Epidemiological characteristics of severe community-acquired pneumonia in children admitted to two tertiary hospitals in Shihezi, Xinjiang Region, China in 2023: a cross-sectional analysis
Highlight box
Key findings
• Mycoplasma pneumoniae (MP) was the primary cause of severe community-acquired pneumonia (SCAP) in children in 2023 in Shihezi, Xinjiang.
• The prevalence of macrolide resistance was high (63.3%), always related to A2063G mutations.
• MP was associated with longer duration of symptoms.
• Macrolide resistance did not seem to impact outcome.
What is known and what is new?
• Metagenomic next-generation sequencing is an emerging molecular biology technology. The combination of multiplex quantitative polymerase chain reaction and next-generation sequencing technology allows to decipher the pathogen spectrum, and antimicrobial resistance genes.
• This study on SCAP in children in Shihezi, Xinjiang, China provides a basis for formulating prevention and control strategies for respiratory infectious diseases in children, and to adjust empirical treatment.
What is the implication, and what should change now?
• When SCAP is diagnosed, initial empirical treatment should be based on local epidemiology. In 2023, children with SCAP should ideally receive doxycycline, as MP was the primary pathogen, with high prevalence of macrolides resistance.
Introduction
Severe community-acquired pneumonia (SCAP) in children is associated with high morbidity and mortality. It may be caused by various pathogens including bacteria, viruses and more rarely fungi, and the epidemiology may differ according to geographical areas, seasons, and epidemics (1,2). According to statistics, 156 million people experience community-acquired pneumonia (CAP) worldwide each year, with 7–13% potentially developing into severe cases (3). Due to their underdeveloped respiratory systems and immunity, children are at higher risk of SCAP (4), with dramatic differences from one setting to another (5,6). Among the various diagnostic methods for SCAP, common pathogen detection techniques such as direct examination and cultures of respiratory samples, antigenic tests, and serological assays have significant caveats, including long turn-around time with delayed results, sub-optimal sensitivity, and inability to identify important pathogens (7). Molecular biology diagnostic techniques may improve the detection rate and clarify the pathogen spectrum of pediatric SCAP. Numerous studies have reported that multiplex quantitative polymerase chain reaction (qPCR) and metagenomic sequencing-based diagnostic techniques can provide reliable tools for early diagnosis and appropriate treatment of lower respiratory tract infections (8-10). The metagenomic next-generation sequencing (mNGS) based on the bioinformatics method may allow rapid identification of the pathogen spectrum of SCAP, even for unsuspected pathogens, providing precise guidance for clinical diagnosis and treatment (11-13). Early identification of the pathogens responsible for SCAP in children can help reduce mortality, and improve treatment outcomes and prognosis.
The dominant respiratory tract pathogens of SCAP vary in different regions. In Guangzhou, China, pathogens such as Mycoplasma pneumoniae (MP) and respiratory syncytial virus (RSV) predominate, while in Fujian, China, pathogens such as Acinetobacter baumannii and Pseudomonas aeruginosa are dominant (14,15). A study in Vietnam indicates that Streptococcus pneumoniae (SP) and rhinovirus (RhV) hold a dominant position among the pathogens in that region (10). RhV and RSV are the predominant pathogens of SCAP in Nepal (16). Previous study has shown that children with SCAP are more prone to mixed infections than those with CAP (17). Shihezi Region is the central city of the 8th Division of the Xinjiang Production and Construction Corps, where the number of child cases is higher than that in surrounding areas. Among various types of diseases, pneumonia is a common disease. A review of relevant literature did not find any epidemiological report on pediatric SCAP in Shihezi, Xinjiang, China. This study analyzes and summarizes the epidemiological characteristics of pediatric SCAP in the Shihezi region from January to December 2023. The objective of this study is to characterize the pathogens responsible for pediatric SCAP in this area, to better inform infection control and prevention, and to adjust empirical treatment to the main pathogens potentially involved. We present this article in accordance with the STROBE reporting checklist (available at https://jtd.amegroups.com/article/view/10.21037/jtd-24-1417/rc).
Methods
Study population and data collection
This study included 309 pediatric patients aged 1 month to 14 years diagnosed with SCAP from 1 January 2023 to 31 December 2023, at the First Affiliated Hospital of Shihezi University and Shihezi People’s Hospital. Both hospitals are part of a comprehensive tertiary medical center with a significant volume of pediatric inpatients, and the medical staff possess strong capabilities in scientific research and have access to substantial funding for their research endeavors, and they are informed and agreed with this study. Nevertheless, the limited enrollment of hospitals, may have resulted in regional differences. The Ministry of Health of China stipulates that the age of pediatric clinical service objects is from birth to 14 years old. The age range of hospitalized children in Shihezi hospitals is below 14 years old. Therefore, children between 15 and 17 years old are not included in this study. The study was conducted in accordance with the Declaration of Helsinki (as revised in 2013). The study was approved by ethics board of the First Affiliated Hospital of Shihezi University (No. KJ2022-216-01). Shihezi People’s Hospital was informed and agreed with this study. Written informed consent was obtained from their parental/legal guardians.
