7.80 [4.84–15.77] | 0.63 |
>10 mg/L (%) | 97 (43.30) | 36 (42.86) | 1.00 | 4 (30.77) | 0.57 | 9 (69.23) | 0.09 | 12 (54.55) | 0.37 | 11 (30.56) | 0.20 |
Serum amyloid (mg/L) | 43.77 [9.94–95.46] | 36.74 [16.72–85.57] | 0.62 | 42.80 [16.65–111.53] | 0.83 | 46.98 [10.53–127.79] | 0.67 | 41.94 [9.14–82.63] | 0.71 | 32.14 [18.47–77.03] | 0.32 |
>10 mg/L (%) | 122 (54.46) | 54 (64.29) | 0.16 | 8 (61.54) | 0.09 | 8 (61.54) | 0.09 | 13 (59.09) | 0.49 | 25 (69.44) | 0.065 |
Procalcitonin (ng/mL) | 0.16 [0.10–0.26] | 0.19 [0.11–0.48] | 0.02 | 0.18 [0.10–0.43] | 0.02 | 0.39 [0.14–1.38] | 0.001 | 0.13 [0.10–0.42] | 0.09 | 0.20 [0.12–0.36] | 0.10 |
>0.5 ng/mL (%) | 19 (8.48) | 16 (19.05) | 0.02 | 2 (15.38) | 0.32 | 5 (38.46) | 0.005 | 3 (13.64) | 0.43 | 6 (16.67) | 0.13 |
LDH (U/L) | 262 [230–307] | 269 [237–314] | 0.33 | 266 [235–324] | 0.95 | 299 [268–351] | 0.16 | 265 [226–313] | 0.99 | 266 [237–314] | 0.98 |
>250 U/L (%) | 128 (57.14) | 59 (70.24) | 0.04 | 10 (76.92) | 0.10 | 12 (92.31) | 0.009 | 14 (63.54) | 0.38 | 23 (63.89) | 0.22 |
AST (U/L) | 31 [27–40] | 36 [27–42] | 0.28 | 33 [27–46] | 0.58 | 38 [29–49] | 0.29 | 32 [26–38] | 0.15 | 37 [29–44] | 0.16 |
ALT (U/L) | 13 [10–17] | 12 [9–16] | 0.40 | 12 [11–17] | 0.52 | 15 [13–17] | 0.40 | 14 [11–17] | 0.30 | 13 [10–18] | 0.15 |
>50 U/L (%) | 2 (0.89) | 3 (3.57) | 0.13 | 0 (0.00) | 1.00 | 1 (7.69) | 0.16 | 0 (0.00) | 1.00 | 2 (5.56) | 0.09 |
Serum creatinine (μmol/L) | 47.3 [40–55] | 46.0 [37.7–52.2] | 0.46 | 46 [37–47] | 0.05 | 47 [37–53] | 0.87 | 47 [37–53] | 0.56 | 45 [39–53] | 0.98 |
Data are shown as n (%) or median [interquartile range]. P values were calculated using the Mann-Whitney U test or Fisher’s exact test. P1: MPP vs. MPP-viral coinfection; P2: MPP vs. MPP-RSV; P3: MPP vs. MPP-ADV; P4: MPP vs. MPP-EV; P5: MPP vs. MPP-IFV. MPP, Mycoplasma pneumoniae pneumonia; LDH, lactate dehydrogenase; AST, aspartate aminotransferase; ALT, alanine aminotransferase; RSV, respiratory syncytial virus; ADV, adenovirus; EV, enterovirus; IFV, influenza virus.
Moreover, we also compared the viral coinfection subgroup with single MPP. There was no significant difference in fever proportion and fever process among all groups, but the body temperature was the highest in the MPP-RSV coinfection group with a median of 39.8 ℃. White blood cell of the MPP-RSV (P=0.03) and MPP-ADV (P=0.002) coinfection group was higher than that of the single MP. The increased in platelet count (P=0.01) and proportion of elevated lymphocyte (P<0.001) were observed in patients with MPP-RSV coinfection. Elevated procalcitonin level was observed in the MPP-RSV (P=0.02) and MPP-ADV (P=0.001) coinfection groups, rather than the MPP-EV (P=0.09) and MPP-IFV (P=0.10) coinfection group. Each group exhibited no significant difference in LDH and serum amyloid level, but the proportion of patients with LDH greater than 250 U/L was significantly increased in the MPP-ADV coinfection group (P=0.009).
Lung imaging characteristics compared with MP pneumonia and viral coinfection in children
Seventy-three (32.59%) patients in the single MPP group and 36 (42.86%) patients in the viral coinfection group underwent chest computed tomography (CT) examination. There was no significant difference in location, patchy shadowing, consolidation, air bronchogram sign and pleural effusion between these two groups (Figure 5). However, children with viral coinfection appeared to have a higher proportion of diffuse large area of inflammation (Table 2).
