Safety and efficacy of a novel quantitative phase I exercise in patients after cardiopulmonary bypass: a single-blind, prospective, non-randomized study
Highlight box
Key findings
• Quantitative phase I exercise with a novel cycle ergometer on the pulmonary function during the early postoperative period following cardiac surgery delivered additional improvements in both pulmonary and physical function domains with a shorter intensive care unit (ICU) stay and comparable total hospitalization costs and hospital stay, without additional adverse events compared with usual care.
What is known and what is new?
• Despite the positive effect of early post cardiac surgery exercises, concerns of safety issues are high, indicating the need for standardized and quantitative mobility indicators to enhance early mobilization monitoring in the ICU while ensuring patient safety.
• Quantitative phase I exercise applied to patients following cardiopulmonary bypass is safe and recovers respiratory and physical capacity more quickly.
What is the implication, and what should change now?
• Quantitative phase I exercise is a safe and feasible option for patients after cardiopulmonary bypass.
Introduction
Cardiovascular diseases are frequent diseases with considerable morbidity and mortality. Numerous heart conditions are treated by cardiac surgery, which in most cases involves cardiopulmonary bypass surgery. Despite recent notable advancements, postoperative morbidity (up to 70%) and pneumonia (2–15%) associated with cardiac surgery remain variable, depending on the specific surgical intervention, patient comorbidities, and frailty (1-4).
Enhanced recovery after surgery (ERAS) represents a comprehensive and interdisciplinary approach to optimize the perioperative management of patients, with the primary goal of facilitating early recovery, enhancing patient satisfaction, and reducing postoperative morbidity, mortality, and hospitalization duration (5). The ERAS pathway encompasses three distinct phases of the surgical process, namely preoperative, perioperative, and postoperative. Postoperative early mobilization after cardiac surgery is recommended to decrease postoperative morbidity and length of stay in the intensive-care unit (ICU) and hospital (6). Indeed, there is evidence that early rehabilitation starting within the first 2 weeks after operation (including cardiovascular, respiratory, and exercise rehabilitation) is associated with a lower incidence of pneumonia, improvement of functional capacity, and better respiratory function (7-11). Among these methods, exercise therapy is essential for a quick postoperative recovery.
Despite the positive effect of early postoperative exercises, there are still some concerns. For example, the intensity of activities is determined based on Borg scale, which is not an objective criterion. Another issue is outcome assessment. The 6-minute walk test (6MWT) is widely recognized as a dynamic indicator of cardiac function. However, its clinical implementation in patients following cardiac surgery is hindered by excessive drainage tubes and pain during the early stages of recovery, which may impede its feasibility. Moreover, the test results are highly subjective, which further complicates the clinical utility of the test.
While early mobilization in ICU certainly offers clinical benefits, patient safety is one of the most commonly reported barriers to delivering early mobilization (12). One reason is that it is difficult to objectively evaluate mobility intervention. Thus, the use of standardized indicators for documenting mobility, quantitative metrics to measure mobility, and trending mobility metrics over time are important to improve early mobility monitoring (13). While in-bed cycling has been used and recommended in ICU by various studies (9,14) and guideline (6) for early initiation of mobilization, there remains a lack of standardized and quantitative methods for setting exercise intensity and evaluating mobility objectively. In contrast, the use of a cycle ergometer as a novel treatment option in ICU offers a more objective approach, particularly among critically ill patients (15) and mechanically ventilated patients (16). However, its widespread utilization is more common among outpatients rather than within hospital settings.
The aim of this study was to examine the short-term effects of a novel lower limb rehabilitation program utilizing cycle ergometer with quantitative exercise prescriptions on the respiratory function of patients following cardiac surgery. The hypothesis of this study is that implementing a novel lower limb rehabilitation program utilizing a cycle ergometer with quantitative exercise prescriptions will lead to short-term improvements in respiratory function compared to usual care among patients following cardiac surgery. The results would provide standardized and high-quality data, supporting trend analysis to improve measurement and monitor early mobilization among patients following cardiac surgery. We present this article in accordance with the TREND reporting checklist (17) (available at https://jtd.amegroups.com/article/view/10.21037/jtd-24-753/rc).
Methods
Study design and participants
This trial was conducted at the Nanjing Drum Tower Hospital of the Affiliated Hospital of Nanjing University Medical School between November 5, 2022, and August 31, 2023. The patients were enrolled voluntarily after providing informed consent. The study was approved by the Ethics Committee of the Nanjing Drum Tower Hospital of the Affiliated Hospital of Nanjing University Medical School (No. 2022-467-03). Of note, it is not ethical to have a no-rehabilitation arm; therefore, usual care served as a control in this study. The trial was registered in the Chinese Clinical Trial Registry (No. ChiCTR2200065367). The study was conducted in accordance with the Declaration of Helsinki (as revised in 2013).
The study included the patients satisfying the following criteria: (I) undergoing on-pump open-heart cardiac surgery; (II) aged 18 years or above; (III) capable of completing bedside pedal exercises; (IV) 24 hours of cardiovascular stability without myocardial ischemia; and (V) willing to participate in the study.
The exclusion criteria were as follows: (I) postoperative cardiac and pulmonary dysfunction; (II) liver and kidney dysfunction; and (III) orthopedic, psychiatric, neurological problems (18,19).
After the first visit, eligible patients were allocated into the intervention group or the control group based on their willingness. As with most cardiopulmonary rehabilitation trials, the patients and providers could not be blind to group allocation.
Intervention
Due to fatigue and other treatment schedule of patients, it is hard for patients to complete daily rehabilitation consecutively for seven days. Therefore, we allowed patients to complete seven rehabilitation sessions within 14 days. Thus, all of the patients completed seven sessions therapy in total (one time per day) during 1–2 weeks’ hospital stay. The therapy was supervised individually with physical therapists with more than 3 years of experience.
The intervention group received cycle ergometer exercise combined with usual care. The control group received only usual care. Participants received personalized feedback on their progress during each session, highlighting their achievements and areas for improvement.
