Sivelestat sodium alleviated sepsis-induced acute lung injury by inhibiting TGF-β/Smad signaling pathways through upregulating microRNA-744-5p
Highlight box
Key findings
• In the model of acute lung injury (ALI), the expression of microRNA (miR)-744-5p was down-regulated.
• Sivelestat sodium (SV) increased in the level of miR-744-5p, suppressed the inflammatory response, and ultimately improved lung injury.
• SV inhibited the transforming growth factor-β/Smad signaling pathway by miR-744-5p, thereby reducing sepsis-induced ALI.
What is known and what is new?
• The sepsis-induced ALI often triggers systemic inflammatory response syndrome. The expression of some miRNAs (miR-181a and miR-377-3p) is reduced in the sepsis-induced ALI.
• The expression of miR-744-5p was down-regulated in the model of ALI. SV can inhibit the occurrence and development of sepsis-induced ALI by enhancing the expression of miR-744-5p, thus suppressing the inflammatory response.
What is the implication, and what should change now?
• In our study, we first discovered that miR-744-5p expression was significantly decreased in lung tissues of sepsis-induced ALI. By increasing the expression of miR-744-5p, SV inhibited severe inflammatory response, thus suppressing the occurrence of septic ALI and providing a new target for the treatment of septic ALI.
Introduction
In critically ill patients after surgery, sepsis is a frequent complication that arises from a bacterial infection and leads to a systemic inflammatory response. Among the organs affected by sepsis, the lung is particularly susceptible, and acute lung injury (ALI) is a prevalent complication associated with sepsis, often resulting in fatal outcomes for critically ill patients (1). Epidemiological studies on ALI caused by sepsis show that approximately 48.9 million people were diagnosed with sepsis worldwide in 2017, and nearly 1/5 of the deaths were due to sepsis (2). Systemic inflammatory response syndrome characterized by refractory hypoxemia and respiratory distress is often observed in sepsis-induced ALI (1,3). Neutrophils, alveolar macrophages, lymphocytes, and alveolar epithelial cells are the primary cells participating in the inflammation of ALI (4). In particular, neutrophils tend to cluster together in the lungs, leading to an elevation in the levels of inflammatory cytokines, which results in harm to the integrity of the alveolar epithelium and pulmonary microvascular endothelium, ultimately contributing to lung injury (5). Although there has been a steady growth in research concerning ALI, the current treatment approaches predominantly revolve around supportive therapies like mechanical ventilation (6,7), which unfortunately do not contribute to improving the prognosis of ALI. Hence, it becomes important to explore an efficacious treatment for this condition actively.
Inflammatory cytokines, which include members of the transforming growth factor-β (TGF-β) superfamily and interleukins are the main driving force behind ALI (8). TGF-β has the ability to regulate proliferation, differentiation, apoptosis, adhesion, and migration in a variety of cells, including neutrophils, macrophages, activated T cells and B cells (9). The signaling pathways responsible for transmitting TGF-β signals inside cells are referred to as the Smad-dependent and Smad-independent pathways (10). According to research, Smad3 is found to be harmful in different inflammatory conditions, whereas Smad2 and Smad7 have a protective effect (11). TGF-β also has the ability to regulate the expression of multiple microRNAs (miRNAs), either in a positive or negative manner. For instance, the TGF-β1/Smad3 signaling pathway controls the regulation of both miR-192 and miR-21, which have been implicated in the development of kidney diseases (12).
Sivelestat sodium (SV), a highly specific neutrophil elastase inhibitor, acts by inhibiting neutrophil elastase activity and intervening in neutrophil-mediated inflammation (13). Multiple inflammatory diseases have provided evidence of SV’s anti-inflammatory properties and ability to protect tissues (5,14-16). The severity of lung tissue damage can be alleviated by SV, as it effectively reduces the release of inflammatory mediators and inhibits cell apoptosis through suppressing neutrophil elastase activity, according to the research (17). Additionally, in a retrospective cohort study, SV improved lung injury syndrome associated with acute respiratory distress syndrome (ARDS) in children aged from 28 days to 18 years old (18). Consequently, the mechanism of SV in the treatment of ALI is gaining more attention.
miRNAs are a type of small non-coding RNAs. Since being discovered in 1993, miRNAs have been extensively investigated in biomedical research due to their ability to regulate the translation process of specific protein-coding genes (19). The crucial role of miRNA in the onset and progression of inflammatory pulmonary diseases, including ALI, has been established by numerous studies. For instance, the study conducted by Fu et al. demonstrated that protecting mice from the effects of ALI could be achieved by targeting Bcl-2 and decreasing the expression of miR-181a (20) and according to recent findings from Wei et al., extracellular vesicle-released miR-377-3p from mesenchymal stem cells enhances autophagy, thereby improving lipopolysaccharide (LPS)-induced ALI through targeting regulatory-associated protein of mTOR (RPTOR) (21). Nevertheless, the extent to which miRNAs participate in the development of ALI, particularly in relation to inflammation, remains largely uncertain. Consequently, comprehending how miRNAs regulate inflammatory responses presents an appealing strategy in the management of ALI disorders.
In this study, we employed microarray analysis to examine the miRNA expression pattern in lung tissues and discovered that miR-744-5p may play a role in controlling the progression of ALI inflammation. Additionally, we have shown that SV can alleviate sepsis-induced ALI by suppressing the TGF-β/Smad signaling pathways, and this is achieved by increasing the levels of miR-744-5p. These outcomes offer fresh research approaches for the molecular therapeutic target of sepsis-induced ALI to a certain degree. We present this article in accordance with the ARRIVE reporting checklist (available at https://jtd.amegroups.com/article/view/10.21037/jtd-24-65/rc).
