The effect of bronchoscopic lung volume reduction on the bronchodilator response
Highlight box
Key findings
• Preliminary data signal that bronchoscopic lung volume reduction (BLVR) may have an impact on bronchodilator response (BDR).
What is known and what is new?
• Landmark studies in BLVR report an increase in post bronchodilator forced expiratory volume in the first second with the procedure.
• The effect on BDR is unknown.
• This retrospective analysis of 20 patients with successful BLVR suggests there may be an impact on the BDR.
What is the implication, and what should change now?
• Further prospective and physiologic studies are required to explore the relationship between BLVR and BDR.
Introduction
Bronchoscopic lung volume reduction (BLVR) is an established intervention for the treatment of advanced chronic obstructive pulmonary disease (COPD) in select patients. The endobronchial approach provides a less invasive method to lung volume reduction surgery which is associated with significant morbidity and mortality. The procedure entails the bronchoscopic placement of one-way valves within the bronchi of severely emphysematous portions of the lung to achieve complete collapse (1). The physiologic changes that occur following BLVR include restoring the diaphragmatic position and thus enhancing the zone of apposition and increasing both forced vital capacity (FVC) and the forced expiratory volume within the first second (FEV1) (2). Investigations measuring the efficacy of BLVR have focused on several parameters including pulmonary function test (PFT), exercise capacity (6-minute walk test), and quality of life (Saint George’s Respiratory Questionnaire) (3).
In nearly all major studies investigating the efficacy of BLVR, the improvement in the FEV1 has been primarily reported based on the comparison of the postbronchodilator value before and after valve placement (4). The difference between the prebronchodilator and post-bronchodilator FEV1, termed the bronchodilator response (BDR), is typically not assessed before and after valve placement (5). However, given the heterogeneity of beta-2 receptor location and non-uniform distribution of aerosolized particles, the placement of endobronchial valves may alter the BDR (6,7). The collapse of portions of the lung with significant emphysema, and thus a reduction in dead space, may result in rerouting inhaled aerosolized particles to healthier areas of the lung, leading to a more robust response.
We, therefore, hypothesize that following successful BLVR, aerosolized inhaled bronchodilator particles may redistribute to other areas of the lung leading to an improvement in the BDR. We performed a retrospective study comparing the BDR before and after BLVR. This manuscript is written in accordance with the STROBE reporting checklist (available at https://jtd.amegroups.com/article/view/10.21037/jtd-24-717/rc).
Methods
We performed a retrospective study to assess the effect of BLVR on the PFT BDR. The study received an institutional review board exemption. All patients who underwent BLVR between December 2019 and August 2023 were assessed.
Patient eligibility for BLVR included those with persistent symptoms despite maximal medical treatment, body mass index (BMI) less than 35 kg/m2, cigarette smoking cessation for at least 4 months, completion of pulmonary rehabilitation within the past 2 years, or willingness to enroll. Additionally, patients required a transthoracic echocardiogram, arterial blood gas while breathing ambient air, and a 6-minute walk test with an ability to ambulate between 100–500 m. A pre-procedure PFT required the following parameters which included an FEV1 of 15–45% predicted, total lung capacity (TLC) >100% predicted, residual volume (RV) >150% predicted, and diffusion capacity of the lungs for carbon monoxide (DLCO) >20% predicted.
All qualified participants also required a high-resolution computed tomography with evidence of heterogenous emphysema with more than a 40% emphysema destruction score in the target lobe (assessed at −910 Hounsfield units or lower) and more than 10% disease emphysema severity difference compared to the ipsilateral lobe. The StratXTM lung analysis platform (Thirona LungQ, Veriosn 1.0, Pulmonx, USA) was utilized to assess emphysema destruction as well as fissure completeness. A fissure completeness score of more than 90% on the right side and 80% on the left side was required to be considered. All patients were evaluated by the performing pulmonologist prior to the procedure. Following the procedure, all patients received a high-resolution CT scan at 45 days post-valve placement to assess for radiologic complete lobar atelectasis. Patients who did not achieve total lung volume reduction (TLVR) were excluded. Patients were also excluded if they required removal of the valves, did not perform PFTs before or after BLVR, or if the PFTs did not meet the American Thoracic Society criteria for acceptability and reproducibility.