Cycle ergometer exercise
In the first two days post-operation in intensive care, the same physical therapist performed the manual muscle testing (MMT) of flexor and extensor in lower extremity, result showed most of the patients had an MMT grade lower than 5. To regain muscle strength, a multifunctional electromagnetic cycle ergometer was employed, with modes including passive exercise (for MMT grades 0 and 1), assisted exercise (for MMT grades 2 and 3), and active resistance exercise (for MMT grades 4 and 5; adjustable resistance from 0 to 100 watts). The patients were exercised until their MMT grade reached grade 5, and their cardiopulmonary function was subsequently tested.
At the first session, the patients wore a noninvasive cardiac output monitor device (Bioz-Plus, Shenzhen Medean Medical Equipment Co., Ltd., Shenzhen, China), which continuously monitored electrocardiogram, blood oxygen, and blood pressure. Continuous cycling (Innovative Exercise Rehabilitation Management System RE1000, Nanjing Hanya Health Technology Co., Ltd., RE Series Products, Nanjing, China) was performed with a gradual increase in power output, ranging from 1 to 4 watts per minute, reaching the symptom-limited maximum exercise intensity within 8–12 minutes. The peak power was recorded for setting the next exercise session intensity.
From the second to the seventh session, each session followed a five-stage protocol. The first stage (warm-up period) involved cycling without resistance for 2 minutes. In the second stage, power gradually increased, reaching the prescribed power output after 2 minutes (the power was set at 50% of the peak power achieved in the previous session). In the third stage, the constant power output was maintained for 10 minutes. In the fourth stage, the power was gradually decreased, reaching a minimum after 2 minutes. Finally, in the fifth stage (recovery period), cycling was performed without resistance for 2 minutes. Subsequently, this training was repeated daily with adjustments to power output based on the patient’s endurance.
Usual care
Passive and active rehabilitation training
Starting on the first day after surgery, nurses assisted the patients in passive hand and lower limb exercises. Hand exercises involved forceful gripping and extension of the fingers in a supine position, with 30 repetitions. Lower limb exercises included regular dorsal and plantar flexion of both feet, while the patient was in a supine position. Then patients were encouraged to mobilize for early ambulation such as sitting, standing and walking. The training session changed from passive to assisted and active according to the patient condition, with 30 repetitions.
Breathing training
Pursed-lip breathing
The patients performed deep inhalation through the nostrils, followed by slow exhalation through pursed lips, resembling a whistle. The exhalation lasted 4–6 seconds, and lip-pursing intensity was adjusted by the patient.
Diaphragmatic breathing
The patients adopted a comfortable posture and were instructed to place one hand on their chest and the other one on their abdomen. During inhalation, the chest had to remain as stable as possible, with the primary focus on abdominal expansion. Inhalation was supposed to occur through the nasal passages, and exhalation could utilize the pursed-lip technique.
Breathing exercises
The patients were guided through various breathing exercises, including diaphragmatic breathing with arm elevation and relaxation, followed by deep breaths, lifting both arms to 90 degrees during inhalation and lowering them during exhalation.
Forced expiration training
The patients were encouraged to perform relaxed but deep inhalation and utilize diaphragmatic breathing. The therapist guided the patients through forceful cough and abdominal muscle contraction. The patients were instructed to place both hands on their abdomen and perform three quick forced expirations, sensing abdominal muscle contraction. The patients also practiced making a “k” sound to feel vocal cord tension, glottis closure, and abdominal muscle contraction. When these actions were combined, the patients were guided to perform deep but relaxed inhalation followed by a rapid double cough.
The usual care was performed six times per set, once in the morning and once in the afternoon.
Outcomes
During the preoperative period (i.e., on the day before surgery), a direct interview was conducted with each subject. Data collected included sex, age, smoking, New York Heart Association (NYHA) class, comorbidities such as hypertension, diabetes mellitus, and chronic lung diseases. For the evaluation of anthropometric measurements, weight (kg), height (cm), and body mass index (kg/m2) were measured. The postoperative data regarding the surgical type, ICU length of stay, hospital length of stay, and adverse effects were collected from each subject’s medical records. The primary and secondary outcomes were assessed before the first session and after seven sessions of postoperative rehabilitation.
To determine the primary outcomes of pulmonary functional test (PFT), the participants in the intervention and control groups were tested with a portable lung function detector XEEK-X1 (Saike Medical Equipment Co., Ltd., China). During the test, forced vital capacity (FVC), forced expiratory volume in 1 s (FEV1), FEV1/FVC ratio, peak expiratory flow rate (PEF), and respiratory muscle strength indexes [maximum inspiratory pressure (MIP) and maximum expiratory pressure (MEP)] were measured.
The secondary outcome was 6MWT. The 6MWT measures the submaximal level of functional capacity. All of the patients underwent a 6MWT along the 30-meter Department’s flat corridor. The patients’ blood pressure was measured before and at the end of the test, and the heart rate and blood oxygen levels were monitored during the test. The total 6MWD was recorded. The maximum load (W), peak metabolic equivalent (METS), systolic blood pressure (SBP), diastolic blood pressure (DBP), peak heart rate, and exercise duration during the ergometer exercise were recorded at the first and last sessions.
All assessments were completed pre- and post-rehabilitation (see Figure 1). The same rehabilitation nurse performed the assessments at each time point, and was blinded to allocation, maintaining separate communication channels for the allocation information or discussing rehabilitation details with participants.
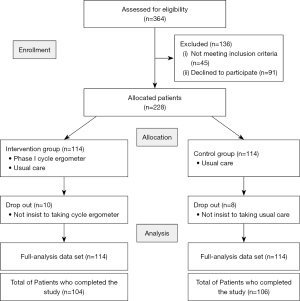
Statistical analysis
Sample size calculation was based on the expectation of FEV1 change was 0.32 L in the intervention group and 0.43 L in the control group (20). The G*Power 3.1.9.2 program was conducted using t tests to determine the required sample size for comparing means between two independent groups. The analysis assumed a two-tailed test with an effect size (Cohen’s d) of 0.4 (medium). The significance level (α) was set at 0.05, aiming for a statistical power (1–β) of 0.8. The allocation ratio between groups (N2/N1) was 1, resulting in a total sample size of 208 participants (104 per group). These parameters were used to ensure adequate statistical power for detecting meaningful differences between groups in the planned study. Considering an estimated 10% loss in our unpublished pilot study, 114 participants were planned to be included in each group (total N=228).
