Reverse triggering in patients with acute brain injury undergoing controlled ventilation
Highlight box
Key findings
• Deep sedation, lower airway delta pressure, and close alignment of ventilator and patient respiratory rates are associated with reverse triggering (RT) in patients with acute brain injury. Pressure of occlusion at 0.1 seconds (P0.1) serves as a reliable predictor for the occurrence of breath stacking (BD).
What is known and what is new?
• RT is common in deeply sedated patients undergoing controlled ventilation.
• Lower airway delta pressure and minimal discrepancies between the set respiratory rate and the actual respiratory rate were associated with RT in patients with acute brain injury. Additionally, P0.1 had good predictive ability for BD.
What is the implication, and what should change now?
• RT is common among patients with acute brain injury. Patients with higher respiratory drives may be more susceptible to BD.
Introduction
Background
Reverse triggering (RT) is a form of ventilatory asynchrony in which the patient’s inspiratory effort is triggered by ventilator insufflation in a repetitive pattern (1). Notably, RT has been observed in deeply sedated patients with acute respiratory distress syndrome (ARDS), anesthetized patients, and even healthy individuals (1-3). However, the pathophysiological mechanisms of RT are not well-understood. The prevailing hypothesis suggests that RT involves the entrainment of the patient’s respiratory rhythm to periodic ventilator insufflation. This phenomenon is thought to engage mechanisms such as the Hering-Breuer reflex mediated by vagal afferents, intercostal-to-phrenic reflexes, and both cortical and subcortical influences (2,4). Furthermore, occurrences of RT in brain-dead patients implies a potential role for spinal reflexes (5). Recent research proposes five distinct phenotypes of RT, classified by their timing in the respiratory cycle and the presence or absence of breath stacking (BD) (6). When inspiratory effort occurs during the inspiratory phase, it may inadvertently increase tidal volume (VT) and transpulmonary pressure, potentially exacerbating lung injury (7). Conversely, inspiratory effort occurring during the expiratory phase might prevent lung collapse and preserve functional residual capacity but could lead to eccentric contraction of the diaphragm, resulting in muscle injury (8). The ultimate impact of RT is contingent upon its timing, the magnitude of inspiratory effort, the prevailing lung conditions, and the duration of RT. The likelihood of RT may be influenced by factors such as ventilator settings, the use of opioids and sedatives, and the patient’s overall condition (7,9,10).
Rationale and knowledge gap
Patients with acute brain injury are particularly susceptible to asynchrony due to potential injuries to the respiratory center (11). Despite this, there remains a significant gap in knowledge regarding RT in this patient group.
Objective
This retrospective study aims to elucidate the incidence and contributing factors of RT among patients with acute brain injury undergoing controlled ventilation, utilizing esophageal pressure (Pes) monitoring to identify RT across different respiratory phases. This manuscript was written in accordance with the STROBE reporting checklist (available at https://jtd.amegroups.com/article/view/10.21037/jtd-24-694/rc).
Methods
Study population
The study was conducted in accordance with the Declaration of Helsinki (as revised in 2013). This study conducted a secondary analysis of data derived from a prospective observational study on patient-ventilator asynchrony in patients with acute brain injury at the intensive care unit (ICU) of Beijing Tiantan Hospital, affiliated with Capital Medical University (ClinicalTrials.gov identifier: NCT03212482). The cohort comprised adult patients diagnosed with acute brain injury who were receiving controlled mechanical ventilation. Exclusion criteria included: (I) mechanical ventilation duration of less than 24 hours; (II) presence of epilepsy; (III) agitation; (IV) contraindications to esophageal balloon catheter insertion; (V) evidence of an active air leak from the lung, including conditions such as bronchopleural fistula, pneumothorax, pneumomediastinum, or the presence of a chest tube; and (VI) absence of spontaneous breathing.
The use of anonymized data was approved by the Institutional Review Board of Beijing Tiantan Hospital, Capital Medical University (approval No. KY 2017-028-02) and the informed consent was waived due to the retrospective nature of this study.