SCAP patients were included according to the clinical guidelines (18,19) and satisfy one of the following conditions: presence of disturbance of consciousness, shortness of breath, dyspnea, multiple lung lobes involvement, and oxygen saturation of anaerobic pulse blood <92%, pleural effusion or extrapulmonary complications. The exclusion criteria of SCAP are as follows: first, respiratory system-related diseases, such as: bronchial asthma, bronchopulmonary dysplasia, foreign body inhalation, tuberculosis infection, etc.; second, recent repeated hospital admissions, hospital acquired pneumonia cannot be ruled out; third, deficiency of immune function, long-term use of glucocorticoids or immunosuppressants; fourth, giving up or refusing treatment without reason or incomplete clinical data. The patients were divided into four age groups: infants (1 month to 1 year), toddlers (>1–3 years), preschool children (>3–7 years), and school-age children (>7–14 years). They were also categorized by season: spring (March, April, May), summer (June, July, August), autumn (September, October, November), and winter (December, January, February).
MP was the dominant pathogen detected during the study period. Based on whether they had a mycoplasma infection, children were grouped into three MP-infection categories (0 = no MP infection, 1 = MP single infection, 2 = MP co-infection with other pathogen). According to whether multiple pathogens were detected by 27 respiratory pathogens using a panel, targeted next-generation sequencing (t-NGS), and mNGS, they were divided into six mixed infection groups (0 = no pathogens detected, 1 = single pathogen detected, 2 = two pathogens detected, 3 = three pathogens detected, 4 = four pathogens detected, 5 = five pathogens detected, 6 = more than 5 pathogens detected) (Figure 1). They were also categorized by macrolide resistance into non-resistant MP and macrolide-resistant MP (MRMP).
According to the Technical Regulation on Ambient Air Quality Index (AQI) (Trial) (HJ633-2012) (20), air quality was classified as: first-level air quality: excellent, PM2.5 ≤50 µg/m3; second-level air quality: good, PM2.5 from 51 to <100 µg/m3. The data of air quality was collected the website of the Department of Ecology and Environment of Xinjiang Uygur Autonomous Region. Information was collected retrospectively through a standardized questionnaire by physicians, covering basic information, epidemiological data, length of hospital stay, fever peak, and PM2.5 concentration in the air. Respiratory specimens were collected within 24 hours of admission, consisting of at least one of the following: throat swab or bronchoalveolar lavage fluid (BALF).
Laboratory testing
Nasopharyngeal swab samples were subjected to nucleic acid testing for 27 respiratory pathogens: 18 viruses, 9 bacteria, including Chlamydophila pneumoniae (CP), and MP (Table S1), or targeted metagenomic sequencing for 107 respiratory pathogens (Table S2). For patients with no respiratory pathogen identified or whose condition required bronchoscopy, 5 mL of BALF was collected via bronchoscopy and sent for t-NGS of 198 respiratory pathogens (Table S3), mNGS, and targeted gene sequencing to detect resistance genes of common pathogens, including MP. However, bronchoscopy was not performed in children with good response to treatment after admission and no pathogen was detected.
Statistical analysis
Statistical analysis was conducted using SPSS 26.0 software (IBM Corp., Armonk, NY, USA). Continuous variables were presented as mean [95% confidence interval (CI)] and compared between two groups using t-tests. Categorical data were described using frequency (percentage). Analysis of variance (ANOVA) was used to compare the course of disease and highest body temperature in different seasons, age and MP-infection groups. Non-parametric tests were used when continuous variables were skewed distributed. Spearman correlation coefficients were used to analyze the correlation between the number of pathogens and the length of hospital stay and fever peak, as well as the correlation between PM2.5 levels and pathogens. Multiple-stage logistic regression was performed to analysis interaction among multiple common respiratory pathogens. A two-sided P value <0.05 was considered statistically significant. Graphs were generated using GraphPad Prism software (GraphPad Software, San Diego, CA, USA).
Results
Study population and overall distribution of pathogens
We enrolled 309 consecutive cases of pediatric SCAP cases admitted during the study period: 157 males (50.8%) and 152 females (49.2%). There were 45 infants (1 month to 1 year, 14.6%), 33 toddlers (>1–3 years, 10.7%), 121 preschool children (>3–7 years, 39.2%), and 110 school-age children (>7–14 years, 35.6%) (Table 1). In this study, the number of SCAP cases in older children was significantly higher than that in younger children. The number of SCAP cases in spring was 39 (12.6%), in summer was 44 (14.2%), in autumn was 137 (44.3%), and in winter was 89 (28.8%). A total of 572 pathogens were detected in this study, primarily MP (n=120, 21.0%), RSV (n=82 strains, 14.3%), and SP (n=67, 11.7%). MP was prevalent mainly during the cold season of autumn, with its detection starting from July and reaching a peak detection rate in November.