All statistical analyses were performed using the statistical software package IBM SPSS 26.0 (IBM Corp., Armonk, NY, USA), and the analyses were according to the intention-to-treat (ITT) principle to provide a more conservative estimate of treatment effect, with the significance set at P<0.05. The Shapiro-Wilk test was used to assess normality of data distribution. Differences in sociodemographic, clinical characteristics, and adverse effects between the groups were examined using the t-test for independent samples and the Chi-squared test. To analyze the differences in primary outcomes, 6MWD and cardiac function, a mixed model two-way repeated-measures analysis of variance (ANOVA) included the group (intervention and control groups) and test time (pre and post) was used. Significant main and interaction differences were examined. Analysis of covariance (ANCOVA) was used with any patient characteristic difference variables as covariates. Post hoc testing was performed using Bonferroni pair-wise tests outside the ANOVA for multiple comparisons. The P value was corrected based on the number of comparisons. For the ergometer exercise parameters, paired-samples t-test was used to analyze the differences with the interventional group.
Results
A total of 364 patients undergoing cardiac surgery were considered for their eligibility. Among them, 228 patients were assigned to the intervention group or the control group. During the study, 10 patients in the intervention group and 8 patients in the control group dropped out because they did not follow the rehabilitation. Finally, all 114 patients in each group were included in the ITT analysis (Figure 1).
Patients’ characteristics
Table 1 shows basic patients’ characteristics. There were more smokers in the intervention group. Postoperative ICU stay was shorter in the intervention group than in the control group. There were no significant differences in other patients’ characteristics between the groups. Valve-only was the most common surgery type in both groups.
Table 1
Variables | Intervention group (n=114) | Control group (n=114) | P value† |
---|---|---|---|
Age (years) | 60.4±11.9 | 57.6±14.9 | 0.14 |
Male | 64 (51.2) | 47 (37.6) | 0.33 |
Current/past smoker | 23 (18.4) | 8 (6.4) | 0.02* |
Weight (kg) | 63.2±10.3 | 62.2±11.9 | 0.50 |
Height (m) | 1.6±0.1 | 1.6±0.1 | 0.98 |
Body mass index (kg/m2) | 24.0±3.1 | 23.6±3.4 | 0.30 |
NYHA class at admission | 0.25 | ||
I | 4 (3.5) | 1 (0.9) | |
II | 53 (46.5) | 45 (39.5) | |
III | 53 (46.5) | 57 (50.0) | |
IV | 4 (3.5) | 11 (9.6) | |
Surgical characteristics (on pump) | |||
CABG-only | 24 (21.1) | 16 (14.0) | 0.35 |
Valve-only | 40 (35.1) | 50 (43.9) | |
CAGB + valve | 15 (13.2) | 11 (9.6) | |
Other‡ | 35 (30.7) | 37 (32.5) | |
Length of stay until surgery, days | 7.1±2.8 | 6.6±3.0 | 0.22 |
Length of postoperative ICU stay, days | 3.0±1.3 | 3.5±2.2 | 0.03* |
Length of postoperative general ward hospital stay, days | 8.7±4.4 | 8.5±3.9 | 0.71 |
Total hospitalization cost, ×105 yuan | 1.2±0.3 | 1.2±0.5 | 0.48 |
Comorbidities | |||
Chronic lung diseases | 62 (54.4) | 56 (49.1) | 0.68 |
Cerebrovascular diseases | 10 (8.8) | 14 (12.3) | 0.43 |
Diabetes mellitus | 16 (14.0) | 13 (11.4) | 0.25 |
Hypertension | 52 (45.6) | 36 (31.6) | 0.26 |
Pulmonary infection after surgery | 71 (62.3) | 53 (46.5) | 0.32 |
Pulmonary infection after rehabilitation | 0 | 0 | – |
Data are presented as mean ± standard deviation or n (%). ‡, in the intervention group: 35 aortic valve replacements and ascending aortic repairs; in the control group: 34 aortic valve replacements and ascending aortic repairs, two ventricular septal defects, and one tumor. †, independent-samples t-test or Chi-squared test. *, P<0.05. NYHA, New York Heart Association; CABG, coronary artery bypass graft.
PFT parameters, 6MWD, and cardiac function
Table 2 showed the differences of primary outcomes, 6MWD and cardiac function for different groups and test times. A significant group × test time interaction was found for FEV1, MEP, PEF and 6MWD. A significant main effect of group was found for 6MWD and significant main effect of test time was found except for LVEF (%).