Methods
Animal models
All C57BL/6 mice aged 10±2 weeks and weighing 18–22 g were purchased from Sibeifu Biotechnology Co., Ltd. (Beijing, China). The mice were placed in a specific pathogen free (SPF) environment with a temperature of 23±2 ℃ and a light period of 12 h. These animals had unrestricted access to food and water. Mice were anesthetized with 1% pentobarbital sodium [50 mg/kg, intraperitoneal (i.p.) injection], and cecal ligation puncture (CLP) was used to establish a mouse sepsis model (22). After that, all mice were randomly divided into six groups (n=8) to study the effect of SV on sepsis-induced ALI, including: (I) sham-operated group; (II) CLP group; (III) CLP + negative control (NC) group; (IV) CLP + antagomir-744-5p group; (V) CLP + SV group; (VI) CLP + SV + antagomir-744-5p group. The sham group solely underwent laparotomy without the procedure of ligation and perforation of the cecum. At 1-h post-surgery, the SV group was administered an i.p. injection of SV (Selleck, USA) at a dosage of 100 mg/kg according to a previous study (23), whereas the sham group and CLP group were given an equivalent dose of saline through injection. The injection of has-miR-744-5p-antagomir (MCE, China) in the antagomir-744-5p group was administered at a dosage of 120 nmol/kg (i.p.). In the CLP + NC group, an equivalent amount of NC antagomir was injected.
Experiments were performed under a project license (No. HYLL-2021-113) granted by the institutional ethics board of The First Affiliated Hospital of Hainan Medical University, in compliance with The First Affiliated Hospital of Hainan Medical University institutional guidelines for the care and use of animals.
Cell culture
Human pulmonary microvascular endothelial cells (HPMECs) were purchased from ATCC (USA), and maintained at 37 ℃ in Dulbecco’s modified Eagle medium (DMEM) supplemented with 10% FBS (BI, USA), 1% penicillin/streptomycin in an atmosphere that has been humidified and contains 5% CO2. The cells were divided into six groups, including: (I) control group; (II) LPS group; (III) LPS + NC group; (IV) LPS + miR-744-5p inhibitor group; (V) LPS + SV group; (VI) LPS + SV + miR-744-5p inhibitor group. The control group cells were cultured for 24 h without any treatment. The LPS group cells were cultured for 24 h after being treated with 2 µg/mL LPS (Sigma-Aldrich, USA). The SV group cells were initially treated with 100 µg/mL SV for 2 h, followed by co-treatment with LPS for 24 h. The miR-744-5p inhibitor group cells underwent the recommended procedure for hsa-miR-744-5p inhibitor (MCE), and all cells were cultured for 24 h and collected for the experiment.
Quantitative real-time polymerase chain reaction (qRT-PCR)
The SYBR Premix Ex Taq II (Takara, Japan) was used for conducting qRT-PCR analysis on the Roche Light Cycler 480 system (Roche, UK). In the same sample, the mRNA level of the target gene was standardized using GAPDH as the reference gene. The primer sequences for detecting qRT-PCR were provided below: miR-744-5p: forward, 5'-AATGCGGGGCTAGGGCTA-3', reverse, 5'-GTGCAGGGTCCGAGGT-3'; Il1β: forward, 5'-ATGATGGCTTATTACAGTGGCAA-3', reverse, 5'-GTCGGAGATTCGTAGCTGGA-3'; Tnfα: forward, 5'-AGCTACGAATCTCCGACCAC-3', reverse, 5'-CGTTATCCCATGTGTCGAAGAA-3'; Il6: forward, 5'-ACTCACCTCTTCAGAACGAATTG-3', reverse, 5'-CCATCTTTGGAAGGTTCAGGTTG-3'; Ccl2: forward, 5'-CCATCTTTGGAAGGTTCAGGTTG, reverse, 5'-GCATTAGCTTCAGATTTACGGGT-3'; GAPDH: forward, 5'-CACCCACTCCTCCACCTTTG-3', reverse, 5'-CCACCACCCTGTTGCTGTAG-3'.
Enzyme-linked immunosorbent assay (ELISA)
After sacrificing the small mouse, its lungs were rinsed with 1 mL of phosphate buffered saline (PBS) for three times. This will facilitate the collection of bronchoalveolar lavage fluid (BALF). Subsequently, employ an ELISA kit to analyze the inflammatory factors within the BALF, such as IL-1β, IL-6, and tumor necrosis factor-α (TNF-α).
For cell experiments, the culture medium was collected and the inflammatory factors within were detected after cell treatment was completed. These kits were obtained from Beyotime (China). The corresponding ELISA kits were used to detect the levels of IL-6, IL-1β, and TNF-α in BALF and cells. Each experiment was conducted three separate times.
Hematoxylin-eosin (H&E), Masson and immunohistochemistry (IHC) staining
The mouse lung tissue samples undergo a series of steps, starting with fixation, followed by slicing, and deparaffinization, the following steps are performed: dewaxing, rehydration, antigen detection, and blocking with 5% bovine serum albumin (BSA) for 1 h at room temperature (RT, 26 ℃). Following that, the primary antibody is incubated overnight at 4 ℃, and then the secondary antibody labeled with biotin is incubated at RT for 1 h. Finally, the microscope is used to record the images and the lung injury pathology score was arranged based on the observed findings.
Cell viability assay (CCK-8)
About 1×104 cells HPMECs were added to every well of a 96-well culture plate. Following 12 h incubation with 5% CO2 at 37 ℃, the cells were exposed to SV and LPS for a duration of 24 h. To determine cell viability, a CCK-8 assay kit (Beyotime) was utilized and the measurements were conducted as per the instructions provided by the manufacturer.