Table 1
Variables | Values (N=309) |
---|---|
The demographic and clinical characteristics | |
Sex, n (%) | |
Male | 157 (50.8) |
Female | 152 (49.2) |
Age, n (%) | |
Infant (1 month to 1 year) | 45 (14.6) |
Toddler (>1–3 years) | 33 (10.7) |
Preschool child (>3–7 years) | 121 (39.2) |
School child (>7–14 years) | 110 (35.6) |
Season, n (%) | |
Spring (Mar to May) | 39 (12.6) |
Summer (Jun to Aug) | 44 (14.2) |
Autumn (Sept to Nov) | 137 (44.3) |
Winter (Dec to Feb) | 89 (28.8) |
The positive detection of pathogens | |
Sex, n (%) | |
Male | 152 (96.8) |
Female | 142 (93.4) |
Age, n (%) | |
Infant (1 month to 1 year) | 45 (100.0) |
Toddler (>1–3 years) | 32 (97.0) |
Preschool child (>3–7 years) | 114 (94.2) |
School child (>7–14 years) | 103 (93.6) |
Season, n (%) | |
Spring (Mar to May) | 36 (92.3) |
Summer (Jun to Aug) | 43 (97.7) |
Autumn (Sept to Nov) | 130 (94.9) |
Winter (Dec to Feb) | 85 (95.5) |
Summarizing the results of respiratory pathogen detection through qPCR testing (n=27), t-NGS (n=107), t-NGS combined with mNGS (n=198), a total of 47 respiratory pathogens were detected at least once in this study (total number of respiratory pathogens detections, n=572): the main respiratory pathogens detected were bacteria (n=337, 58.9%), including 120 MP (21.2%), 67 SP (11.7%) and 64 Haemophilus influenzae cases (11.2%). The second pathogens were viruses (n=232, 40.6%), including RSV (n=82, 14.3%). Only 3 fungal respiratory pathogens were detected (Figure 2A).
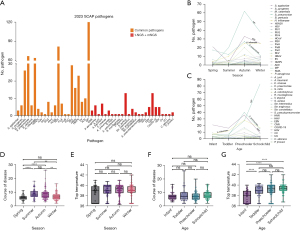
The study identified a seasonal pattern in pathogen detection. Among the pathogens detected at high frequencies, RSV had the highest detection rate in the spring, MP began appearing in the summer, peaked in the autumn, and decreased in the winter, and MP, H. influenzae, and RSV were the primary pathogens present in winter (Figure 2B). Regarding age-specific pathogen characteristics, RSV was the most commonly detected pathogen causing SCAP in infants; toddlers were mostly infected with RSV and H. influenzae; SP had the highest detection rate in preschool children; and MP had the highest detection rate in school-age children, with its infection rate increasing with age (Figure 2C).
Hospitalization duration and fever peaks in children with SCAP by different season and age groups
The average hospital stay duration for children with SCAP in spring, summer, autumn, and winter were 6.15 (95% CI: 5.48–6.83), 8.73 (95% CI: 7.73–9.72), 8.37 (95% CI: 7.79–8.96), and 7.00 (95% CI: 6.39–7.61) days, respectively. Hospital stays were longer in summer and autumn than in spring and winter (Figure 2D). The average fever peaks for spring, summer, autumn, and winter were 38.53 (95% CI: 38.06–39.01), 38.90 (95% CI: 38.55–39.25), 39.04 (95% CI: 38.86–39.21), and 39.04 (95% CI: 38.79–39.30) ℃, respectively, with no statistically significant differences in fever peaks among the four seasons (Figure 2E). The average hospital stays durations for infants, toddlers, preschool children, and school-age children were 7.29 (95% CI: 6.42–8.15), 8.24 (95% CI: 6.52–9.96), 7.35 (95% CI: 6.82–7.88), and 8.23 (95% CI: 7.65–8.81) days, respectively, with no statistically significant differences among the four age groups (Figure 2F). The average fever peaks for infants, toddlers, preschool children, and school-age children were 37.82 (95% CI: 37.46–38.18), 38.96 (95% CI: 38.60–39.31), 39.07 (95% CI: 38.85–39.30), and 39.29 (95% CI: 39.13–39.45) ℃, respectively. The maximum body temperature during the course of illness in infants was significantly lower than in the other three age groups (P<0.0001) (Figure 2G).
Multiple pathogens co-detection
We conducted a statistical analysis on the co-detection of respiratory pathogens in 309 children with SCAP. Among them, 15 cases (4.9%) had no pathogen detected, 145 (46.9%) had one pathogen detected, and 149 (48.2%) had multiple pathogens detected. Among SCAP with multiple respiratory pathogen detections, two pathogens were co-detected in 74 cases (50.0%), three in 45 cases (30.2%), four in 16 cases (10.7%), five in 11 cases (7.4%), and more than five in 3 cases (2.0%) (Figure 3A). As the number of co-detected pathogens increased, the hospitalization duration for children with SCAP increased. There was a weak positive correlation between number of co-detected pathogens and hospital stay duration (r=0.135, P<0.05) (Figure 3B).
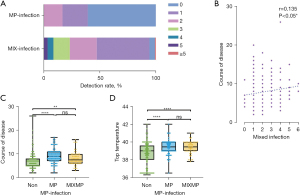
A total of 120 children (38.8%) had MP detected, including 69 (22.3%) with no co-infection, and 51 (16.5%) having MP co-detected with other pathogens (Figure 3A). The hospital stay duration of children with MP and co-infection was longer than that of children without MP detected (P<0.01), and the hospital stay duration of children with MP as a single pathogen was longer than that of children with no MP detected (P<0.0001) (Figure 3C). Similar findings were observed fever peak in children with MP as the single pathogen was higher than those with no MP infection (P<0.0001) (Figure 3D).