Table 2
Variables | Intervention group (n=114) | Control group (n=114) | Group × test time | Group | Test time | |||||||||
---|---|---|---|---|---|---|---|---|---|---|---|---|---|---|
F | P | η² | F | P | η² | F | P | η² | ||||||
FEV1 (L) | 6.26 | 0.01* | 0.02 | 0.58 | 0.45 | 0.00 | 98.45 | <0.001* | 0.19 | |||||
Before 1st session | 0.66±0.29 | 0.73±0.29 | ||||||||||||
After 7th session | 1.10±0.39 | 0.99±0.43 | ||||||||||||
FVC (L) | 3.16 | 0.08 | 0.01 | 0.29 | 0.59 | 0.00 | 48.64 | <0.001* | 0.11 | |||||
Before 1st session | 0.86±0.45 | 0.93±0.41 | ||||||||||||
After 7th session | 1.32±0.67 | 1.20±0.51 | ||||||||||||
FEV1/FVC (%) | 2.43 | 0.12 | 0.01 | 0.04 | 0.84 | 0.00 | 11.61 | 0.001* | 0.03 | |||||
Before 1st session | 0.79±0.17 | 0.82±0.17 | ||||||||||||
After 7th session | 0.87±0.14 | 0.85±0.15 | ||||||||||||
MIP (cmH2O) | 1.74 | 0.19 | 0.00 | 0.12 | 0.73 | 0.00 | 145.74 | <0.001* | 0.26 | |||||
Before 1st session | 14.46±5.66 | 15.97±6.67 | ||||||||||||
After 7th session | 26.60±10.89 | 25.72±12.24 | ||||||||||||
MEP (cmH2O) | 5.36 | 0.02* | 0.01 | 3.23 | 0.07 | 0.01 | 118.43 | <0.001* | 0.22 | |||||
Before 1st session | 21.39±8.92 | 22.00±7.85 | ||||||||||||
After 7th session | 37.00±14.68 | 32.14±14.95 | ||||||||||||
PEF (L/min) | 4.03 | 0.045* | 0.01 | 1.56 | 0.21 | 0.00 | 109.56 | <0.01* | 0.21 | |||||
Before 1st session | 1.27±0.59 | 1.33±0.51 | ||||||||||||
After 7th session | 2.19±0.87 | 1.95±0.93w | ||||||||||||
6MWD (m) | 11.53 | 0.001* | 0.03 | 13.07 | <0.001* | 0.03 | 190.28 | <0.001* | 0.32 | |||||
Before 1st session | 148.0±51.4 | 146.6±55.8 | ||||||||||||
After 7th session | 253.2±75.1 | 210.3±63.9 | ||||||||||||
LVEF (%) | 0.03 | 0.87 | 0.00 | 0.29 | 0.59 | 0.00 | 2.70 | 0.10 | 0.01 | |||||
Before surgery | 57.2±8.1 | 56.9±9.0 | ||||||||||||
7 days after surgery | 56.0±6.9 | 55.5±7.7 | ||||||||||||
LVEDD (mm) | 0.09 | 0.77 | 0.00 | 0.49 | 0.49 | 0.00 | 32.99 | <0.001* | 0.07 | |||||
Before surgery | 52.9±7.6 | 52.7±8.3 | ||||||||||||
7 days after surgery | 49.2±5.4 | 48.6±6.0 | ||||||||||||
LVESD (mm) | 0.00 | 0.98 | 0.00 | 0.10 | 0.75 | 0.00 | 17.76 | <0.001* | 0.04 | |||||
Before surgery | 37.5±7.3 | 37.3±8.1 | ||||||||||||
7 days after surgery | 34.6±5.5 | 34.4±6.4 |
Data are presented as mean ± SD. †, two-way repeated measure ANOVA on group and test time. *, P<0.05. SD, standard deviation; PFT, pulmonary function test; FEV1, forced expiratory volume in 1 s; FVC, forced vital capacity; MIP, maximum inspiratory pressure; MEP, maximum expiratory pressure; PEF, peak expiratory flow rate; 6MWD, 6-minute walk test distance; LVEF, left ventricular ejection fraction; LVEDD, left ventricular end-diastolic diameter; LVESD, left ventricular end-systolic diameter.
Additionally, ANCOVA with smoking as covaries showed the same result with ANOVA except for the significant group × test time interaction in PEF (supplementary material, Table S1).
For the main effect of group, Bonferroni pair-wise tests (significant P value corrected to 0.025) revealed statistically significant difference in post-test compared to pre-test in all the parameters among intervention group, but except for FEV1/FVC (%) among control group (Table 3).
Table 3
Variables | Intervention group (n=114) | Control group (n=114) | Test result§ | P value | Effect size (d) |
---|---|---|---|---|---|
FEV1 (L) | |||||
Before 1st session | 0.66±0.29 | 0.73±0.29 | −1.502 | 0.14 | 0.241 |
After 7th session | 1.10±0.39 | 0.99±0.43 | 2.002 | 0.02* | −0.270 |
P value | <0.001* | <0.001* | |||
Test result | −13.856‡ | −6.364‡ | |||
FVC (L) | |||||
Before 1st session | 0.86±0.45 | 0.93±0.41 | −1.076 | 0.28 | 0.163 |
After 7th session | 1.32±0.67 | 1.20±0.51 | 2.078 | 0.02* | −0.202 |
P value | <0.001* | <0.001* | |||
Test result | −7.944‡ | −5.320‡ | |||
FEV1/FVC (%) | |||||
Before 1st session | 0.79±0.17 | 0.82±0.17 | −1.153 | 0.25 | 0.176 |
After 7th session | 0.87±0.14 | 0.85±0.15 | 1.053 | 0.30 | −0.139 |
P value | <0.001* | 0.07 | |||
Test result | −6.603‡ | −1.843‡ | |||
MIP (cmH2O) | |||||
Before 1st session | 14.46±5.66 | 15.97±6.67 | −1.769 | 0.08 | 0.244 |
After 7th session | 26.60±10.89 | 25.72±12.24 | −0.552 | 0.58 | −0.038 |
P value | <0.001* | <0.001* | |||
Test result | −13.477‡ | −9.750‡ | |||
MEP (cmH2O) | |||||
Before 1st session | 21.39±8.92 | 22.00±7.85 | −0.521 | 0.60 | 0.073 |
After 7th session | 37.00±14.68 | 32.14±14.95 | 2.367 | 0.02* | −0.328 |
P value | <0.001* | <0.001* | |||
Test result | −13.247‡ | −8.366‡ | |||
PEF (L/min) | |||||
Before 1st session | 1.27±0.59 | 1.33±0.51 | −0.723 | 0.47 | 0.109 |
After 7th session | 2.19±0.87 | 1.95±0.93 | 1.913 | 0.057 | −0.267 |
P value | <0.001* | <0.001* | |||
Test result | −13.067‡ | −7.496‡ | |||
6MWD (m) | |||||
Before 1st session | 148.0±51.4 | 146.6±55.8 | 0.180 | 0.86 | 0.026 |
After 7th session | 253.2±75.1 | 210.3±63.9 | 4.406 | <0.001* | −0.615 |
P value | <0.001* | <0.001* | |||
Test result | −19.743‡ | −16.039‡ | |||
△6MWD (m) | 105.3±56.7 | 63.7±38.9 | 6.076 | <0.001* | −0.856 |
LVEF (%) | |||||
Before surgery | 57.2±8.1 | 56.9±9.0 | 0.247 | 0.81 | −0.035 |
7 days after surgery | 56.0±6.9 | 55.5±7.7 | 0.544 | 0.59 | −0.068 |