Western blot assay
The radio immunoprecipitation assay (RIPA) lysis buffer (Solarbio, China) was used to extracte total proteins from the mice lung tissues or cells. The BCA protein concentration determination kit (Beyotime) was used to determine the protein concentration. The proteins in different samples were separated by SDS-PAGE, and transferred to the polyvinylidene fluoride (PVDF) membrane. The membrane was sealed with 5% BSA at RT for 2 h. It was then incubated overnight at 4 ℃ with different primary antibodies (1:500–1,000). After that, once the unbound primary antibodies were removed from the membrane, the corresponding horseradish peroxidase (HRP)-conjugated Abs (1:5,000–10,000) was incubated at RT for 1 h. Finally, the images were processed using the enhanced chemiluminescence (ECL) detection kit (Beyotime). The data was quantified by Image J software.
Edema assay
Upon removal from the mice, the lung samples were promptly weighed and subjected to drying at 100 ℃ for 24 h. Subsequently, it was weighed again. The degree of lung edema is determined by calculating the ratio of dry weight to wet weight.
Apoptosis assay
Annexin V-fluorescein 5-isothiocyanate (FITC)/propidium iodide (PI) Apoptosis Detection Kit (Vazyme, China) was used to detect cell apoptosis. Took 1×106 cells, rinsed with PBS, added 3 mL of 70% ethanol slowly to the cells, and allowed them to fix at 4 ℃ overnight. Then, the cells were mixed with Annexin V-FITC and PI, gently stirred, and kept at RT for 15 min. Employed the FACScalibur flow cytometer (BD Biosciences, USA) to examine cell distribution and ultimately utilized the FlowJo software for analysis.
Wound healing assay
About 1×105 cells were seeded into a 6-well plate and cultured to 90–100% confluence after 24 h. A pipette tip was used to create wounds in each well of the 6-well plate. Subsequently, the migration of cells was visualized under a microscope and photographed at different time points (0 and 24 h).
Statistical analysis
GraphPad Prism 9.0 software was used to conduct all analyses. The experiments were presented as mean ± standard error of the mean (SEM, n≥3). The comparison between the two groups was performed using a non-parametric t-test. To assess multiple groups and determine statistical differences, one-way analysis of variance (ANOVA) and Tukey’s test were utilized. A P value below 0.05 was deemed significant statistically.
Results
SV enhanced cell proliferation and suppressed apoptosis in LPS-treated cells by upregulating miR-744-5p
In our previous research using miRNA microarray technology to screen differentially expressed miRNAs in ALI after SV treatment, we identified miR-744-5p as a related gene. To explore the relationship between miR-744-5p and ALI, we initially developed an in vitro cell model induced by LPS. The results revealed a significant decrease in miR-744-5p levels in LPS-prompted HPMECs, indicating a potential correlation with ALI (Figure 1A). Cell viability was greatly reduced by LPS, but this effect was alleviated by varying concentrations of SV. In particular, 2% SV had a notable restorative effect on cell viability (Figure 1B). The occurrence of cell apoptosis is essential in the pathology of ALI. To assess the impact of SV on LPS-induced cell apoptosis, we utilized flow cytometry to measure the ratios of early and late apoptosis in ALI cells after administration of SV. As predicted, the proportion of apoptotic cells notably rose with LPS treatment compared to the control group. However, the introduction of 2% SV significantly hindered the occurrence of LPS-induced cell apoptosis (Figure 1C). In order to investigate the impact of miR-744-5p on the therapeutic function of SV in LPS-induced cells, we utilized the miR-744-5p inhibitor. The research results show that SV significantly upregulated the expression of miR-744-5p in cells induced by LPS and compared with the LPS + SV group. The expression of miR-744-5p in the LPS + SV + miR-744-5p inhibitor group has significantly decreased, indicating that the level of intracellular miR-744-5p is effectively inhibited by the inhibitor (Figure 1D). The CCK-8 outcomes demonstrated that LPS resulted in a significant hindrance in cell proliferation, yet this was relieved by SV. Nonetheless, the utilization of miR-744-5p inhibitor reversed the therapeutic impact of SV (Figure 1E). Concurrently, the miR-744-5p inhibitor effectively countered the anti-apoptotic impact of SV on LPS-induced cells, resulting in a notable rise in apoptotic cells induced by LPS (Figure 1F). The aforementioned results demonstrated that the SV increased cell activity and decreased cell apoptosis in cells treated with LPS, whereas this effect was cancelled by the miR-744-5p inhibitor.