Spirometry test
PFTs were performed by skilled respiratory therapists using current guidelines per European Respiratory Society/American Thoracic Society (ERS/ATS) protocol. At least three acceptable maneuvers were required to demonstrate validity. Aerosolized medications including inhaled corticosteroids (ICSs), long-acting beta-agonists (LABAs), long-acting muscarinic antagonists (LAMAs) were noted and patients were instructed to avoid the use of bronchodilators prior to undergoing PFT for the recommended timeframe (6–12 hours for short-acting bronchodilator medications and 24–48 hours for long-acting/ultra-long-acting bronchodilator medications). Post-bronchodilator testing was performed after administering 2.5 mg albuterol in 3 mL normal saline via nebulizer per bronchodilator protocol. A significant BDR was defined based on the criteria proposed by the ERS/ATS task force which recommended an improvement in FEV1 of >12% and 200 mL. We also reviewed all patient charts to assess for the presence of asthma/COPD overlap syndrome (ACOS). This was based on a combination of a history of diagnosed asthma, reversibility on spirometry, presence of eosinophilia, known allergies, and immunoglobulin E (IgE) levels. Lung volumes were determined by plethysmography and the DLCO was performed using single-breath methods with reported percent predicted values adjusted for hemoglobin. The values were reported based on the percent predicted when adjusted for age, height, and BMI.
Procedure protocol
During BLVR, patients were again assessed by bronchoscopy for collateral circulation using the ChartisTM Pulmonary Assessment System (Pulmonx Inc., Redwood City, CA, USA), and endobronchial valves were placed if there was no evidence of collateral ventilation after balloon inflation. Patients remained in the intensive care units for a minimum of 3 days post-procedure for close monitoring of complications, in particular, pneumothorax.
Statistical analysis
We compared the difference in the BDR before and after BLVR. A PFT was performed within 6 months before undergoing BLVR. The BDR from this PFT was used as the control for each patient. The mean BDR for all patients, including those that demonstrated a positive or negative value at baseline was determined. A separate mean was determined for patients with a baseline positive BDR before valve placement. A second PFT was performed within 6 months following BLVR and after ensuring that complete collapse of the target lobe was achieved. The mean BDR was also calculated for all patients and those who only demonstrated a baseline positive response prior to BLVR. All values were reported in mL. The difference between the BDR before and after valve placement was calculated for all patients who qualified and overall reported as the mean (in mL) with P values calculated. A comparison between both groups was performed using a t-test through Stata/BE 18.0 (StataCorp, TX, USA).
Ethical statement
The study was conducted in accordance with the Declaration of Helsinki (as revised in 2013). The study was approved by the ethics board of Saint Luke’s University Health Network (FWA 00003557. No. SLIR 2023-28) and individual consent for this retrospective analysis was waived.
Results
A total of 35 patients were assessed for the study. Of these, 15 patients were excluded (9 patients due to not achieving complete lobar collapse, 3 due to requiring removal of the valves, 1 due to non-reliable post-procedure PFTs, 1 due to no pre-procedure bronchodilator assessment, and 1 due to no post-valve PFT obtained). The 20 remaining patients underwent PFT before and after BLVR which included assessing the BDR. All patients were noted to be on ICS/LABA/LAMA combination therapy prior to valve placement. The mean time of the pre- and post-PFTs from the procedure time was 96±69 and 57±25 days, respectively. Endobronchial valve placement occurred in the left upper lobe in 11/20 patients, combined right upper and right middle lobe in 3/20 patients, and left lower lobe in 6/20 patients. Table 1 summarizes the demographics for all patients.
Table 1
Characteristic | Value |
---|---|
Patients | 20 (100.0) |
Age (years) | 69±5.3 |
Male | 12 (60.0) |
GOLD COPD classification | |
B | 7 (35.0) |
D | 13 (65.0) |
Target lobe | |
Left upper lobe | 11 (55.0) |
Right upper and middle lobe | 3 (15.0) |
Left lower lobe | 6 (30.0) |
Data are presented as n (%) or mean ± SD. GOLD, Global Initiative for Chronic Obstructive Lung Disease; COPD, chronic obstructive pulmonary disease; SD, standard deviation.
A total of 14 of the 20 patients had a baseline positive response to the bronchodilator based on the FEV1 before valve placement, although none met the criteria for significant response (>12% and 200 mL). None of the patients were considered to have ACOS when assessed for a history of asthma, absolute eosinophil count, and known allergies. The mean eosinophil count was 335±229 K/µL. IgE levels were not obtained for any of the patients within the cohort. Of these 14 patients with a baseline non-significant BDR prior to valve placement, 13 demonstrated further increases in the BDR after valve placement. Of the 20 patients, 16 demonstrated a positive BDR after valve placement. Three of these 16 patients did meet criteria for a significant response. Four patients who demonstrated a negative response to BDR before valve placement developed a positive BDR following BLVR. Two patients who had a positive response to BDR prior to BLVR developed a negative response following the procedure. One patient had a negative response to BDR that improved following BLVR but remained a negative value.