P value | 0.02* | 0.01* | |||
Test result | 2.058‡ | 2.573‡ | |||
LVEDD (mm) | |||||
Before surgery | 52.9±7.6 | 52.7±8.3 | 0.247 | 0.81 | −0.025 |
7 days after surgery | 49.2±5.4 | 48.6±6.0 | 0.855 | 0.39 | −0.1.5 |
P value | <0.001* | <0.001* | |||
Test result | 8.197‡ | 8.873‡ | |||
LVESD (mm) | |||||
Before surgery | 37.5±7.3 | 37.3±8.1 | 0.188 | 0.85 | −0.026 |
7 days after surgery | 34.6±5.5 | 34.4±6.4 | 0.279 | 0.78 | −0.034 |
P value | <0.001* | <0.001* | |||
Test result | 6.861‡ | 6.024‡ |
Data are presented as mean ± standard deviation. †, Bonferroni pair-wise test. ‡, paired-samples t-test. §, independent-samples t-test. *, P<0.025. PFT, pulmonary function test; FEV1, forced expiratory volume in 1 s; FVC, forced vital capacity; MIP, maximum inspiratory pressure; MEP, maximum expiratory pressure; PEF, peak expiratory flow rate; 6MWD, 6-minute walk test distance; LVEF, left ventricular ejection fraction; LVEDD, left ventricular end-diastolic diameter; LVESD, left ventricular end-systolic diameter.
For the main effect of test time, Bonferroni pair-wise tests (significant P value corrected to 0.025) revealed none statistically significant difference among all the pre-test parameters, but with post-test difference between two groups in FEV1, FVC, MEP, 6MWD and △6MWD (Table 3). Moreover, the △6MWD increase in the intervention group was greater than that in the control group (Table 3).
In the intervention group, the ergometer exercise parameters improved significantly (P<0.001) (Table 4). All of the patients experienced significantly improved maximum load maximal exercise capacity, and exercise duration at the end of the training period.
Table 4
Variables | Intervention group (n=114) | Test result† | P value |
---|---|---|---|
Maximum load (W) | −5.774 | <0.001* | |
1st session | 18.18±10.52 | ||
7th session | 25.38±6.60 | ||
Maximum METs | −7.487 | <0.001* | |
1st session | 1.70±0.84 | ||
7th session | 2.38±0.50 | ||
SBP (mmHg) | 2.577 | 0.01* | |
1st session | 125.7±17.3 | ||
7th session | 120.1±18.5 | ||
DBP (mmHg) | −3.930 | <0.001* | |
1st session | 63.7±9.0 | ||
7th session | 69.2±10.4 | ||
Peak heart rate (beats/min) | −2.517 | 0.01* | |
1st session | 88.7±16.3 | ||
7th session | 93.7±14.8 | ||
Exercise duration (min) | −10.596 | <0.001* | |
1st session | 10.9±3.9 | ||
7th session | 15.3±2.2 |
Data are presented as mean ± standard deviation. †, paired-samples t-test. *, P<0.025. METs, metabolic equivalents; SBP, systolic blood pressure; DBP, diastolic blood pressure.
Pulmonary complications
After operation, there was no significant difference in the occurrence of pulmonary infections between the intervention group and the control group (Table 1). After rehabilitation, all of the patients were without pulmonary infection.
Discussion
This study evaluated the safety and efficacy of quantitative phase I exercise with a novel cycle ergometer on the pulmonary function during the early postoperative period following cardiac surgery. With the precise evaluation of power during cycle ergometry, the intervention group protocol delivered additional improvements relative to the control group, in both pulmonary and physical function domains. Furthermore, quantitative phase I exercise was associated with a shorter ICU stay and comparable total hospitalization costs and hospital stay, and there were no additional adverse events compared with usual care.
Pulmonary complications after cardiac surgery are common due to significant hemodynamic and physiologic alterations (21). Pulmonary dysfunction is a significant complication, which results in notable reductions in lung volumes, vital capacity, and oxygenation, as well as a 30–50% decrease in FEV1, FVC, and functional residual capacity (22). Early postoperative mobilization within the first 2 weeks following cardiac surgery is strongly recommended to reduce postoperative complications and length of stay in hospital. There is early evidence that direct respiratory function rehabilitation using incentive spirometer (23) and expiratory muscle training device (24) can effectively improve dynamic lung volumes (FEV, FEV1, FVC) and respiratory muscle strength (MIP, MEP). The lack of randomization allowed the majority of smoking patients to go to the intervention group. More smokers may attenuate the pulmonary function improvement induced by the intervention, yet the results demonstrate a more significant improvement in lung function (in FEV1, MEP and PEF) among patients in the intervention group. Analyses adjusting for smoking as a covariate did not show significant PEF improvement in the intervention group, suggesting that smoking may diminish PEF improvement, which needs confirmation in future studies. Previous studies compared the pulmonary function at the time before operation and after rehabilitation to evaluate the effect of rehabilitation (8,23,25). Unlike previous studies, we directly compared the pulmonary function before the first session and after seven sessions of postoperative rehabilitation, which allowed a more precise detection of the rehabilitation effect.