Interactions among multiple co-detected pathogens
We analyzed the interaction of two respiratory pathogens among the most common co-infections: co-detection of RSV and S. pneumoniae (n=21), RSV and H. influenzae (n=21), S. pneumoniae and MP (n=19), and H. influenzae and MP (n=16). Given that MP had the highest detection rate, we analyzed the co-detection of pathogens related to MP and found that most pathogens had a negative correlation with MP co-detection, the strongest negative correlation being between MP and S. pneumoniae (Figure 4A).
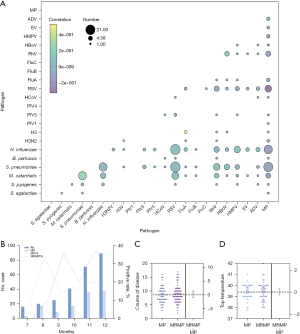
Mycoplasma and related resistance genes
Temporal analysis of the 120 cases of MP SCAP diagnosed (34.0%), found that the epidemic started in July and peaked in November with 36 cases detected and a detection rate of 50.7% that month. We found genes associated with macrolide resistance in 76 cases (63.3%) (Figure 4B), with no statistically significant differences in hospital stay duration and fever peaks between children with SCAP due to MP with, or without resistance genes to macrolides (Figure 4C,4D).
Correlation between PM2.5 levels and pathogen infections
In this study, we included the monitoring of PM2.5 levels in the environment. The PM2.5 levels in the ambient air in Shihezi were highest in the spring and lowest in the autumn. However, the number of SCAP in children were lowest in the spring and highest in the autumn, showing an inverse relationship between PM2.5 concentrations and the number of SCAP cases (Figure 5A). When PM2.5 levels were at a satisfactory level, the main pathogens were MP, RSV, and H. influenzae. When PM2.5 levels were at a good level, the main pathogens were MP, RSV, and S. pneumoniae (Figure 5B). Higher PM2.5 levels were associated with an increased likelihood of MP, with or without co-infections (r=0.149, P<0.01) (Figure 5C).
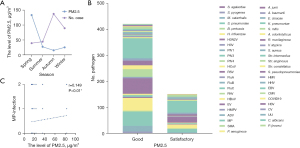
Discussion
The pathogen spectrum of SCAP varies in different regions, and identifying the pathogen spectrum can help in early intervention to prevent disease progression and reduce SCAP incidence.
The main findings of this prospective study in the Shihezi region, with extensive testing for pathogens on respiratory samples, including t-NGS and MNGS, are the followings: (I) the most common pathogens responsible for SCAP in children in 2023 were MP, RSV, and S. pneumoniae; (II) MP was the primary cause of SCAP in children, especially school-age, with a seasonal peak in autumn, increased risk of co-infections, and high prevalence of macrolides resistance—all related to mutations at the A2063G site; (III) co-detection of more than one potential respiratory pathogens was common, in 48% of children with SCAP during the study period.
The pathogen spectrum of SCAP varies in different regions, and may vary over time. To better characterize the spectrum of respiratory pathogens responsible for SCAP can guide infection prevention and control measures, and improve the selection of empirical antimicrobial regimens, more likely to be active if the main pathogens to be targeted are identified. Huang et al. reported that MP, RSV, and S. pneumoniae were the main pathogens causing SCAP in children in Guangzhou City (14). Tong et al. reported that the main pathogens causing severe pneumonia in children in a certain area of the Fujian Province were Acinetobacter baumannii, Pseudomonas aeruginosa, Klebsiella pneumoniae, and Staphylococcus aureus (15). Li et al. reported that the main pathogens causing SCAP in children in a certain area of Guangzhou City were RSV, RhV, and P. aeruginosa (21). In this study, the main pathogens in Shihezi region were MP, RSV, and S. pneumoniae, in line with previous findings by Mathisen et al. (16).
The clinical characteristics of SCAP due to MRMP strains need further investigation. A previous study has shown that MP are responsible for explosive outbreaks every 3–7 years (22). The last MP epidemic in China before our study occurred in 2019, and was followed by enhanced preventive measures. The usual pace of MP epidemics with free intervals during 3–7 years between each epidemic, may be related to the herd immunity, primarily in children and young adults—the main population affected by MP epidemics. Our study findings have been dramatically affected by the occurrence of a large MP outbreak, worldwide, in 2023.
Different pathogens were prevalent in different age groups. This study found that RSV was the most commonly detected pathogen causing SCAP in infants. Toddlers were mostly infected with RSV and H. influenzae, preschool children had the highest detection rate of S. pneumoniae, and school-age children had the highest detection rate of MP, with MP infection rates increasing with age. Pathogen prevalence also varies by season. We observed a seasonal pattern in pathogen detection: besides winter, RSV detection rates were generally higher in spring and autumn. Mathisen et al. reported that RSV is most prevalent in winter, which differs from our findings (16). Our study found that MP appeared in the summer, peaked in the autumn, and decreased in the winter. Wang et al. reported that autumn is the peak season for MP prevalence, which is consistent with these results (23). Therefore, SCAP prevention should be based on the epidemiological characteristics of pathogens in children of different ages and seasons in this region, and vaccinations should be administered accordingly.