Data collection
Demographic and clinical data were collected at ICU admission and included the classification of acute brain injury, Glasgow Coma Scale (GCS) score, comorbidities, and the Acute Physiology and Chronic Health Evaluation (APACHE) II score. Additional data included the ratio of partial pressure of oxygen to fraction of inspired oxygen (PaO2/FiO2 ratio), and the duration of mechanical ventilation.
Patients were ventilated using an AVEA ventilator (CareFusion Co., Ltd., Illinois, USA) equipped with Pes monitoring (SmartCath-G catheter: LOT 7003300; CareFusion Co., Ltd.). The optimal position of the esophageal balloon was verified using Baydur’s occlusion test for patients with spontaneous breathing (12) or the positive pressure occlusion test for those without spontaneous breathing (13). Airway pressure (Paw), flow, and Pes waveforms were collected through a dedicated acquisition system (VOXP Research Data Collector 3.2; Applied Biosignals GmbH, Weener, Germany) at a frequency of 100 Hz. Recordings were taken four times daily, each lasting 15 minutes, and were compiled into waveform datasets. Data collection spanned 3 days or until the patient was separated from the ventilator. End-expiratory occlusion tests were performed at the end of each recording session. In patients with spontaneous breathing, the pressure of occlusion at 0.1 seconds (P0.1) was measured and averaged across 5 occlusions to assess respiratory drive (14).
The ventilatory modes and settings, respiratory parameters [peak inspiratory pressure (PIP), positive end-expiratory pressure (PEEP), and Pes], and the usage of opioids or sedatives were documented for each recording session. The Sedation-Agitation Scale (SAS) score was evaluated following waveform data collection. Arterial blood gas measurements were recorded at least once daily. The investigators were not involved in the clinical management of the patients, and the ICU physicians were responsible for determining ventilatory settings, as well as the administration of opioids or sedatives, which remained constant during the recording periods.
Definition of RT
RT was defined as an inspiratory effort, indicated by a negative fluctuation of Pes, occurring after a mandatory insufflation in a repetitive and stable manner (1,15). The entrainment ratio for RT was determined by the count of RT breaths for each ventilator breath. A 1:1 ratio required presence in at least three or more consecutive cycles, and for a 1:2 ratio or other ratios, presence in at least six cycles was necessary (16). RT phenotypes were classified based on the respiratory phase at which the inspiratory effort commenced and where the maximum inspiratory effort occurred. Classes included “Early RT with early relaxation”, “Early RT with delayed relaxation”, “Mid-cycle RT”, “Late RT”, and “RT with breath staking” (6). To differentiate from ineffective efforts during expiration, Late RT was defined as the contraction of the respiratory muscles occurred during the early expiration, in which the maximal Pes deflection occurred less than 1.5 s after the machine insufflation beginning (17). The incidence of RT was calculated as the ratio of the number of RT breaths to the total number of breaths (9,17). The level of inspiratory effort originated from an RT was assessed by the change in Pes (ΔPes) (18). For each RT phenotype, the average Pes values for the first, last and middle events in each waveform dataset were utilized (19). Each breath was independently evaluated and diagnosed by two investigators (X.Y.L. and Y.M.Z.). Consensus was required for a diagnosis, and discrepancies were resolved through discussion among a review panel (H.L.L., Y.L.Y., and J.X.Z.).
Statistical analysis
Continuous variables were reported as medians and interquartile ranges (IQRs) and were compared between groups using the Mann-Whitney U test or Kruskal-Wallis analysis-of-variance test, with Bonferroni correction applied for multiple comparisons. Categorical variables were presented as proportions and analyzed using the Chi-squared test or Fisher’s exact test. Inter-rater agreement between investigators in classifying RT was assessed by Kappa statistics. The predictive capacity of P0.1 for BD was assessed by calculating the area under the receiver operating characteristic (ROC) curve. The correlation between P0.1 (patient triggered breath) and ΔPes (inspiratory effort originated from an RT) was assessed by Pearson correlation. Logistic regression was used to determine factors associated with BD. All statistical analyses were performed using SPSS version 21.0 (IBM Corp., Armonk, NY, USA). P values less than 0.05 were considered to be statistically significant.