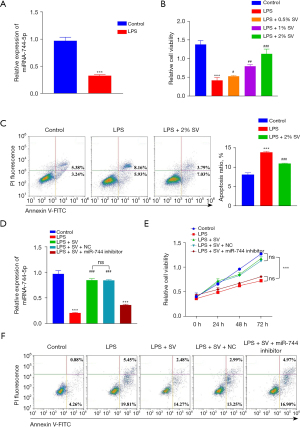
Inhibited miR-744-5p aggravated sepsis-induced ALI in mice
We investigated whether the aggravation of the ALI process would occur by inhibiting the expression of miR-744-5p in vivo after confirming the decrease in miR-744-5p expression in LPS-induced cells. The CLP model is widely recognized as the most commonly employed model for sepsis due to its close resemblance to human sepsis. It is advantageous because it involves the introduction of the animal’s own mixed microbial flora into the peritoneum through binding with inactivated tissue (24). The mice in the CLP group exhibited noticeable inflammatory alterations in their lungs, including edema, necrosis, and infiltration by neutrophils in comparison to the control group (Figure 2A). Afterward, the histopathological changes were assessed and the outcomes demonstrated that CLP substantially caused damage to the tissue structure, confirming the successful creation of the model (Figure 2B). To investigate the potential contribution of miR-744-5p in the development of ALI in CLP mice, we employed qRT-PCR to analyze the levels of miR-744-5p in lung tissues across different groups. Our findings exhibited a noteworthy decrease in miR-744-5p expression in the CLP group when compared to the sham group (Figure 2C). This aligned with the findings from our previous in vitro experiments. The miRNA antagomir, which has undergone special chemical modifications, is an antagonist of miRNA. It can effectively compete with mature miRNA within the body and hinder its pairing with the mRNA of target genes, ultimately inhibiting the function of miRNA (25). In in vivo experiments, it demonstrates greater stability and effectiveness in inhibiting miRNA compared to regular miRNA inhibitors (26). In CLP mice, the levels of miR-744-5p were significantly inhibited by miR-744-5p antagomir when compared to the control group (Figure 2C). Moreover, according to the H&E and Masson staining results, it can be concluded that the severity of ALI in CLP mice were notably worsened by the utilization of miR-744-5p antagomir and it was also supported by the results obtained from the Lung Injury score and lung wet-dry (W/D) ratio (Figure 2D). To further assess the anti-inflammatory impact of miR-744-5p, the levels of pro-inflammatory cytokines in BALF were examined. The results revealed a significant increase in the levels of IL-1β and TNF-α in the BALF of the CLP group, compared to the control group. However, treatment with miR-744-5p antagomir effectively suppressed the levels of pro-inflammatory cytokines in CLP mice (Figure 2E). The levels of proinflammatory cytokines in lung tissues of mice in each group were detected by qRT-PCR. The results showed that mRNA levels of Il1β, Tnfα, Il6 and Ccl2 in lung tissue of CLP group were significantly increased compared with the control group. However, treatment with miR-744-5p antagonists can effectively inhibit the expression level of proinflammatory cytokines in CLP mice (Figure S1). It has been previously observed that TGF-β1 plays a crucial role in immune and inflammatory responses to microbial infections and is associated with the development of ALI (27,28). Accordingly, TGF-β1 was picked for further analysis. As shown in the results of the western blot (Figure 2F) and IHC (Figure 2G) analysis, the CLP group showed a significant increase in TGF-β1 and the ratio of p-Smad3/t-Smad3 compared to the sham group, and this outcome was worsened with the administration of antagomir-744-5p.
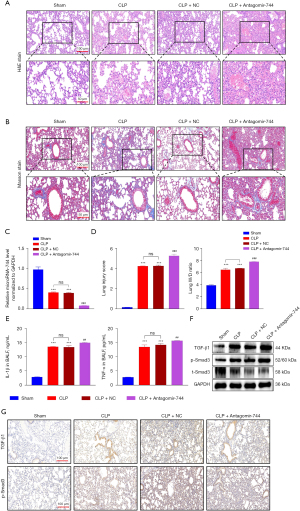
Down-regulation of miR-744-5p exacerbated cellular inflammation injury in LPS-induced ALI cells
To analyze the effectiveness of miR-744-5p on ALI cells induced by LPS in vitro, we used a miR-744-5p inhibitor for further experiments. As shown in Figure 3A, the level of miR-744-5p was significantly reduced by the miR-744-5p inhibitor. During the testing of the cell culture medium, it was observed that the presence of LPS considerably increases the production of pro-inflammatory cytokines like IL-1β (Figure 3B) and IL-6 (Figure 3C), and this effect is further intensified by the miR-744-5p inhibitor. According to EDU results, cell proliferation was significantly suppressed by LPS, and the presence of the miR-744-5p inhibitor enhanced this effect (Figure 3D). In addition, during the wound healing experiment, it was discovered that the cell migration ability was significantly impaired due to LPS treatment, while the application of the miR-744-5p inhibitor appeared to intensify this effect (Figure 3E). The detection of cell apoptosis indicated that LPS promoted cell apoptosis, and this impact was considerably intensified following the restraint of miR-744-5p (Figure 3F). Afterwards, we collected total proteins from cells belonging to various groups and conducted protein immunoblotting to examine the impact of miR-744-5p on TGF-β1 and phosphorylated Smad3. As anticipated, the suppression of miR-744-5p resulted in a more substantial increase in the expression of TGF-β1 and phosphorylated Smad3 in cells induced by LPS (Figure 3G). The results mentioned above highlight that suppressing miR-744-5p greatly increased the inflammation level of HPMECs induced by LPS. It also hampered cell proliferation and migration, induced cell apoptosis, and intensified the activation of the TGF-β1/Smad3 signaling pathway.
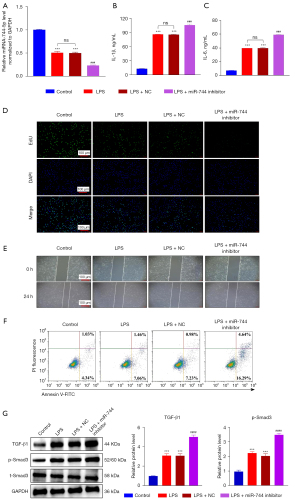
MiR-744-5p specifically targeted TGF-β1
As previously stated, we used miRDB (29) and TargetScan8.0 (30) to project the potential target of miR-744-5p. According to the analysis, TGF-β1 was regarded as the most suitable choice (Figure 4A). Up-regulating miR-744-5p can suppress the mRNA levels of TGFB1, conversely, adding the inhibitor of miR-744-5p can significantly promote the mRNA levels of TGFB1 (Figure 4B). To verify whether TGF-β1 is directly targeted by miR-744-5p, we created a dual luciferase reporter system that included the TGF-β1 3'-untranslated region (3'-UTR) and the presumed binding site for miR-744-5p (Figure 4C). According to the results of luciferase reporter gene assay (Figure 4D), the luciferase activity decreased significantly when both the TGF-β1 3'-UTR and miR-744-5p mimics were co-transfected into WT cells. On the other hand, the luciferase activity increased significantly when the luciferase reporter gene and miR-744-5p inhibitor were co-transfected into WT cells. However, these effects were reversed when mutations occurred in the TGF-β1 sequence.