Previous studies have shown that breathing exercise (e.g., respiratory and inspiratory muscle exercises) could enhance 6MWD after cardiac surgery (8,26). In specific conditions, such as in elderly patients, lower training volume and longer recovery periods may be sufficient (27). The current study showed enhanced 6MWD in both groups, with higher overall changes in the exercise group relative to the control group (105.3 vs. 63.7 m). The minimal clinically important difference (MCID) for 6MWT during rehabilitation was 25 m in coronary artery disease (28) and 14–30 m in other diseases (such as chronic obstructive pulmonary disease) (29). It is worth noting that the baseline 6MWD in previous studies ranged from 295 to 551 m (29), which is nearly two times higher than what we found in the current study (148.0 m in the intervention group and 146.6 m in the control group). Although patients in the current study had a lower baseline 6MWD, the improvement after rehabilitation was much higher than MCID. In a recent study that has investigated the effect of pulmonary rehabilitation in patients with open-heart cardiac surgery (on-pump), which had similar patients to our study, 6MWD improved from 107 to 177 m (19). Considering that the clinical care was 4 days in the previous study, the higher improvement in 6MWT in our study might be related to more rehabilitation sessions.
The precise definition of exercise intensity plays a crucial role in deciding whether to continue or suspend therapy. During phase I of cardiac rehabilitation, a focus on low-intensity exercise is paramount, aiming to optimize the patient’s physical and psychological well-being before hospital discharge (30). As for the duration of exercise in phase I, the South American Guidelines for Cardiovascular Prevention and Rehabilitation (31) suggest 40–60 minutes per day, and Japanese guidelines (32) suggest at least 10 minutes with a final goal of 20–60 minutes of aerobic exercise. Nevertheless, there is a lack of consensus regarding the optimal therapy duration for phase I of cardiovascular rehabilitation. Regardless of the technique or exercise type used for mobilization, the essential point is to avoid bed rest. However, for patients that could not ambulate due to pain or intubation, the in-bed rehabilitation with a cycle ergometer used in the current study is safe and feasible (14). Our results support the evidence that patients adhere well to the cycle ergometer exercise, without drop out and adverse events during the intervention.
For early mobilization in the ICU, prioritizing risk management during rehabilitation is of utmost importance. In that context, safety standards for early mobilization (33) and cancellation criteria (34) are necessary for risk management. A previous study investigated the safety and effect of early mobilization in the ICU according to cancellation criteria, including setting specific rehabilitation goals based on the patient’s condition, considering factors such as pain level, respiratory and circulatory status, scale Richmond Agitation-Sedation Scale (delirium), or Glasgow scale score for consciousness and sedation level (35). The study showed no serious mobilization-related adverse events during the study period. The present study used a noninvasive cardiac output monitoring device, which is a more objective method for risk management. In addition, our research findings may provide a new reference approach for risk management in phase I postoperative cardiac rehabilitation. In terms of risk management, the initiation moment of mobilization should not be too early or too late, and the current study also revealed that the protocol starting from the first day after open-heart cardiac surgery was safe and effective in improving pulmonary and physical function.
The safety is one of the most commonly reported barriers to delivering early mobilization (12) following cardiac surgery. Although the incidence rate of adverse events such as high heart rate, low blood pressure, or oxygen desaturation is low (2%) and rarely results in additional care requirements (36), poor initial psychological feelings would affect subsequent mobilization (37).
The barriers also include unclear protocol criteria. Majority of therapies are of progressive mobilization. In few studies, early mobilization following cardiac surgery has been conducted using cycle ergometer (38-40); however, none of the protocols were based on quantified amount and the indicators used to monitor patients’ response during exercise were subjective (such as Borg scale or participants’ feeling, heart rate change from 20 to 30 beats/min above the resting heart rate, speech test). Some of the studies had a relatively small sample size (n=24 or n=31), and only physical function was investigated.
The strength of this trial is in the use of precise evaluation of power during cycle ergometry to develop the exercise prescriptions individually. Each training session was monitored by experienced physical therapists; heart rate was monitored during each session; and power test before each session allowed the objective and progressive mobilization. Collectively, these findings confirmed that training intensity and duration were enhanced in the intervention group. Moreover, the absence of adverse events during the intervention revealed that the rehabilitation program used in our study could both ensure the exercise intensity and safety during early mobilization.
Both the exercise and usual care protocols presented in this study showed benefits to patients, the intervention group protocol delivered additional improvements, mainly in the primary outcome variables. Also, independently from the protocol used, no intercurrence was observed during the rehabilitation sessions. This indicates that the interventions ensured patient recovery and safety in the performance of physical therapy. The usual care of the control group consisted of muscle exercise and respiratory physiotherapy which was routinely employed following cardiac surgery to prevent and treat postoperative pulmonary complications (41-43), thus despite with non-randomized design, it is probable that the results will be generalizable to a wider clinical community.
There are some limitations in this study. To date, there has been no evidence of improved long-term outcomes after cardiac surgery other than coronary artery bypass graft. The surgical type was also diverse in our study. Therefore, the current quantitative phase I exercise should be applied in a single surgical type in the future, and the long-term effects along with the activity of daily living and quality of life should be evaluated. As the non-randomized design, it is not possible to completely rule out that more smokers and shorter ICU stays in intervention group could affect the results, which needs to be verified in future randomized controlled trials.
Conclusions
To the best of our knowledge, this is the first clinical trial to reveal that the short-term quantitative phase I exercise applied to patients who have undergone open-heart cardiac surgery is safe and can help patients recover their respiratory function more quickly. This study highlights the need for further development of interventions to optimize measurement and monitoring of early mobilization among patients following cardiac surgery with randomized design trials to support the findings.
Acknowledgments
We thank the patients and staff of the Division of Rehabilitation, Nanjing Drum Tower Hospital of the Affiliated Hospital of Nanjing University Medical School for their valuable contributions to this study.
Funding: None.