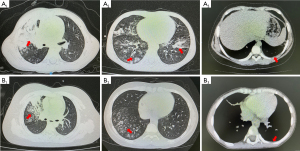
Table 2
Variable | Total (n=308) | MPP (n=224) | MPP-viral coinfection (n=84) | P |
---|---|---|---|---|
Single lung | 127 (41.23) | 85 (37.95) | 42 (50.00) | 0.07 |
Bilateral lung | 181 (58.77) | 139 (62.05) | 42 (50.00) | 0.07 |
Patchy shadowing | 298 (96.75) | 215 (95.98) | 83 (98.81) | 0.30 |
Consolidation | 49 (15.91) | 32 (14.29) | 17 (20.24) | 0.22 |
Air bronchogram sign | 42 (13.64) | 25 (11.16) | 17 (20.24) | 0.06 |
Pleural fluid | 9 (2.92) | 6 (2.68) | 3 (3.57) | 0.25 |
Data are shown as n (%). P values were calculated using the Chi-square test or Fisher’s exact test. MPP, Mycoplasma pneumoniae pneumonia.
Significant associations with viral coinfection in children with MPP
Table 3 shows the significant associations of viral coinfection in children with MPP. Multivariable analysis indicated that fever (adjusted odds ratio, 3.46; 95% confidence interval: 1.02–11.74; P=0.047) and elevated procalcitonin greater than 5 ng/mL (adjusted odds ratio, 3.17; 95% confidence interval: 1.50–6.72; P=0.003) were strong predisposing factors for viral coinfection (Table 3).
Table 3
Variable | Adjusted ORs with 95% CI |
P |
---|---|---|
Fever | 3.46 (1.02–11.74) | 0.047 |
Procalcitonin (>5 ng/mL) | 3.17 (1.50–6.72) | 0.003 |
MPP, Mycoplasma pneumoniae pneumonia; OR, odds ratio; CI, confidence interval.
Discussion
During the COVID-19 pandemic, the detection rate of respiratory pathogens decreased mainly due to the implementation of NPIs (5,9). However, multiple respiratory pathogens have emerged after the COVID-19 pandemic, and some people can be infected with one or more respiratory pathogens at the same time or in close period. Many studies have reported the clinical characteristics and risk factors of patients with MP and bacterial and viral coinfection before and during COVID-pandemic (11-14). However, there has been no specific analysis of different types of viral coinfection. This study is the first to analyze the detection rate of MP in adults and children in southern Guangzhou since 2023 without the COVID-19 restrictions, and further analyze the clinical characteristics of different types of MPP-viral coinfection.
Similar to previous studies, we found that MP infection was at a high level in autumn and winter in 2023, with a detection rate ranging from 33.33% to 40.09% in children. However, the detection rate of MP in adults was significantly lower, ranging from 6.62–12.85%. In terms of age distribution, the highest detection rate of MP in patients of 0–3 years old was 41.78%, while the lowest detection rate in patients with 41–60 years was 9.49%. However, it slightly increased in patients over 60 years old with percentage of about 12.09%. We also analyzed the detection rate of other respiratory viruses in patients with MPP including RSV, ADV, EV and IFV, and the positive rate of viral coinfection was 27.27%. RSV coinfection was detected in 4.22% of children with MPP, and it was more common in younger children, which may be due to the immature immune systems making it more susceptible to respiratory pathogens (15). It has been reported that the most common pathogen of virus co-infective with MP is RSV before COVID-19 pandemic (3). However, in our study, IFV was found to be the most common type of viral coinfection after COVID-19 pandemic without restrictions in southern Guangzhou, with the highest detection rate of 11.68%. This could be related to the high incidence of influenza at the same time. We further analyzed the characteristics of different types of MPP-virus coinfection. The median age of patients with MPP-RSV coinfection was the youngest in all groups, and respiratory rate, white blood cell count, lymphocyte count, platelet count and procalcitonin were higher in MPP-RSV coinfection than single MPP. The body temperature was the highest in MPP-ADV coinfection, and the white blood cell, CRP, procalcitonin and LDH were higher than single MP pneumonia. This indicated that there was a stronger systemic inflammatory response in patients with viral coinfection. There was no significant difference in clinical features and related laboratory test in MP pneumonia with EV and IFV coinfection. The proportion of elevated serum amyloid (>10 mg/L) in the MPP-IFV group was increased, but the other indicators were not significantly different from those in the MPP group, which was similar to the results reported by Kim et al. (16). Previous studies have found that elevated liver enzyme levels were more commonly observed in respiratory viral infection at a younger age (17), and the LDH level increased during viral coinfection in MPP (18). In our study, we found that there was no significant difference in LDH, AST and ALT level among the viral coinfection groups, but the proportion of LDH (>250 U/L) was significantly increased in the viral coinfection group. PCT was a useful biomarker for distinguishing bacterial and for identifying severe bacterial infection. However, Gautam et al. have shown that procalcitonin was also elevated in pure viral infection in the absence of bacterial pneumonia (19). Respiratory viral infection could lead to disruption of the normal microbial community, which contributed to the development of post-viral bacterial pneumonia (20). Infectious indicators including white blood cell, C-reactive protein and serum PCT were all increased in MPP with viral coinfection in our study, suggesting that viral infections might aggravate the primary MP infection or predispose patients to secondary bacterial infections (21).