This study found that there were fewer SCAP cases in the summer, but the hospitalization duration in the summer was significantly longer than in other seasons. This could be related to the following reasons: first, limited medical resources: the number of SCAP cases in autumn and winter was significantly higher than in summer, resulting in faster bed turnover and shorter hospital stays. Second, due to limited bed availability, some children could not be hospitalized early and had already received routine antibiotic treatment outside the hospital, leading to hospitalization during the recovery phase of pneumonia and thus shorter hospital stays. Third, in this study, after MRMP infection was confirmed by t-NGS, and Chinese clinical guidelines recommend the use of doxycycline in children with drug-resistant mycoplasma infection (15), many children were switched to oral doxycycline to overcome macrolides resistance, which may have decreased the impact of macrolide resistance on hospital stays duration.
In a previous study, molecular diagnostic tests for identification of SCAP pathogens mostly used qPCR. Mathisen et al. re-analyzed 610/627 well-preserved respiratory samples from children with SCAP using qPCR technology that may detect 22 different pathogens: They could identify at least one respiratory pathogen in 30% of cases (16). The detection rate for single or mixed infections in our study was 98.7%, which suggests that the detection rate of a single method is lower than that of combined methods. In this study, in addition to qPCR screening for 27 respiratory pathogens and t-NGS screening for 107 respiratory pathogens, the combined use of t-NGS and mNGS, screening for 198 different pathogens, could detect a total of 47 respiratory pathogens (Figure 2A). In addition to 22 common respiratory pathogens, 25 rare pathogens were also detected (16 bacteria, 7 viruses and 2 fungi). Among all children with SCAP investigated in our study, only 2 had no respiratory pathogen detected with the qPCR, but further mNGS allowed detection of additional pathogens, often as co-infection, demonstrating the potential added value of NGS technology, even when one pathogen has been identified. Sequencing results of respiratory samples from these 2 cases, when compared with the CARD database, confirmed the presence of the NDM-6 resistance gene, primarily originating from Acinetobacter baumannii, Klebsiella pneumoniae, and Enterobacter (24-26). Gong et al. reported that carbapenem-resistant Klebsiella pneumoniae carrying the NDM-6 gene is resistant to almost all β-lactam antibiotics except monobactams (26).
Huang et al. reported that 50.0% of SCAP children had multiple pathogens detected simultaneously, with 75% of these patients having two pathogens detected and 25% having more than two pathogens detected (27). Mathisen et al. observed that 53.3% of SCAP children had multiple pathogens detected, with 15.4% of patients having more than two pathogens detected (16). In this study, 48.2% of SCAP children had multiple pathogens co-detected, and 24.4% had more than two pathogens detected. The rate of multiple pathogen co-detection in this study was higher than that in previous studies. Compared to other epidemiological studies, we found that most previous research used single detection methods. This study employed qPCR combined with high-throughput sequencing platforms, which significantly increased the positive detection rate of SCAP pathogens and supplemented the pathogen spectrum. This study confirms that NGS technology may improve the diagnosis and treatment levels of pediatric SCAP.
Among the co-infections involving the 22 most common pathogens, S. pneumoniae was the main pathogen detected. This suggests that, at least in SCAP cases, if S. pneumoniae infection is documented, it may still be valuable to screen for co-infections, especially with RSV, MP and H. influenzae, to ensure that all pathogens potentially involved are identified, and treated, for this life-threatening disease. Except for MP, the co-detection patterns between the other 21 pathogens mostly showed positive correlations, with the strongest positive correlation between two influenza A isolates. Wang et al. also found that MP had a negative correlation with most other pathogens (i.e., co-infection rarely involves MP), except for co-infection with S. pneumoniae (23), which is consistent with our findings.
MP may be responsible for up to 40% of all cases of CAP during epidemics (28). This may also apply to SCAP, as MP was the primary pathogen responsible for SCAP in Shihezi in 2023. This must be outlined, as MP has long been considered as a rather benign CAP, often self-resolving. However, Fan et al. found that children with MP CAP had higher fever peaks and longer hospital stays durations than other causes of CAP in this population (29), which is consistent with the results of our study.
Many empirical treatments recommended for CAP target the bacteria cell wall. Hence, they have no activity on MP, an intra-cellular bacterium with no cell wall. Macrolides are the first-choice empirical treatment for MP, particularly in children younger than 8 years, for whom the main alternative, doxycycline, has long been contra-indicated. The misuse of antibiotics in Asia has led to a high prevalence of MRMP (30-32). The complexity of diagnosing macrolides resistance in MP, a non-culturable organism in mots microbiology lab, delays the diagnosis and appropriate treatment of MRMP, which may increase the risk of SCAP (33). Therefore, early identification of MRMP is crucial for optimal treatment of CAP. The currently known MRMP sites include the A2063G, A2064G/C, A2067G, and A2017G mutations in the V domain of the 23SrRNA gene, as well as mutations in ribosomal proteins L4 and L22 (34). Among these, mutations at the A2063G and A2064G/C sites are the primary causes of high-level resistance to macrolides (34). Besides the known resistance sites, Faucher et al. discovered that chromosomal transfer in MP leads to gene recombination, resulting in unpredictable resistance (35). The MRMP prevalence in a certain area of Beijing has recently increased, reaching 93.9% of all MP in 2020 (36). In our study, the MRMP prevalence among SCAP children in Shihezi region was slightly (i.e., 63%), but still of high concern. The different MRMP prevalence across regions might be due to real differences, or sub-optimal estimates due to insufficient case numbers, highlighting the need to increase the number of sentinel hospitals or extend the study period to expand the sample size. However, Xu et al. found no significant correlation between macrolides resistance and either fever peaks or hospital stay duration (37), as in our study.