Footnote
Reporting Checklist: The authors have completed the TREND reporting checklist. Available at https://jtd.amegroups.com/article/view/10.21037/jtd-24-753/rc
Data Sharing Statement: Available at https://jtd.amegroups.com/article/view/10.21037/jtd-24-753/dss
Peer Review File: Available at https://jtd.amegroups.com/article/view/10.21037/jtd-24-753/prf
Conflicts of Interest: All authors have completed the ICMJE uniform disclosure form (available at https://jtd.amegroups.com/article/view/10.21037/jtd-24-753/coif). The authors have no conflicts of interest to declare
Ethical Statement: The authors are accountable for all aspects of the work in ensuring that questions related to the accuracy or integrity of any part of the work are appropriately investigated and resolved. The study was conducted in accordance with the Declaration of Helsinki (as revised in 2013). The study was approved by the Ethics Committee of the Nanjing Drum Tower Hospital of the Affiliated Hospital of Nanjing University Medical School (No. 2022-467-03). Informed consent was obtained from all individual participants.
Open Access Statement: This is an Open Access article distributed in accordance with the Creative Commons Attribution-NonCommercial-NoDerivs 4.0 International License (CC BY-NC-ND 4.0), which permits the non-commercial replication and distribution of the article with the strict proviso that no changes or edits are made and the original work is properly cited (including links to both the formal publication through the relevant DOI and the license). See: https://creativecommons.org/licenses/by-nc-nd/4.0/.
References
- Kinlin LM, Kirchner C, Zhang H, et al. Derivation and validation of a clinical prediction rule for nosocomial pneumonia after coronary artery bypass graft surgery. Clin Infect Dis 2010;50:493-501. [Crossref] [PubMed]
- Wang D, Huang X, Wang H, et al. Risk factors for postoperative pneumonia after cardiac surgery: a prediction model. J Thorac Dis 2021;13:2351-62. [Crossref] [PubMed]
- Engelman DT, Ben Ali W, Williams JB, et al. Guidelines for Perioperative Care in Cardiac Surgery: Enhanced Recovery After Surgery Society Recommendations. JAMA Surg 2019;154:755-66. [Crossref] [PubMed]
- Patra C, Chamaiah Gatti P, Panigrahi A. Morbidity After cardiac surgery under cardiopulmonary bypass and associated factors: A retrospective observational study. Indian Heart J 2019;71:350-5. [Crossref] [PubMed]
- Engelman RM, Rousou JA, Flack JE 3rd, et al. Fast-track recovery of the coronary bypass patient. Ann Thorac Surg 1994;58:1742-6. [Crossref] [PubMed]
- Mertes PM, Kindo M, Amour J, et al. Guidelines on enhanced recovery after cardiac surgery under cardiopulmonary bypass or off-pump. Anaesth Crit Care Pain Med 2022;41:101059. [Crossref] [PubMed]
- Herdy AH, Marcchi PL, Vila A, et al. Pre- and postoperative cardiopulmonary rehabilitation in hospitalized patients undergoing coronary artery bypass surgery: a randomized controlled trial. Am J Phys Med Rehabil 2008;87:714-9. [Crossref] [PubMed]
- Savci S, Degirmenci B, Saglam M, et al. Short-term effects of inspiratory muscle training in coronary artery bypass graft surgery: a randomized controlled trial. Scand Cardiovasc J 2011;45:286-93. [Crossref] [PubMed]
- Hirschhorn AD, Richards DA, Mungovan SF, et al. Does the mode of exercise influence recovery of functional capacity in the early postoperative period after coronary artery bypass graft surgery? A randomized controlled trial. Interact Cardiovasc Thorac Surg 2012;15:995-1003. [Crossref] [PubMed]
- Doyle MP, Indraratna P, Tardo DT, et al. Safety and efficacy of aerobic exercise commenced early after cardiac surgery: A systematic review and meta-analysis. Eur J Prev Cardiol 2019;26:36-45. [Crossref] [PubMed]
- Tashiro N, Takahashi S, Takasaki T, et al. Efficacy of cardiopulmonary rehabilitation with adaptive servo-ventilation in patients undergoing off-pump coronary artery bypass grafting. Circ J 2015;79:1290-8. [Crossref] [PubMed]
- Hodgson CL, Capell E, Tipping CJ. Early Mobilization of Patients in Intensive Care: Organization, Communication and Safety Factors that Influence Translation into Clinical Practice. Crit Care 2018;22:77. [Crossref] [PubMed]
- Fazio SA, Doroy AL, Anderson NR, et al. Standardisation, multi-measure, data quality and trending: A qualitative study on multidisciplinary perspectives to improve intensive care early mobility monitoring. Intensive Crit Care Nurs 2021;63:102949. [Crossref] [PubMed]
- Newman ANL, Kho ME, Harris JE, et al. The experiences of cardiac surgery critical care clinicians with in-bed cycling in adult patients undergoing complex cardiac surgery. Disabil Rehabil 2022;44:5038-45. [Crossref] [PubMed]
- dos Santos LJ, de Aguiar Lemos F, Bianchi T, et al. Early rehabilitation using a passive cycle ergometer on muscle morphology in mechanically ventilated critically ill patients in the Intensive Care Unit (MoVe-ICU study): study protocol for a randomized controlled trial. Trials 2015;16:383. [Crossref] [PubMed]
- Berney S, Hopkins RO, Rose JW, et al. Functional electrical stimulation in-bed cycle ergometry in mechanically ventilated patients: a multicentre randomised controlled trial. Thorax 2021;76:656-63. [Crossref] [PubMed]
- Des Jarlais DC, Lyles C, Crepaz N, et al. Improving the reporting quality of nonrandomized evaluations of behavioral and public health interventions: the TREND statement. Am J Public Health 2004;94:361-6. [Crossref] [PubMed]