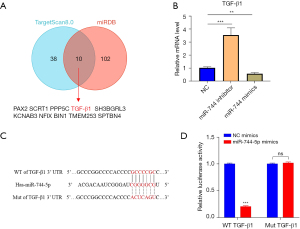
SV improved ALI in CLP mice by upregulating miR-744-5p to suppress the TGF-β1/Smad3 signaling pathway
To determine whether the increased expression of miR-744-5p induced by SV has a protective effect on ALI in CLP mice, we carried out SV treatment on the CLP mouse model and suppressed the level of miR-744-5p by using miR-744-5p antagomir. Subsequently, we evaluated the extent of lung tissue damage in mice by conducting H&E and Masson staining, and also examined the levels of TGF-β1 and phosphorylated Smad3 expression through IHC and western blot analysis. Lung tissue damage was assessed by employing H&E (Figure 5A) and Masson’s staining (Figure 5B). In comparison to the sham group, CLP caused a notable pulmonary histological damage in mice, which was observed as the breakdown of alveolar structure, significant build-up of fibrotic exudation in alveolar cavities, extensive infiltration of inflammatory cells surrounding blood vessels, noticeable edema and thickening of alveolar septa, and increased presence of collagen fibers in the overall tissue region. Despite SV alleviating lung injury in CLP mice, the administration of miR-744-5p antagomir worsened the extent of this damage. In addition, the lung injury was not amplified or treated when using SV and miR-744-5p antagomir at the same time in CLP mice. In the following study conducted on the TGF-β1/Smad3 signaling pathway, we observed that this phenomenon remains present. The expression levels of TGF-β1 and phosphorylated Smad3 in lung tissues were found to be significantly increased in the CLP group compared to the sham group, as indicated by the IHC (Figure 5C) and western blot (Figure 5D) experimental results. Furthermore, the promotion of this effect was significantly enhanced by miR-744-5p antagomir treatment, while the expression of TGF-β1 and phosphorylated Smad3 in the lung tissues of septic mice was significantly reduced with SV treatment. In the CLP + SV + antagomir-744-5p group, the expression levels of TGF-β1 and phosphorylated Smad3 were not significantly different from those in the CLP group (P>0.05). Using qRT-PCR, miR-744-5p levels were initially determined in lung tissue samples. The outcomes revealed that the presence of SV significantly elevated miR-744-5p levels. Conversely, there was no notable disparity in miR-744-5p levels between CLP mice treated with both SV and miR-744-5p antagomir and CLP mice alone (P>0.05) (Figure 5E). This observation implied that the contrasting influences of SV and miR-744-5p antagomir on miR-744-5p might nullify each other. The results stated above implied that the TGF-β1/Smad3 signaling pathway was suppressed by SV upregulating miR-744-5p, resulting in improved ALI in CLP mice.
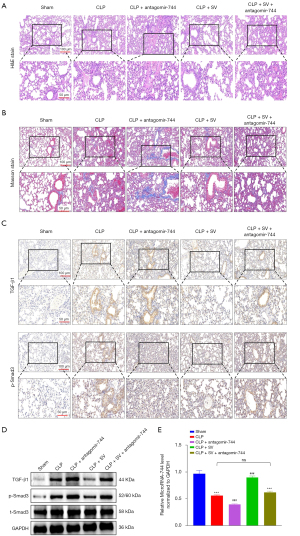
SV inhibited the TGF-β1/Smad3 signaling pathway by upregulating miR-744-5p
Having confirmed the alleviating effect of SV on lung injury in CLP-induced ALI mice through the upregulation of miR-744-5p, we led to proceeded to examine if SV can also alleviate ALI in vitro in HPMECs by enhancing the expression of miR-744-5p. The results are presented as follows. In LPS-treated HPMECs, the expression levels of miR-744-5p were significantly decreased compared to the control group, as indicated by the qRT-PCR results. However, this outcome was reversed in the LPS + SV group. Additionally, the group treated with both SV and miR-744-5p inhibitor did not show any significant alteration in miR-744-5p levels, as compared to the LPS group (P>0.05) (Figure 6A). The phenomenon was also observed in the detection of pro-inflammatory factors in cell culture medium. The expression of pro-inflammatory factors was significantly increased by LPS, but was restored to normal levels with SV treatment. Additionally, there were no significant changes in the expression of pro-inflammatory factors when SV and miR-744-5p inhibitor were used together compared to the LPS group (P>0.05). It was important to note that using miR-744-5p inhibitor alone in LPS-induced ALI cells led to a significant increase in the expression of pro-inflammatory factors, suggesting that miR-744-5p has an inhibitory effect on inflammation response in ALI (Figure 6B). Afterward, we examined the impact of SV on the proliferation, migration, and apoptosis of cells with LPS-induced ALI, and the findings are outlined below. Cell proliferation was significantly hindered by LPS, whereas miR-744-5p was upregulated by SV, enhancing cell proliferation (Figure 6C). Moreover, migration ability in ALI cells was suppressed by LPS, but restored by SV (Figure 6D). This suggests that SV has a positive impact on improving the lung microenvironment of ALI and can potentially prevent its progression into more severe conditions like ARDS and pulmonary fibrosis (PF). In comparison to the control group, cells in the LPS group exhibited significant apoptosis, and the presence of SV partially alleviated this occurrence. Furthermore, among all groups, the LPS + miR-744-5p inhibitor group displayed the highest ratio of apoptotic cells. However, in the LPS + SV + miR-744-5p inhibitor group, the effects of SV and the miR-744-5p inhibitor on cell apoptosis negated each other (Figure 6E). The detection of the TGF-β1/Smad3 signaling pathway in LPS-induced ALI cells revealed a significant enhancement of the pathway due to SV, which was found to be considerably inhibited by the miR-744-5p inhibitor (Figure 6F). The results mentioned above suggest that SV effectively reduces LPS-induced ALI by increasing miR-744-5p levels and improving the TGF-β1/Smad3 signaling pathway, including suppressing the expression of pro-inflammatory factors, restoring cell proliferation and migration, and inhibiting cell apoptosis.