Figure 1 summarizes the changes in BDR before and after BLVR. The mean absolute BDR before and after valve placement for all 20 patients was 47.5 vs. 86.5 mL, respectively, with an improvement of 39 mL [95% confidence interval (CI): 22.3 to 100.3, P=0.21]. The mean absolute BDR before and after valve placement for patients who demonstrated a positive BDR at baseline was 83.6 vs. 116.3 mL. The mean improvement in BDR for patients who demonstrated a positive BDR at baseline was 32.7 mL (95% CI: −32.5 to 97.8, P=0.31). The mean improvement in post-bronchodilator FEV1 before and after valve placement was 20.2 mL (95% CI: 6.6 to 33.8, P=0.005). Table 2 summarizes all spirometric results.
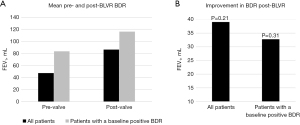
Table 2
Functional measurement | Pre-valve (95% CI) | Post-valve (95% CI) | Difference (95% CI) | P value |
---|---|---|---|---|
Prebronchodilator FEV1 (mL) | 77.85 (69.3 to 86.4) | 94.15 (85.2 to 103) | 16.3 (4.3 to 28.2) | 0.009 |
Postbronchodilator FEV1 (mL) | 82.6 (72.4 to 92.8) | 102.8 (93.1 to 112) | 20.2 (6.6 to 33.8) | 0.005 |
Bronchodilator response (mL)—all patients | 47.5 (13.0 to 82.0) | 86.5 (33.3 to 140) | 39 (22.3 to 100.3) | 0.21 |
Bronchodilator response (mL)—patients with baseline positive BDR | 83.6 (52.1 to 115.0) | 116.3 (59.0 to 173.4) | 32.7 (−32.5 to 97.8) | 0.31 |
PreBD FVC (mL) | 210 (181 to 239) | 247.5 (226 to 269) | 37.5 (2.4 to 72.6) | 0.04 |
PostBD FVC (mL) | 226.9 (189 to 264) | 272.5 (240 to 305) | 45.65 (2.2 to 93.5) | 0.06 |
FVC BDR (mL) | 169 (36.9 to 301) | 250.5 (109 to 392) | 81.5 (−37.6 to 200.6) | 0.38 |
TLC (mL) | 782.7 (705 to 860) | 707.3 (637 to 777) | −75.4 (−176.4 to 25.5) | 0.14 |
RV (mL) | 523.6 (460 to 587) | 424.1 (374 to 474) | −99.5 (−177.2 to −21.9) | 0.01 |
DLCO (% predicted, Hgb adjusted) | 33.25 (29.4 to 37.1) | 39.4 (33.8 to 45.0) | 6.15 (−0.4 to 12.7) | 0.06 |
6MWD (meters) | 254.8 (216 to 294) | 321.4 (278 to 365) | 66.6 (9.9 to 123.3) | 0.02 |
O2 requirement (L) | 1.8 (0.88 to 2.72) | 0.8 (0.08 to 1.52) | −1 (−2.1 to 0.1) | 0.08 |
CI, confidence interval; FEV1, forced expiratory volume in the first second; BDR, bronchodilator response; BD, bronchodilator; FVC, forced vital capacity; TLC, total lung capacity; RV, residual volume; DLCO, diffusion capacity of the lungs for carbon monoxide; Hgb, hemoglobin; 6MWD, six-minute walk distance.
Discussion
Our study suggests that BLVR may impact the BDR, although the results were non-significant. The mean improvement in BDR may contribute to the overall improvement in FEV1 reported by several studies (8). In addition, since some patients exhibited a negative response to bronchodilators before and after valve placement, we opted to assess the increase in BDR for all patients and those who demonstrated a positive response at baseline separately. In our cohort, a proportion of patients had a non-significant BDR before valve placement, however, this can occur in patients with COPD. To ensure that the incomplete reversibility was not compounded by a diagnosis of ACOS, a review of medical records was performed to assess for other features of asthma such as eosinophilia, allergies, IgE levels, and history of asthma. To our knowledge, none of the patients were categorized as having ACOS. In addition, there was no correlation within our study between eosinophil count and reversibility. Our study does not suggest that the BDR alone is responsible for the improvement following BLVR, but rather may be a factor in addition to those already well-known (improvement in diaphragmatic positive, ventilation-perfusion matching, etc.) in the overall improvement after complete lobar atelectasis.