Results
Demographic and clinical characteristics of patients
From June 2017 to July 2019, 264 patients diagnosed with acute brain injury and undergoing mechanical ventilation were screened. Of these patients, a total of 211 were excluded for the following reasons: age under 18 years (104 patients), epilepsy (25 patients), mechanical ventilation for less than 24 hours (32 patients), contraindications to esophageal balloon catheter insertion (3 patients), absence of spontaneous breathing (6 patients), and use of pressure support ventilation during the recording period (41 patients). The final cohort consisted of 53 patients, for whom 334 waveform datasets were generated from 92,013 ventilator breaths. The diagnoses for the cohort included stroke (41.5%), post craniotomy for brain tumor (30.2%), and traumatic brain injury (TBI; 28.3%). The median APACHE II score at ICU admission was 18 (IQR, 14–22). Among these patients, 12 (22.6%) were diagnosed with ARDS, presenting a median PaO2/FiO2 ratio of 171.3 (IQR, 107.1–222.7) mmHg. The characteristics of this cohort are presented in Table 1. Of the 334 waveform datasets analyzed, 166 (49.7%) were on pressure assist/control ventilation (PACV), 110 (32.9%) on pressure-preset synchronized intermittent mandatory ventilation (P-SIMV), and 58 (17.4%) on volume assist/control ventilation (VACV).
Table 1
Category | RT (n=16) | No-RT (n=37) | P |
---|---|---|---|
Age (years) | 50 [37–63] | 53 [39–64] | 0.70 |
Male | 12 (75.0) | 22 (59.5) | 0.28 |
APACHE II at ICU admission | 18 [13–25] | 18 [14–22] | 0.73 |
ARDS | 5 (31.3) | 7 (18.9) | 0.33 |
Type of brain injury | 0.69 | ||
Stroke | 6 (37.5) | 16 (43.2) | |
Post-craniotomy for brain tumors | 4 (25.0) | 12 (32.4) | |
TBI | 6 (37.5) | 9 (24.3) | |
Infratentorial lesions | 6 (37.5) | 17 (45.9) | 0.57 |
SOFA at inclusion | 7 [5–8] | 5 [4–7] | 0.06 |
GCS at inclusion | 9 [5–11] | 10 [5–11] | 0.81 |
PaO2/FiO2 ratio at inclusion (mmHg) | 178.0 [123.1–288.1] | 272.5 [185.8–396.3] | 0.009 |
PaCO2 at inclusion (mmHg) | 41.5 [34.0–47.3] | 37.0 [32.5–40.0] | 0.15 |
Comorbidity | |||
COPD | 0 | 2 (5.4) | >0.99 |
Hypertension | 7 (43.8) | 14 (37.8) | 0.69 |
Diabetes mellitus | 4 (25.0) | 6 (16.2) | 0.47 |
Coronary heart disease | 2 (12.5) | 4 (10.8) | >0.99 |
Outcomes | |||
ICU mortality | 4 (25.0) | 4 (10.8) | 0.22 |
In-hospital mortality | 5 (31.3) | 5 (13.5) | 0.15 |
Categorical variables are shown as number (percentage); continuous variables are shown as median [interquartile range]. RT, reverse triggering; APACHE II, Acute Physiology and Chronic Health Evaluation II; ICU, intensive care unit; ARDS, acute respiratory distress syndrome; TBI, traumatic brain injury; SOFA, Sequential Organ Failure Assessment; GCS, Glasgow Coma Scale; PaO2, partial pressure of oxygen; FiO2, fraction of inspired oxygen; PaCO2, partial pressure of carbon dioxide in artery; COPD, chronic obstructive pulmonary disease.
Incidence and distribution of RT
A total of 7,724 RT breaths (8.4%) were detected across 59 datasets (17.7%). The median incidence of RT in datasets that showed RT was 43.1% (IQR, 20.2–65.8%). Sixteen patients (30.2%) experienced at least one RT event. RT occurrence was more frequent in the stroke and TBI groups compared to the post-craniotomy for brain tumor group (10.3% vs. 8.8% vs. 4.7%, P<0.001). Patients with ARDS exhibited a higher frequency of RT breaths compared to those without ARDS (18.4% vs. 4.8%, P<0.001).