PM2.5 levels in ambient air have been correlated with reduced lung function and the incidence of respiratory diseases (38). PM2.5 can induce lung injury and inflammatory responses by upregulating TLR2 and TLR4 on alveolar macrophages through the transfer of Th1/Th2 cells in the inflammatory response (39,40). In Shihezi, we found an inverse relationship between PM2.5 levels and SCAP incidence in children (Figure 5A). The detection rates of pathogens such as MP, RSV, H. influenzae, and S. pneumoniae showed a negative correlation with PM2.5 levels (Figure 5B). This finding might be related to reduced outdoor activities during the cold season in Xinjiang, increased social awareness of protective measures, and a higher rate of mask-wearing. Additionally, our study may have been underpowered in this regard, due to limited sample size.
This study has certain limitations. First, the samples are limited to two sentinel hospitals, which may have resulted in regional differences. Second, the limited bed availability and the use of empirical antibiotics outside the hospital for some children could have affected the detection rate of pathogens. Additionally, the relatively limited number of cases collected may have impacted the study results to some extent. So, we are going to increase the number of sentinel hospitals and lengthen the study period to help identify more comprehensive epidemiological trends.
This study has limitations. First, the samples are limited to two sentinel hospitals in Shihezi, in only one year. Hence our findings may not apply to other settings (i.e., other geographical area, or other years). This is especially relevant given the important MP epidemic that occurred during our study period. Second, the limited bed availability and the large use of empirical antibiotics as outpatients may have decreased the number of SCAP admitted in the hospital, and introduced biases (e.g., cases resistant to empirical treatment may be over-represented). Finally, the detection of potential respiratory pathogens in respiratory samples does not necessarily mean that these pathogens are, indeed, responsible for SCAP. This must be kept in mind given the high prevalence of multiple pathogens detection: Indeed, although we considered these cases as co-infections, we cannot rule out that only one pathogen was responsible for SCAP, while the other was part of the respiratory microbiota.
In this study, the combination of multiplex qPCR and NGS technology has supplemented and improved the pathogen spectrum of SCAP in this region. This provides a basis for formulating prevention and control strategies for respiratory infectious diseases related to pediatric SCAP and helps guide precise etiological treatment of SCAP in children. When SCAP is diagnosed, initial empirical treatment should be based on the regional pathogen spectrum. At the same time, early identification of the infecting pathogen is essential, and treatment plans should be adjusted according to the pathogen detection results. Ye et al. observed that patients with SCAP spent more than CAP, and their disease burden of SCAP is more significant than that of CAP, which posing a severe threat to the physical and mental well-being of children (13). Early identification of epidemiological characteristics of SCAP pathogens can help to early diagnosis and reduce the occurrence of severe cases.
Conclusions
To sum up, the most common pathogens causing SCAP in the Shihezi region were MP, RSV, and SP, with significant seasonal and age-related variations. The high prevalence of MRMP highlights the need for targeted antibiotic strategies. The study emphasizes the importance of early pathogen identification and appropriate empirical treatment based on regional pathogen spectra to improve clinical outcomes in pediatric SCAP cases. Further research with larger and more diverse populations is recommended to validate these findings and explore additional factors influencing SCAP epidemiology
Acknowledgments
Funding: This work was supported by
Footnote
Reporting Checklist: The authors have completed the STROBE reporting checklist. Available at https://jtd.amegroups.com/article/view/10.21037/jtd-24-1417/rc
Data Sharing Statement: Available at https://jtd.amegroups.com/article/view/10.21037/jtd-24-1417/dss
Peer Review File: Available at https://jtd.amegroups.com/article/view/10.21037/jtd-24-1417/prf
Conflicts of Interest: All authors have completed the ICMJE uniform disclosure form (available at https://jtd.amegroups.com/article/view/10.21037/jtd-24-1417/coif). Z.L. is from Key Laboratory of Digital Technology in Medical Diagnostics of Zhejiang Province, Dian Diagnostics Group Co., Ltd. The other authors have no conflicts of interest to declare.
Ethical Statement: The authors are accountable for all aspects of the work in ensuring that questions related to the accuracy or integrity of any part of the work are appropriately investigated and resolved. The study was conducted in accordance with the Declaration of Helsinki (as revised in 2013). The study was approved by ethics board of the First Affiliated Hospital of Shihezi University (No. KJ2022-216-01). Shihezi People’s Hospital was informed and agreed with this study. Written informed consent was obtained from their parental/legal guardians.