Most recent studies such as the EMPROVE and REACH trials demonstrated an improvement in the post-bronchodilator FEV1 of 101 and 104 mL, respectively (9,10). However, the reported range of improvement indicates a potentially large variability which we encountered as well. When compared to these studies, our data showed only a 20.2 mL improvement in post-bronchodilator FEV1, however, this may have been due to the small sample size which is susceptible to potential outliers. In our study, the improvement encountered in the BDR, although non-significant, was greater than the improvement in post-bronchodilator FEV1 before and after valve placement. In addition, similar to investigations assessing the benefits of BLVR, our study also demonstrated an increase in the distance achieved during a 6-minute walk test and a reduction in the RV (11).
The exact mechanism for the improvement of BDR in our study is unknown, however, we postulate several theories. It is important to note that physiologic studies assessing inhaled aerosolization have demonstrated that the distribution of inhaled particles is influenced by several factors including particle size and presence of emphysema (12,13). In addition, the dispersion of aerosolized particles differs along the entire respiratory tract (14). We, therefore, propose that the endobronchial valves prevent inhaled bronchodilator particles from entering the obstructed bronchus, thus rerouting aerosolized bronchodilator medication to alternate areas of the lung. These areas with less emphysematous airway destruction offer a greater response to the inhaled bronchodilator. Therefore, the redistribution of inhaled medication to other areas of the lung may increase the concentration of drug present and receptor saturation leading to a more robust BDR. Alternatively, severe air trapping causes the respiratory muscles, particularly, the intercostal muscles and diaphragm to acquire a position at rest that is suboptimal for muscle fiber overlap to produce maximal tension. This phenomenon, coupled with increased positive pressure at rest from static hyperinflation, may hinder inspiratory airflow (15,16). The associated reduction in RV that occurs following BLVR leads to improvement in diaphragmatic positioning and therefore inspiratory capacity. This effect may lead to improvement in inspiratory flow and increased aerosolized drug delivery to all airways.
We recognize that this study has significant limitations. First, the sample size is low and did not reach the threshold for statistical significance based on the P value. In addition, the study was susceptible to selection bias since it was limited to the assessment of patients who achieved TLVR, and PFTs within a certain time frame, as well as other inclusion criteria. There was also a wide range of variation which may not represent the broader population and is subject to outliers influencing the data. Finally, PFT is subject to patient effort with each measurement and may be variable based on the patient’s learning curve, level of fatigue, timing of last dose of medication, time of day, medication inhalation technique, etc. We did our best to control some aspects of this by providing self-control group metrics and excluding patients who did not meet ATS criteria for acceptability, however, many of these effects may still be in play.
Conclusions
Our preliminary data suggest that BLVR may influence the BDR in patients who achieve complete lobar atelectasis. Although our study favored an improvement in the BDR based on the limited data, we recommend additional investigations with a larger sample population. In addition, subgroup analysis should be performed to assess the changes in BDR based on the target lobe.
Acknowledgments
Funding: None.
Footnote
Reporting Checklist: The authors have completed the STROBE reporting checklist. Available at https://jtd.amegroups.com/article/view/10.21037/jtd-24-717/rc
Data Sharing Statement: Available at https://jtd.amegroups.com/article/view/10.21037/jtd-24-717/dss
Peer Review File: Available at https://jtd.amegroups.com/article/view/10.21037/jtd-24-717/prf
Conflicts of Interest: All authors have completed the ICMJE uniform disclosure form (available at https://jtd.amegroups.com/article/view/10.21037/jtd-24-717/coif). The authors have no conflicts of interest to declare.
Ethical Statement: The authors are accountable for all aspects of the work in ensuring that questions related to the accuracy or integrity of any part of the work are appropriately investigated and resolved. The study was conducted in accordance with the Declaration of Helsinki (as revised in 2013). The study was approved by the ethics board of Saint Luke’s University Health Network (FWA 00003557. No. SLIR 2023-28) and individual consent for this retrospective analysis was waived.