Four phenotypes of RT were detected (Figure 1). The Kappa index was 0.752 [95% confidence interval (CI): 0.740–0.764, P<0.001] between investigators in classifying RT (Table S1). Most disagreements in reads occurred between “Early RT with early relaxation” and “Early RT with delayed relaxation” (Figure S1), as well as “Mid-cycle RT” and “Late RT” (Figure S2). Mid-cycle RT was the most prevalent, accounting for 61.1% of all cases of RT, followed by BD at 16.6%. Late RT typically occurred during the transition from the ventilator-inspired phase to the expired phase. Among the patients, 11 (20.8%) experienced at least one BD event, affecting 36 waveform datasets (10.8%). Among these patients, the median incidence of BD was 8.7% (IQR, 1.7–21.6%), with a higher proportion observed in patients without ARDS (Figure 2).
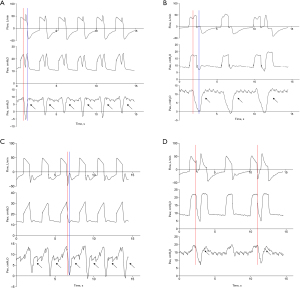
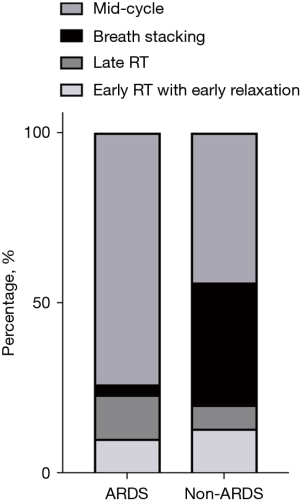
Factors associated with RT
Analgesia/sedation strategy
Patients were categorized into four groups based on their receipt of opioids/sedatives. Patients in the opioids-plus-sedatives group exhibited a higher incidence of RT and higher P0.1 compared to those in the opioids-only, sedatives-only, and no-drug groups. Additionally, this group demonstrated lower PaO2/FiO2 ratios than both the opioids-only and no-drugs groups (Figure 3). The SAS scores were similar among the opioids-plus-sedatives, opioids-only, and no-drug groups, but were significantly lower compared to the sedatives-only group [3 (IQR, 1–3) vs. 2 (IQR, 2–4) vs. 3 (IQR, 2–4) vs. 4 (IQR, 3–4), P<0.001]. Moreover, patients in the TBI and stroke groups received more frequent administration of opioids/sedatives, particularly the combination of opioids-plus-sedatives, and had lower SAS scores [2 (IQR, 2–3) vs. 2 (IQR, 2–4) vs. 4 (IQR, 3–4), P<0.001], when compared with those in the post craniotomy for brain tumor groups (P<0.001) (Figure 4).
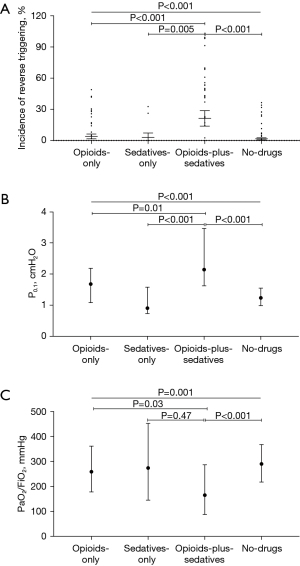
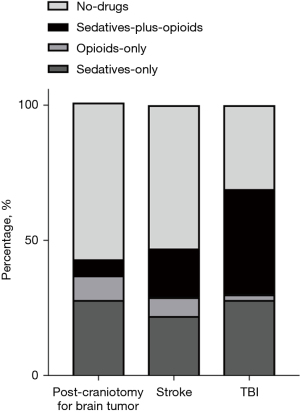
Respiratory parameters
Waveform datasets featuring RT were characterized by higher P0.1 values, smaller differences between the set respiratory rate (RRset) and the actual respiratory rate (RRact), and lower PaO2/FiO2 ratios compared to waveform datasets without RT [2.1 (IQR, 1.3–3.1) vs. 1.3 (IQR, 1.0–1.8) cmH2O, P=0.01; 0 (IQR, 0–0) vs. 0 (IQR, 0–2), P=0.001; 220.4 (IQR, 130.7–336.9) vs. 275.0 (IQR, 196.0–352.8) mmHg, P=0.06, respectively]. There were no statistically significant differences in VT and delta airway pressure (PIP-PEEP) between the two groups [8.0 (IQR, 7.4–9.5) vs. 7.9 (IQR, 7.1–8.8) mL/kg, P=0.16; 12 (IQR, 10–15) vs. 12 (IQR, 12–14) cmH2O, P=0.24, respectively].