Similar to previous study, consolidation and air bronchogram sign were observed in both single MPP and viral coinfection group (22). The severe airway damage and enhanced inflammatory response caused by the viral coinfection could lead to refractory MP pneumonia or even necrotizing pneumonia (23,24).
However, it must be acknowledged that there are certain limitations in this study. Firstly, it is a retrospective study, which appears to have a selection bias, and some respiratory pathogens including rhinovirus, parainfluenza virus and metapneumovirus have not been routinely tested. Secondly, the distribution of patients among the five groups could not be balanced, as the number of cases in each viral coinfection group was not very high. Thus, research on incorporating larger datasets is required to be carried out in future studies.
Conclusions
In conclusion, an increase in MP infections was observed in both children and adults with respiratory infection in southern Guangzhou after the COVID-19 pandemic. Viral coinfection in MPP has been common in children. Fever and elevated procalcitonin (>5 ng/mL) were significantly associated with viral coinfection in children with MPP. Increase in procalcitonin should be considered as a possibility of viral coinfection in MPP. This finding suggests the need for viral testing for MPP with elevated procalcitonin, which could help clinicians identify viral coinfection at an early stage and provide timely antiviral intervention.
Acknowledgments
Funding: This study was supported by
Footnote
Reporting Checklist: The authors have completed the STROBE reporting checklist. Available at https://jtd.amegroups.com/article/view/10.21037/jtd-24-729/rc
Data Sharing Statement: Available at https://jtd.amegroups.com/article/view/10.21037/jtd-24-729/dss
Peer Review File: Available at https://jtd.amegroups.com/article/view/10.21037/jtd-24-729/prf
Conflicts of Interest: All authors have completed the ICMJE uniform disclosure form (available at https://jtd.amegroups.com/article/view/10.21037/jtd-24-729/coif). The authors report the funding from the Plan on Enhancing Scientific Research in GMU (No. 0803030072) and Guangdong Medical Science and Technology Research Fund (No. A2024432). The authors have no other conflicts of interest to declare.
Ethical Statement: The authors are accountable for all aspects of the work in ensuring that questions related to the accuracy or integrity of any part of the work are appropriately investigated and resolved. The study was conducted in accordance with the Declaration of Helsinki (as revised in 2013). The study protocol was reviewed and approved by the Institution Ethics Board of the Second Affiliated Hospital of Guangzhou Medical University
Open Access Statement: This is an Open Access article distributed in accordance with the Creative Commons Attribution-NonCommercial-NoDerivs 4.0 International License (CC BY-NC-ND 4.0), which permits the non-commercial replication and distribution of the article with the strict proviso that no changes or edits are made and the original work is properly cited (including links to both the formal publication through the relevant DOI and the license). See: https://creativecommons.org/licenses/by-nc-nd/4.0/.