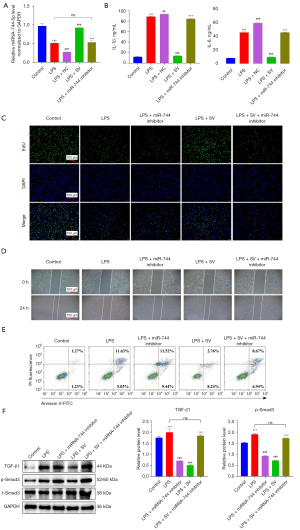
Discussion
According to clinical studies, the lung is highly vulnerable to injury in the progression of sepsis. In the initial phases of sepsis, patients may experience ALI and ARDS (31-33). The occurrence of lung injury is closely tied to the prognosis of sepsis and is also the primary factor leading to death in septic patients. The inflammatory reaction triggered by ALI caused by sepsis is exceptionally intense, resulting in pulmonary interstitial edema and alveolar collapse, leading to irreversible harm to the alveoli. As a consequence, pulmonary compliance diminishes and refractory hypoxemia occurs in patients, ultimately resulting in the demise of the organism (1,34,35). At present, there are no specific drugs and effective treatment measures to treat this disease. Therefore, the treatment strategy for sepsis-related ALI currently focuses on supportive treatment and symptom management, including respiratory support, infection control, fluid management and hemodynamic support (36). These treatments are aimed at reducing inflammatory response, maintaining organ function and correcting metabolic disorders, thereby improving survival (37). In addition to the supportive treatments mentioned above, some new treatment strategies are under study, such as targeted therapy for specific inflammatory pathways, immunomodulatory therapy and antioxidant therapy (38). In the present study, SV is a potent inhibitor of neutrophil elastase, specifically designed to inhibit its activity (39). Researches have demonstrated that SV effectively decreases the release of inflammatory mediators, inhibits cell apoptosis, and thereby reduces the extent of tissue damage (40). In our study, SV successfully alleviated cell damage caused by LPS, as it greatly decreased the expression of inflammatory factors, improved lung tissue function, restored the capacity of HPMECs to proliferate and migrate, and prevented cell apoptosis. Additionally, the therapeutic efficacy of SV was confirmed in mice with ALI induced by CLP. Besides, the fact that the presence of miR-744-5p antagomir and miR-744-5p inhibitor reverses the inhibitory effects of SV on mice ALI induced by CLP and HPMECs ALI induced by LPS is intriguing, indicating that miR-744-5p might have a role in SV’s mechanism of action in ALI.
The role of miRNA as a biomarker for ALI and its potential as therapeutic targets for this condition has been highlighted in recent research. For instance, Ju et al. discovered that miR-27a alleviates LPS-induced ALI in mice by inhibiting inflammation and apoptosis through the regulation of TLR4 (41). The NF-κB signaling pathway is inhibited while Nrf2 and HO-1 expression is upregulated by miR-27b in order to alleviate LPA-induced ALI in mice (25). Additionally, Jansing et al. discovered that injury and remodeling in ALI were significantly diminished when miR-21 was knocked out (42). In our study, through the use of miRNA chips and bioinformatics analysis in SV-treated patients, we found a strong correlation between miR-744-5p and ALI. Subsequently, we utilized miRDB and TargetScan8.0 to predict potential targets of miR-744-5p, and discovered a significant association with TGF-β1, which was later confirmed through dual-luciferase reporter gene assays. And TGF-β1 has been shown to be significantly associated with many inflammatory diseases (43). Further experiments revealed that the therapeutic effects of SV on CLP or LPS-induced ALI in mice and HPMECs were significantly hindered by the miR-744-5p antagomir and miR-744-5p inhibitor. In addition, IHC and western blot experiments confirmed that SV increased miR-744-5p expression, resulting in the reduction of TGF-β1 and phosphorylated Smad3 expression. These findings strongly suggest that SV improves sepsis-induced ALI by increasing miR-744-5p levels to inhibit the TGF-β1/Smad3 signaling pathway.
Although these results are meaningful, our study does have some limitations. For instance, further investigation is required to examine the role of SV in regulating TGF-β receptors and elucidate the reasons behind its upregulation of miR-744-5p. Additionally, more research on different manifestations in the CLP-induced ALI animal model, such as endoplasmic reticulum stress, myeloperoxidase (MPO) activity, and neutrophil accumulation, is necessary to provide further evidence for the clinical use of SV. And in order to enhance clinical application of SV, it is imperative for future studies to integrate clinical investigation to intensively examine the mechanism of action of SV, identify the most effective dosage patterns, and explore its concurrent use with other treatment modalities.
Conclusions
Our study suggests that SV improves sepsis-induced ALI by up-regulating miR-744-5p to inhibit the TGF-β1/Smad3 signaling pathway. Furthermore, our findings demonstrate that SV effectively treats lung tissue damage by reducing collagen fiber deposition, suppressing cell apoptosis, and alleviating inflammatory reactions.