Open Access Statement: This is an Open Access article distributed in accordance with the Creative Commons Attribution-NonCommercial-NoDerivs 4.0 International License (CC BY-NC-ND 4.0), which permits the non-commercial replication and distribution of the article with the strict proviso that no changes or edits are made and the original work is properly cited (including links to both the formal publication through the relevant DOI and the license). See: https://creativecommons.org/licenses/by-nc-nd/4.0/.
References
- Agustí A, Celli BR, Criner GJ, et al. Global Initiative for Chronic Obstructive Lung Disease 2023 Report: GOLD Executive Summary. Eur Respir J 2023;61:2300239. [Crossref] [PubMed]
- Fessler HE, Scharf SM, Ingenito EP, et al. Physiologic basis for improved pulmonary function after lung volume reduction. Proc Am Thorac Soc 2008;5:416-20. [Crossref] [PubMed]
- Patel M, Chowdhury J, Zhao H, et al. Meta-analysis and Systematic Review of Bronchoscopic Lung Volume Reduction Through Endobronchial Valves in Severe Emphysema. J Bronchology Interv Pulmonol 2022;29:224-37. Erratum in: J Bronchology Interv Pulmonol 2022;29:311. [Crossref] [PubMed]
- van Agteren JE, Hnin K, Grosser D, et al. Bronchoscopic lung volume reduction procedures for chronic obstructive pulmonary disease. Cochrane Database Syst Rev 2017;2:CD012158. [Crossref] [PubMed]
- Graham BL, Steenbruggen I, Miller MR, et al. Standardization of Spirometry 2019 Update. An Official American Thoracic Society and European Respiratory Society Technical Statement. Am J Respir Crit Care Med 2019;200:e70-88. [Crossref] [PubMed]
- Carstairs JR, Nimmo AJ, Barnes PJ. Autoradiographic visualization of beta-adrenoceptor subtypes in human lung. Am Rev Respir Dis 1985;132:541-7. [PubMed]
- Labiris NR, Dolovich MB. Pulmonary drug delivery. Part I: physiological factors affecting therapeutic effectiveness of aerosolized medications. Br J Clin Pharmacol 2003;56:588-99. [Crossref] [PubMed]
- Abia-Trujillo D, Johnson MM, Patel NM, et al. Bronchoscopic Lung Volume Reduction: A New Hope for Patients With Severe Emphysema and Air Trapping. Mayo Clin Proc 2021;96:464-72. [Crossref] [PubMed]
- Criner GJ, Delage A, Voelker K, et al. Improving Lung Function in Severe Heterogenous Emphysema with the Spiration Valve System (EMPROVE). A Multicenter, Open-Label Randomized Controlled Clinical Trial. Am J Respir Crit Care Med 2019;200:1354-62. [Crossref] [PubMed]
- Li S, Wang G, Wang C, et al. The REACH Trial: A Randomized Controlled Trial Assessing the Safety and Effectiveness of the Spiration® Valve System in the Treatment of Severe Emphysema. Respiration 2019;97:416-27. [Crossref] [PubMed]
- Alshabani K, Gildea TR, Machuzak M, et al. Bronchoscopic lung volume reduction with valves: What should the internist know? Cleve Clin J Med 2020;87:278-87. [Crossref] [PubMed]
- Kirby M, Svenningsen S, Kanhere N, et al. Pulmonary ventilation visualized using hyperpolarized helium-3 and xenon-129 magnetic resonance imaging: differences in COPD and relationship to emphysema. J Appl Physiol (1985) 2013;114:707-15. [Crossref] [PubMed]
- Oakes JM, Marsden AL, Grandmont C, et al. Distribution of aerosolized particles in healthy and emphysematous rat lungs: comparison between experimental and numerical studies. J Biomech 2015;48:1147-57. [Crossref] [PubMed]
- Cheng YS. Mechanisms of pharmaceutical aerosol deposition in the respiratory tract. AAPS PharmSciTech 2014;15:630-40. [Crossref] [PubMed]
- Chen Y, Li J, Zhu Z, et al. Lung Ultrasound Assessment of Lung Hyperinflation in Patients with Stable COPD: An Effective Diagnostic Tool. Int J Chron Obstruct Pulmon Dis 2024;19:319-30. [Crossref] [PubMed]
- Gagnon P, Guenette JA, Langer D, et al. Pathogenesis of hyperinflation in chronic obstructive pulmonary disease. Int J Chron Obstruct Pulmon Dis 2014;9:187-201. [PubMed]