References
- Jain S, Williams DJ, Arnold SR, et al. Community-acquired pneumonia requiring hospitalization among U.S. children. N Engl J Med 2015;372:835-45. [Crossref] [PubMed]
- Atkinson TP, Balish MF, Waites KB. Epidemiology, clinical manifestations, pathogenesis and laboratory detection of Mycoplasma pneumoniae infections. FEMS Microbiol Rev 2008;32:956-73. [Crossref] [PubMed]
- Lee E, Kim CH, Lee YJ, et al. Annual and seasonal patterns in etiologies of pediatric community-acquired pneumonia due to respiratory viruses and Mycoplasma pneumoniae requiring hospitalization in South Korea. BMC Infect Dis 2020;20:132. [Crossref] [PubMed]
- Juvén T, Mertsola J, Waris M, et al. Etiology of community-acquired pneumonia in 254 hospitalized children. Pediatr Infect Dis J 2000;19:293-8. [Crossref] [PubMed]
- Cowling BJ, Ali ST, Ng TWY, et al. Impact assessment of non-pharmaceutical interventions against coronavirus disease 2019 and influenza in Hong Kong: an observational study. Lancet Public Health 2020;5:e279-88. [Crossref] [PubMed]
- Huang QS, Wood T, Jelley L, et al. Impact of the COVID-19 nonpharmaceutical interventions on influenza and other respiratory viral infections in New Zealand. Nat Commun 2021;12:1001. [Crossref] [PubMed]
- von Hammerstein AL, Aebi C, Barbey F, et al. Interseasonal RSV infections in Switzerland - rapid establishment of a clinician-led national reporting system (RSV EpiCH). Swiss Med Wkly 2021;151:w30057. [Crossref] [PubMed]
- Haapanen M, Renko M, Artama M, et al. The impact of the lockdown and the re-opening of schools and day cares on the epidemiology of SARS-CoV-2 and other respiratory infections in children - A nationwide register study in Finland. EClinicalMedicine 2021;34:100807. [Crossref] [PubMed]
- Olsen SJ, Winn AK, Budd AP, et al. Changes in influenza and other respiratory virus activity during the COVID-19 pandemic-United States, 2020-2021. Am J Transplant 2021;21:3481-6. [Crossref] [PubMed]
- Meyer Sauteur PM, Beeton ML, Uldum SA, et al. Mycoplasma pneumoniae detections before and during the COVID-19 pandemic: results of a global survey, 2017 to 2021. Euro Surveill 2022;27:2100746. [Crossref] [PubMed]
- Song Q, Xu BP, Shen KL. Effects of bacterial and viral co-infections of mycoplasma pneumoniae pneumonia in children: analysis report from Beijing Children's Hospital between 2010 and 2014. Int J Clin Exp Med 2015;8:15666-74.
- Lim YK, Kweon OJ, Kim HR, et al. Impact of bacterial and viral coinfection in community-acquired pneumonia in adults. Diagn Microbiol Infect Dis 2019;94:50-4. [Crossref] [PubMed]
- Liu YN, Zhang YF, Xu Q, et al. Infection and co-infection patterns of community-acquired pneumonia in patients of different ages in China from 2009 to 2020: a national surveillance study. Lancet Microbe 2023;4:e330-9. [Crossref] [PubMed]
- Choo S, Lee YY, Lee E. Clinical significance of respiratory virus coinfection in children with Mycoplasma pneumoniae pneumonia. BMC Pulm Med 2022;22:212.
- Chen Q, Lin L, Zhang N, et al. Adenovirus and Mycoplasma pneumoniae co-infection as a risk factor for severe community-acquired pneumonia in children. Front Pediatr 2024;12:1337786. [Crossref] [PubMed]
- Kim JH, Kwon JH, Lee JY, et al. Clinical features of Mycoplasma pneumoniae coinfection and need for its testing in influenza pneumonia patients. J Thorac Dis 2018;10:6118-27. [Crossref] [PubMed]
- Oh JS, Choi JS, Lee YH, et al. The Relationships between Respiratory Virus Infection and Aminotransferase in Children. Pediatr Gastroenterol Hepatol Nutr 2016;19:243-50. [Crossref] [PubMed]
- Zhang C, Zhang Q, Du JL, et al. Correlation Between the Clinical Severity, Bacterial Load, and Inflammatory Reaction in Children with Mycoplasma Pneumoniae Pneumonia. Curr Med Sci 2020;40:822-8. [Crossref] [PubMed]
- Gautam S, Cohen AJ, Stahl Y, et al. Severe respiratory viral infection induces procalcitonin in the absence of bacterial pneumonia. Thorax 2020;75:974-81. [Crossref] [PubMed]
- Hanada S, Pirzadeh M, Carver KY, et al. Respiratory Viral Infection-Induced Microbiome Alterations and Secondary Bacterial Pneumonia. Front Immunol 2018;9:2640. [Crossref] [PubMed]
- Simon L, Gauvin F, Amre DK, et al. Serum procalcitonin and C-reactive protein levels as markers of bacterial infection: a systematic review and meta-analysis. Clin Infect Dis 2004;39:206-17. [Crossref] [PubMed]
- Huang X, Gu H, Wu R, et al. Chest imaging classification in Mycoplasma pneumoniae pneumonia is associated with its clinical features and outcomes. Respir Med 2024;221:107480. [Crossref] [PubMed]
- Zhang X, Sun R, Jia W, et al. Clinical Characteristics of Lung Consolidation with Mycoplasma pneumoniae Pneumonia and Risk Factors for Mycoplasma pneumoniae Necrotizing Pneumonia in Children. Infect Dis Ther 2024;13:329-43. [Crossref] [PubMed]
- Zhang X, Chen Z, Gu W, et al. Viral and bacterial co-infection in hospitalised children with refractory Mycoplasma pneumoniae pneumonia. Epidemiol Infect 2018;146:1384-8. [Crossref] [PubMed]