Acknowledgments
Funding: The study was supported by
Footnote
Reporting Checklist: The authors have completed the ARRIVE reporting checklist. Available at https://jtd.amegroups.com/article/view/10.21037/jtd-24-65/rc
Data Sharing Statement: Available at https://jtd.amegroups.com/article/view/10.21037/jtd-24-65/dss
Peer Review File: Available at https://jtd.amegroups.com/article/view/10.21037/jtd-24-65/prf
Conflicts of Interest: All authors have completed the ICMJE uniform disclosure form (available at https://jtd.amegroups.com/article/view/10.21037/jtd-24-65/coif). The authors have no conflicts of interest to declare.
Ethical Statement: The authors are accountable for all aspects of the work in ensuring that questions related to the accuracy or integrity of any part of the work are appropriately investigated and resolved. Experiments were performed under a project license (No. HYLL-2021-113) granted by institutional ethics board of The First Affiliated Hospital of Hainan Medical University, in compliance with The First Affiliated Hospital of Hainan Medical University institutional guidelines for the care and use of animals.
Open Access Statement: This is an Open Access article distributed in accordance with the Creative Commons Attribution-NonCommercial-NoDerivs 4.0 International License (CC BY-NC-ND 4.0), which permits the non-commercial replication and distribution of the article with the strict proviso that no changes or edits are made and the original work is properly cited (including links to both the formal publication through the relevant DOI and the license). See: https://creativecommons.org/licenses/by-nc-nd/4.0/.
References
- Park I, Kim M, Choe K, et al. Neutrophils disturb pulmonary microcirculation in sepsis-induced acute lung injury. Eur Respir J 2019;53:1800786. [Crossref] [PubMed]
- Weiss SL, Fitzgerald JC, Pappachan J, et al. Global epidemiology of pediatric severe sepsis: the sepsis prevalence, outcomes, and therapies study. Am J Respir Crit Care Med 2015;191:1147-57. [Crossref] [PubMed]
- Chen R, Cao C, Liu H, et al. Macrophage Sprouty4 deficiency diminishes sepsis-induced acute lung injury in mice. Redox Biol 2022;58:102513. [Crossref] [PubMed]
- Mohsin M, Tabassum G, Ahmad S, et al. The role of mitophagy in pulmonary sepsis. Mitochondrion 2021;59:63-75. [Crossref] [PubMed]
- Yang T, Zhang J, Sun L, et al. Combined effects of a neutrophil elastase inhibitor (sivelestat sodium) and a free radical scavenger (edaravone) on lipopolysaccharide-induced acute lung injury in rats. Inflamm Res 2012;61:563-9. [Crossref] [PubMed]
- Sadowitz B, Roy S, Gatto LA, et al. Lung injury induced by sepsis: lessons learned from large animal models and future directions for treatment. Expert Rev Anti Infect Ther 2011;9:1169-78. [Crossref] [PubMed]
- Sahebnasagh A, Saghafi F, Safdari M, et al. Neutrophil elastase inhibitor (sivelestat) may be a promising therapeutic option for management of acute lung injury/acute respiratory distress syndrome or disseminated intravascular coagulation in COVID-19. J Clin Pharm Ther 2020;45:1515-9. [Crossref] [PubMed]
- Derynck R, Budi EH. Specificity, versatility, and control of TGF-β family signaling. Sci Signal 2019;12:eaav5183. [Crossref] [PubMed]
- Travis MA, Sheppard D. TGF-β activation and function in immunity. Annu Rev Immunol 2014;32:51-82. [Crossref] [PubMed]
- Hata A, Chen YG. TGF-β Signaling from Receptors to Smads. Cold Spring Harb Perspect Biol 2016;8:a022061. [Crossref] [PubMed]
- Hu Y, He J, He L, et al. Expression and function of Smad7 in autoimmune and inflammatory diseases. J Mol Med (Berl) 2021;99:1209-20. [Crossref] [PubMed]
- Chung AC, Yu X, Lan HY. MicroRNA and nephropathy: emerging concepts. Int J Nephrol Renovasc Dis 2013;6:169-79. [Crossref] [PubMed]
- Zhang H, Zeng J, Li J, et al. Sivelestat sodium attenuates acute lung injury by inhibiting JNK/NF-κB and activating Nrf2/HO-1 signaling pathways. Biomol Biomed 2023;23:457-70. [Crossref] [PubMed]
- Zhang R, Gao X, Hu F, et al. Myocardial protective effect of sivelestat sodium in rat models with sepsis-induced myocarditis. J Thorac Dis 2022;14:4003-11. [Crossref] [PubMed]
- Yoshikawa S, Tsushima K, Koizumi T, et al. Effects of a synthetic protease inhibitor (gabexate mesilate) and a neutrophil elastase inhibitor (sivelestat sodium) on acid-induced lung injury in rats. Eur J Pharmacol 2010;641:220-5. [Crossref] [PubMed]
- Wang H, Tang AM, Liu D, et al. Renoprotective activity of sivelestat in severe acute pancreatitis in rats. Exp Ther Med 2013;6:29-32. [Crossref] [PubMed]
- Weng J, Liu D, Shi B, et al. Sivelestat sodium alleviated lipopolysaccharide-induced acute lung injury by improving endoplasmic reticulum stress. Gene 2023;884:147702. [Crossref] [PubMed]
- Wang Y, Wang M, Zhang H, et al. Sivelestat improves clinical outcomes and decreases ventilator-associated lung injury in children with acute respiratory distress syndrome: a retrospective cohort study. Transl Pediatr 2022;11:1671-81. [Crossref] [PubMed]