Open Access Statement: This is an Open Access article distributed in accordance with the Creative Commons Attribution-NonCommercial-NoDerivs 4.0 International License (CC BY-NC-ND 4.0), which permits the non-commercial replication and distribution of the article with the strict proviso that no changes or edits are made and the original work is properly cited (including links to both the formal publication through the relevant DOI and the license). See: https://creativecommons.org/licenses/by-nc-nd/4.0/.
References
- Pneumonia Etiology Research for Child Health (PERCH) Study Group. Causes of severe pneumonia requiring hospital admission in children without HIV infection from Africa and Asia: the PERCH multi-country case-control study. Lancet 2019;394:757-79. [Crossref] [PubMed]
- Fan CN, Mao YY, Liu J, et al. Dynamics of platelet parameters in children with severe community-acquired pneumonia between viral and bacterial infections. Transl pediatr 2024;13:52-62. [Crossref] [PubMed]
- Ao XX. The epidemiology of hospital death following pediatric severe community acquired pneumonia. Ital J Pediatr 2021;47:25. [Crossref] [PubMed]
- Chen Q, Lin L, Zhang N, et al. Adenovirus and Mycoplasma pneumoniae co-infection as a risk factor for severe community-acquired pneumonia in children. Front Pediatr 2024;12:1337786. [Crossref] [PubMed]
- Oumei H, Xuefeng W, Jianping L, et al. Etiology of community-acquired pneumonia in 1500 hospitalized children. J Med Virol 2018;90:421-8. [Crossref] [PubMed]
- Zar HJ, Polack FP. Childhood pneumonia: the role of viruses. Thorax 2015;70:811-2. [Crossref] [PubMed]
- Qian YY, Wang HY, Zhou Y, et al. Improving Pulmonary Infection Diagnosis with Metagenomic Next Generation Sequencing. Front Cell Infect Microbiol 2020;10:567615. [Crossref] [PubMed]
- Silva-Caso W, Pérez-Lazo G, Aguilar-Luis MA, et al. Identification and Clinical Characteristics of Community-Acquired Acinetobacter baumannii in Patients Hospitalized for Moderate or Severe COVID-19 in Peru. Antibiotics (Basel) 2024;13:266. [Crossref] [PubMed]
- Yen TY, Chen JF, Lu CY, et al. Application of nested multiplex polymerase chain reaction respiratory and pneumonia panels in children with severe community-acquired pneumonia. J Med Virol 2023;95:e28334. [Crossref] [PubMed]
- Tran Quang K, Tran Do H, Pham Hung V, et al. Study on the co-infection of children with severe community-acquired pneumonia. Pediatr Int 2022;64:e14853. [Crossref] [PubMed]
- Yu C, Guo W, Zhang Z, et al. The Impact of mNGS Technology in the Etiological Diagnosis of Severe Pneumonia in Children During the Epidemic of COVID-19. Infect Drug Resist 2023;16:2395-402. [Crossref] [PubMed]
- Zuo YS, Shen WY, Chen GM, et al. Prediction of target genes in community-acquired pneumonia based on the bioinformatics method. J Thorac Dis 2023;15:2694-2707. [Crossref] [PubMed]
- Ye Y, Su L, Gui Y, et al. Prediction of target genes in community-acquired pneumonia based on the bioinformatics method: a cross-sectional analysis. Transl Pediatr 2023;12:308-319. [Crossref] [PubMed]
- Huang L, Zheng YJ, Yang WG, et al. Pathogen Analysis of 774 Cases of Severe Pneumonia in Children in Shenzhen Area. Laboratory Medicine and Clinic 2020;17:2232-5.
- Tong CS. Analysis of Pathogen Distribution and Prognostic Risk Factors in Severe Pediatric Pneumonia. Chinese Remedies & Clinics 2021;21:683-4.
- Mathisen M, Basnet S, Christensen A, et al. Viral and Atypical Bacterial Detection in Young Nepalese Children Hospitalized with Severe Pneumonia. Microbiol Spectr 2021;9:e0055121. [Crossref] [PubMed]
- Liu YN, Zhang YF, Xu Q, et al. Infection and co-infection patterns of community-acquired pneumonia in patients of different ages in China from 2009 to 2020: a national surveillance study. Lancet Microbe 2023;4:e330-9. [Crossref] [PubMed]
- Respiratory Group of the Pediatric Division of the Chinese Medical Association. The Guidelines for the Management of Community-Acquired Pneumonia in Children (2013 Revision) (Part 1). Chinese Journal of Pediatrics 2013;51:745-52.
- "Guidelines for Diagnosis and Treatment of Mycoplasma pneumoniae pneumonia in children (2023 edition) ". National Health Commission of the People's Republic of China. 6 February. 2023. Available online: https://www.gov.cn/zhengce/zhengceku/2023-02/16/content_5741770.htm
- "The Technical Regulation on Ambient Air Quality Index (AQI) (Trial) (HJ633-2012)". Ministry of Ecology and Environment of the People's Republic of China, 15 January. 2016. Available online: www.mee.gov.cn/ywgz/fgbz/bz/bzwb/jcffbz/201203/t20120302_224166.shtml
- Li YW, Lin JQ, Xiong HR, et al. The epidemiological and clinical characteristics of severe pneumonia in children (2020-2021). Electronic Journal of Emerging Infectious Diseases 2023;8:53-8.