For patients with ARDS, waveform datasets with RT showed lower VT, lower PaO2/FiO2 ratios, and a trend towards higher PIP-PEEP compared to those without RT (Figure 5). The differences between RRact and RRset were similar between these groups [0 (IQR, 0–2) vs. 0 (IQR, 0–2), P=0.34]. Conversely, among patients without ARDS, datasets with RT had larger VT, similar PaO2/FiO2 ratios, and lower PIP-PEEP compared to those without RT (Figure 5). The difference between RRset and RRact in the RT group was smaller than in the no-RT group [0 (IQR, 0–0) vs. 0 (IQR, 0–2), P=0.002].
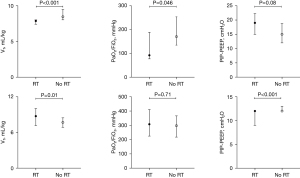
After adjusting for classification of brain injury, P0.1, VT, and PaO2/FiO2 ratio, the use of opioids-plus-sedatives, lower SAS scores, lower PIP-PEEP, and a smaller difference between RRset and RRact were independently associated with RT (Table 2).
Table 2
Factors | Odds ratio (95% CI) | P |
---|---|---|
Opioids/sedatives use | <0.001 | |
Sedatives-only | 0.388 (0.075–1.997) | 0.52 |
Opioids-plus-sedatives | 2.975 (1.278–6.925) | 0.006 |
No-drugs | 0.502 (0.220–1.144) | 0.07 |
RRact-RRset | 0.770 (0.640–0.943) | 0.01 |
PIP-PEEP | 0.877 (0.805–0.956) | 0.001 |
SAS score | 0.676 (0.481–0.951) | 0.03 |
The model is adjusted for classification of acute brain injury, P0.1, PaO2/FiO2 ratio, and tidal volume. RT, reverse triggering; CI, confidence interval; RRact, actual respiratory rate; RRset, set respiratory rate; PIP, peak inspiratory pressure; PEEP, positive end-expiratory pressure; SAS, Sedation-Agitation Scale; P0.1, pressure of occlusion at 0.1 second; PaO2, partial pressure of oxygen; FiO2, fraction of inspired oxygen.
BD
Waveform datasets with BD had higher P0.1 values and lower PaO2/FiO2 ratios compared to those without BD [2.1 (IQR, 1.7–3.3) vs. 1.3 (IQR, 1.0–2.0) cmH2O, P<0.001; 178.0 (IQR, 90.0–275.0) vs. 273.8 (IQR, 195.0–365.0) mmHg, P=0.003, respectively]. The difference between RRset and RRact was smaller in datasets with BD [0 (IQR, 0–0) vs. 0 (IQR, 0–2), P=0.007]. Furthermore, patients with BD received more opioids-plus-sedatives administration, although the SAS scores were comparable between the groups [58.3% vs. 16.4%, P<0.001; 3 (IQR, 2–3) vs. 3 (IQR, 2–4), P=0.56, respectively]. Moreover, P0.1 demonstrated robust predictive ability for BD occurrence, with the area under the curve (AUC) being 0.72 (95% CI: 0.64–0.80, P<0.001). The optimal cutoff value was determined to be 1.69 cmH2O, achieving 83.3% sensitivity and 67.1% specificity.
ΔPes originated from RT breaths was higher in BD than in other phenotypes [7.2 (IQR, 5.6–11.4) vs. 3.7 (IQR, 2.3–6.0) cmH2O, P<0.001]. However, the correlation between ΔPes of RT and P0.1 and was poor (r=0.003, P=0.98).