- Lee RC, Feinbaum RL, Ambros V. The C. elegans heterochronic gene lin-4 encodes small RNAs with antisense complementarity to lin-14. Cell 1993;75:843-54. [Crossref] [PubMed]
- Fu X, Zeng L, Liu Z, et al. MicroRNA-206 regulates the secretion of inflammatory cytokines and MMP9 expression by targeting TIMP3 in Mycobacterium tuberculosis-infected THP-1 human macrophages. Biochem Biophys Res Commun 2016;477:167-73. [Crossref] [PubMed]
- Wei X, Yi X, Lv H, et al. MicroRNA-377-3p released by mesenchymal stem cell exosomes ameliorates lipopolysaccharide-induced acute lung injury by targeting RPTOR to induce autophagy. Cell Death Dis 2020;11:657. [Crossref] [PubMed]
- Jiang J, Huang K, Xu S, et al. Targeting NOX4 alleviates sepsis-induced acute lung injury via attenuation of redox-sensitive activation of CaMKII/ERK1/2/MLCK and endothelial cell barrier dysfunction. Redox Biol 2020;36:101638. [Crossref] [PubMed]
- Sakai H, Sagara A, Matsumoto K, et al. Neutrophil recruitment is critical for 5-fluorouracil-induced diarrhea and the decrease in aquaporins in the colon. Pharmacol Res 2014;87:71-9. [Crossref] [PubMed]
- Chen G, Hou Y, Li X, et al. Sepsis-induced acute lung injury in young rats is relieved by calycosin through inactivating the HMGB1/MyD88/NF-κB pathway and NLRP3 inflammasome. Int Immunopharmacol 2021;96:107623. [Crossref] [PubMed]
- Huang Y, Huang L, Zhu G, et al. Downregulated microRNA-27b attenuates lipopolysaccharide-induced acute lung injury via activation of NF-E2-related factor 2 and inhibition of nuclear factor κB signaling pathway. J Cell Physiol 2019;234:6023-32. [Crossref] [PubMed]
- Baigude H, Rana TM. Strategies to antagonize miRNA functions in vitro and in vivo. Nanomedicine (Lond) 2014;9:2545-55. [Crossref] [PubMed]
- Bosmann M, Ward PA. Protein-based therapies for acute lung injury: targeting neutrophil extracellular traps. Expert Opin Ther Targets 2014;18:703-14. [Crossref] [PubMed]
- Zhang YG, Singhal M, Lin Z, et al. Infection with enteric pathogens Salmonella typhimurium and Citrobacter rodentium modulate TGF-beta/Smad signaling pathways in the intestine. Gut Microbes 2018;9:326-37. [Crossref] [PubMed]
- Chen Y, Wang X. miRDB: an online database for prediction of functional microRNA targets. Nucleic Acids Res 2020;48:D127-31. [Crossref] [PubMed]
- McGeary SE, Lin KS, Shi CY, et al. The biochemical basis of microRNA targeting efficacy. Science 2019;366:eaav1741.
- O'Brien JM Jr, Ali NA, Aberegg SK, et al. Sepsis. Am J Med 2007;120:1012-22. [Crossref] [PubMed]
- Li W, Li D, Chen Y, et al. Classic Signaling Pathways in Alveolar Injury and Repair Involved in Sepsis-Induced ALI/ARDS: New Research Progress and Prospect. Dis Markers 2022;2022:6362344. [Crossref] [PubMed]
- Liu C, Zhou Y, Tu Q, et al. Alpha-linolenic acid pretreatment alleviates NETs-induced alveolar macrophage pyroptosis by inhibiting pyrin inflammasome activation in a mouse model of sepsis-induced ALI/ARDS. Front Immunol 2023;14:1146612. [Crossref] [PubMed]
- Jiang K, Yang J, Guo S, et al. Peripheral Circulating Exosome-Mediated Delivery of miR-155 as a Novel Mechanism for Acute Lung Inflammation. Mol Ther 2019;27:1758-71. [Crossref] [PubMed]
- Jiao Y, Zhang T, Zhang C, et al. Exosomal miR-30d-5p of neutrophils induces M1 macrophage polarization and primes macrophage pyroptosis in sepsis-related acute lung injury. Crit Care 2021;25:356. [Crossref] [PubMed]
- Mowery NT, Terzian WTH, Nelson AC. Acute lung injury. Curr Probl Surg 2020;57:100777. [Crossref] [PubMed]
- Li J, Deng SH, Li J, et al. Obacunone alleviates ferroptosis during lipopolysaccharide-induced acute lung injury by upregulating Nrf2-dependent antioxidant responses. Cell Mol Biol Lett 2022;27:29. [Crossref] [PubMed]
- Lu Q, Huang S, Meng X, et al. Mechanism of Phosgene-Induced Acute Lung Injury and Treatment Strategy. Int J Mol Sci 2021;22:10933. [Crossref] [PubMed]
- Hagiwara S, Iwasaka H, Hidaka S, Hasegawa A, Noguchi T. Neutrophil elastase inhibitor (sivelestat) reduces the levels of inflammatory mediators by inhibiting NF-kB. Inflamm Res 2009;58:198-203. [Crossref] [PubMed]
- Suda K, Takeuchi H, Hagiwara T, et al. Neutrophil elastase inhibitor improves survival of rats with clinically relevant sepsis. Shock 2010;33:526-31. [Crossref] [PubMed]
- Ju M, Liu B, He H, et al. MicroRNA-27a alleviates LPS-induced acute lung injury in mice via inhibiting inflammation and apoptosis through modulating TLR4/MyD88/NF-κB pathway. Cell Cycle 2018;17:2001-18. [Crossref] [PubMed]
- Jansing JC, Fiedler J, Pich A, et al. miR-21-KO Alleviates Alveolar Structural Remodeling and Inflammatory Signaling in Acute Lung Injury. Int J Mol Sci 2020;21:822. [Crossref] [PubMed]
- Aghasafari P, George U, Pidaparti R. A review of inflammatory mechanism in airway diseases. Inflamm Res 2019;68:59-74. [Crossref] [PubMed]