- Atkinson TP, Balish MF, Waites KB. Epidemiology, clinical manifestations, pathogenesis and laboratory detection of Mycoplasma pneumoniae infections. FEMS Microbiol Rev 2008;32:956-73. [Crossref] [PubMed]
- Wang X, Li M, Luo M, et al. Mycoplasma pneumoniae triggers pneumonia epidemic in autumn and winter in Beijing: a multicentre, population-based epidemiological study between 2015 and 2020. Emerg Microbes Infect 2022;11:1508-17. [Crossref] [PubMed]
- Çakar A, Akyön Y, Gür D, et al. Investigation of carbapenemases in carbapenem-resistant Escherichia coli and Klebsiella pneumoniae strains isolated in 2014 in Turkey. Mikrobiyoloji bulteni. 2016;50:21-33. [Crossref] [PubMed]
- Xanthopoulou K, Urrutikoetxea-Gutiérrez M, Vidal-Garcia M, et al. First Report of New Delhi Metallo-β-Lactamase-6 (NDM-6) in a Clinical Acinetobacter baumannii Isolate From Northern Spain. Front Microbiol 2020;11:589253. [Crossref] [PubMed]
- Gong Y, Lu Y, Xue D, et al. Emergence of a Carbapenem-Resistant Klebsiella pneumoniae Isolate Co-harbouring Dual blaNDM- 6 -Carrying Plasmids in China. Front Microbiol 2022;13:900831. [Crossref] [PubMed]
- Huang K, Li HY, Chen MH, et al. Analysis of the clinical features and the risk factors of severe human metapneu movirus-associated community acquired pneumonia in children. Chinese Journal of Pediatrics 2023;61:322-7. [Crossref] [PubMed]
- Jacobs E, Ehrhardt I, Dumke R. New insights in the outbreak pattern of Mycoplasma pneumoniae. Int J Med Microbiol 2015;305:705-8. [Crossref] [PubMed]
- Fan F, Lv J, Yang Q, et al. Clinical characteristics and serum inflammatory markers of community-acquired mycoplasma pneumonia in children. Clin Respir J 2023;17:607-17. [Crossref] [PubMed]
- Yang HJ. Benefits and risks of therapeutic alternatives for macrolide resistant Mycoplasma pneumoniae pneumonia in children. Korean J Pediatr 2019;62:199-205. [Crossref] [PubMed]
- Zhu Z, Zhang T, Guo W, et al. Clinical characteristics of refractory mycoplasma pneumoniae pneumonia in children treated with glucocorticoid pulse therapy. BMC Infect Dis 2021;21:126. [Crossref] [PubMed]
- Rivaya B, Jordana-Lluch E, Fernández-Rivas G, et al. Macrolide resistance and molecular typing of Mycoplasma pneumoniae infections during a 4 year period in Spain. J Antimicrob Chemother 2020;75:2752-9. [Crossref] [PubMed]
- Liu H, Zhang Y, Yang J, et al. Application of mNGS in the Etiological Analysis of Lower Respiratory Tract Infections and the Prediction of Drug Resistance. Microbiol Spectr 2022;10:e0250221. [Crossref] [PubMed]
- Jiang FC, Wang RF, Chen P, et al. Genotype and mutation patterns of macrolide resistance genes of Mycoplasma pneumoniae from children with pneumonia in Qingdao, China, in 2019. J Glob Antimicrob Resist 2021;27:273-8. [Crossref] [PubMed]
- Faucher M, Nouvel LX, Dordet-Frisoni E, et al. Mycoplasmas under experimental antimicrobial selection: The unpredicted contribution of horizontal chromosomal transfer. PLoS Genet 2019;15:e1007910. [Crossref] [PubMed]
- Qu J, Zhang J, Chen Y, et al. Aetiology of severe community acquired pneumonia in adults identified by combined detection methods: a multi-centre prospective study in China. Emerg Microbes Infect 2022;11:556-66. [Crossref] [PubMed]
- Xu C, Deng H, Zhang J, et al. Mutations in domain V of Mycoplasma pneumoniae 23S rRNA and clinical characteristics of pediatric M. pneumoniae pneumonia in Nanjing, China. J Int Med Res 2021;49:3000605211016376. [Crossref] [PubMed]
- Hou W, Xu X, Lei Y, et al. The role of the PM2.5-associated metals in pathogenesis of child Mycoplasma Pneumoniae infections: a systematic review. Environ Sci Pollut Res Int 2016;23:10604-14. [Crossref] [PubMed]
- Jia H, Liu Y, Guo D, et al. PM2.5-induced pulmonary inflammation via activating of the NLRP3/caspase-1 signaling pathway. Environ Toxicol 2021;36:298-307. [Crossref] [PubMed]
- Zhao C, Liao J, Chu W, et al. Involvement of TLR2 and TLR4 and Th1/Th2 shift in inflammatory responses induced by fine ambient particulate matter in mice. Inhal Toxicol 2012;24:918-27. [Crossref] [PubMed]