- Tamulevičiūtė-Prascienė E, Beigienė A, Thompson MJ, et al. The impact of additional resistance and balance training in exercise-based cardiac rehabilitation in older patients after valve surgery or intervention: randomized control trial. BMC Geriatr 2021;21:23. [Crossref] [PubMed]
- Girgin Z, Ciğerci Y, Yaman F. The Effect of Pulmonary Rehabilitation on Respiratory Functions, and the Quality of Life, following Coronary Artery Bypass Grafting: A Randomised Controlled Study. Biomed Res Int 2021;2021:6811373. [Crossref] [PubMed]
- Alaparthi GK, Augustine AJ, Anand R, et al. Comparison of Diaphragmatic Breathing Exercise, Volume and Flow Incentive Spirometry, on Diaphragm Excursion and Pulmonary Function in Patients Undergoing Laparoscopic Surgery: A Randomized Controlled Trial. Minim Invasive Surg 2016;2016:1967532. [Crossref] [PubMed]
- Tanner TG, Colvin MO. Pulmonary Complications of Cardiac Surgery. Lung 2020;198:889-96. [Crossref] [PubMed]
- Chen JO, Liu JF, Liu YQ, et al. Effectiveness of a perioperative pulmonary rehabilitation program following coronary artery bypass graft surgery in patients with and without COPD. Int J Chron Obstruct Pulmon Dis 2018;13:1591-7. [Crossref] [PubMed]
- Alwekhyan SA, Alshraideh JA, Yousef KM, et al. Nurse-guided incentive spirometry use and postoperative pulmonary complications among cardiac surgery patients: A randomized controlled trial. Int J Nurs Pract 2022;28:e13023. [Crossref] [PubMed]
- Crisafulli E, Venturelli E, Siscaro G, et al. Respiratory muscle training in patients recovering recent open cardiothoracic surgery: a randomized-controlled trial. Biomed Res Int 2013;2013:354276. [Crossref] [PubMed]
- Chen S, Li X, Wu Y, et al. Preoperative respiratory muscle training combined with aerobic exercise improves respiratory vital capacity and daily life activity following surgical treatment for myasthenia gravis. J Cardiothorac Surg 2023;18:160. [Crossref] [PubMed]
- Cordeiro AL, de Melo TA, Neves D, et al. Inspiratory Muscle Training and Functional Capacity in Patients Undergoing Cardiac Surgery. Braz J Cardiovasc Surg 2016;31:140-4. [Crossref] [PubMed]
- Lavie CJ, Milani RV, Marks P, et al. Exercise and the heart: risks, benefits, and recommendations for providing exercise prescriptions. Ochsner J 2001;3:207-13.
- Gremeaux V, Troisgros O, Benaïm S, et al. Determining the minimal clinically important difference for the six-minute walk test and the 200-meter fast-walk test during cardiac rehabilitation program in coronary artery disease patients after acute coronary syndrome. Arch Phys Med Rehabil 2011;92:611-9. [Crossref] [PubMed]
- Bohannon RW, Crouch R. Minimal clinically important difference for change in 6-minute walk test distance of adults with pathology: a systematic review. J Eval Clin Pract 2017;23:377-81. [Crossref] [PubMed]
- Sebastian LA, Reeder S, Williams M. Determining target heart rate for exercising in a cardiac rehabilitation program: a retrospective study. J Cardiovasc Nurs 2015;30:164-71. [Crossref] [PubMed]
- Herdy AH, López-Jiménez F, Terzic CP, et al. South American guidelines for cardiovascular disease prevention and rehabilitation. Arq Bras Cardiol 2014;103:1-31. [Crossref] [PubMed]
- Makita S, Yasu T, Akashi YJ, et al. JCS/JACR 2021 Guideline on Rehabilitation in Patients With Cardiovascular Disease. Circ J 2022;87:155-235. [Crossref] [PubMed]
- Hodgson CL, Stiller K, Needham DM, et al. Expert consensus and recommendations on safety criteria for active mobilization of mechanically ventilated critically ill adults. Crit Care 2014;18:658. [Crossref] [PubMed]
- Sakai T, Jinno T, Hoshino C, et al. Cancellation Criteria of Acute Rehabilitation: Rehabilitation Risk Management. Prog Rehabil Med 2019;4:20190013. [Crossref] [PubMed]
- Sakai T, Hoshino C, Okawa A, et al. The Safety and Effect of Early Mobilization in the Intensive Care Unit According to Cancellation Criteria. Prog Rehabil Med 2020;5:20200016. [Crossref] [PubMed]
- Nydahl P, Sricharoenchai T, Chandra S, et al. Safety of Patient Mobilization and Rehabilitation in the Intensive Care Unit. Systematic Review with Meta-Analysis. Ann Am Thorac Soc 2017;14:766-77. [Crossref] [PubMed]
- Choi J, Tasota FJ, Hoffman LA. Mobility interventions to improve outcomes in patients undergoing prolonged mechanical ventilation: a review of the literature. Biol Res Nurs 2008;10:21-33. [Crossref] [PubMed]
- Gama Lordello GG, Gonçalves Gama GG, Lago Rosier G, et al. Effects of cycle ergometer use in early mobilization following cardiac surgery: a randomized controlled trial. Clin Rehabil 2020;34:450-9. [Crossref] [PubMed]
- Trevisan MD, Lopes DG, Mello RG, et al. Alternative Physical Therapy Protocol Using a Cycle Ergometer During Hospital Rehabilitation of Coronary Artery Bypass Grafting: a Clinical Trial. Braz J Cardiovasc Surg 2015;30:615-9. [Crossref] [PubMed]
- Windmöller P, Bodnar ET, Casagrande J, et al. Physical Exercise Combined With CPAP in Subjects Who Underwent Surgical Myocardial Revascularization: A Randomized Clinical Trial. Respir Care 2020;65:150-7. [Crossref] [PubMed]
- Westerdahl E, Urell C, Jonsson M, et al. Deep breathing exercises performed 2 months following cardiac surgery: a randomized controlled trial. J Cardiopulm Rehabil Prev 2014;34:34-42. [Crossref] [PubMed]
- Westerdahl E. Optimal technique for deep breathing exercises after cardiac surgery. Minerva Anestesiol 2015;81:678-83.
- Rehabilitation AoCPiC. ACPICR Standards for Physical Activity and Exercise in the Cardiovascular Population. 2015.