RT and outcomes
No significant differences were observed in ICU mortality, in-hospital mortality, duration of mechanical ventilation, or weaning rate between patients with and without RT. However, among patients who survived to hospital discharge, those with RT had a shorter ICU stay (Table 1). Among patients who survived to hospital discharge, those with RT exhibited a trend towards shorter duration of mechanical ventilation, shorter ICU stay, and shorter hospital stay [6 (IQR, 5–14) vs. 8 (IQR, 5–19) days, P=0.37; 9 (IQR, 7–20) vs. 19 (IQR, 10–30) days, P=0.049; 19 (IQR, 11–30) vs. 37 (IQR, 27–48) days, P=0.11, respectively]. Similar results were noted between patients with and without BD (Table S2). Among patients who survived to hospital discharge, those with BD exhibited shorter ICU stay [9 (IQR, 7–20) vs. 18 (IQR, 10–30) days, P=0.03].
Discussion
The main observations of this study can be summarized as follows: (I) RT was prevalent among patients with acute brain injury and who were undergoing controlled ventilation. (II) The use of opioids-plus-sedatives, lower SAS scores, lower PIP-PEEP, and a smaller difference between RRset and RRact were independently associated with RT. (III) Respiratory drive, as indicated by the P0.1 value, showed significant predictive ability for the development of BD.
Incidence of RT and associated factors
The incidence of RT in this study was 8.4% of total breaths, which with the lower range of 2.4% to 24% of all mechanical insufflations reported in previous studies (10,16,17). Sixteen patients (30.2%) experienced at least one RT event, a proportion consistent with previous findings ranging from 30% to 66.7% (4,20). An automated algorithm based on Paw and flow waveforms could detect more RT breaths than visual inspection of ventilator waveforms used in the present study (17). Furthermore, most of the previous studies were conducted among patients with ARDS, who seemed have higher incidence of RT than those without (1,4,6,7). However, only 12 (22.6%) patients were diagnosed with ARDS in the present study. Both VT and set respiratory rate could influence RT (1,16,20). Previous studies have reported mixed results regarding VT and RT. For instance, Damiani et al. found that lower VT was associated with RT in experimental pigs (7), while Rodriguez et al. reported that lower VT increased the incidence of RT in the early phase of ARDS (9). In contrast, Shimatani et al. identified that large VT was independently associated with RT in pediatric ARDS (10). In our study, datasets with RT demonstrated lower VT in patients with ARDS, but the opposite was true in patients without ARDS. After adjusting for confounding factors, VT was no longer associated with RT. Moreover, lower PIP-PEEP and a smaller difference between RRset and RRact were also associated with RT, similar to findings in Shimatani’s study of pediatric patients with ARDS (10). Periodic mechanical insufflations may synchronize the patient’s intrinsic respiratory rhythm with the ventilator, thereby facilitating patient-ventilator interaction (2,16). Decreasing the respiratory rate below the patient’s intrinsic neural rate may reduce RT breaths (1,9,21), although some studies have reported contrary findings (16,22).
The pathophysiological mechanisms of RT are not fully understood, but the predominant hypothesis is respiratory entrainment or respiratory phase locking. This involves the Hering-Breuer reflexes mediated by slowly adapting receptors, the intercostal-to-phrenic reflex, and both cortical and subcortical influences (2,4,5). The Hering-Breuer reflex may be activated during mechanical inflation to shorten the neural inspiratory time and protect the lungs from overinflation, or during the patient’s neural expiratory phase to prolong expiratory time (4). RT has frequently been reported in deeply sedated patients (1,9,17), as deep sedation may alter cortical modulation of the respiratory control center in the brainstem, increasing susceptibility to entrainment by mechanical insufflations (2,4,20). However, deep sedation was uncommon in the present study, with a median SAS score of 3 (IQR, 2–4). Patients in the stroke and TBI groups exhibited higher incidences of RT compared to those in the post craniotomy for brain tumor group (Figure 4). These groups were given more opioids-plus-sedatives and had lower SAS scores, which were independently associated with RT.
BD occurred in 16.6% of RT breaths, which is lower than reported in previous studies (10,16,17). BD was more likely to occur when the inspiratory effort generated was sufficiently large. We found that having a P0.1 ≥1.69 cmH2O was strong predictive of BD occurrence. However, patients with acute brain injury exhibited relatively low respiratory drive, as indicated by a median P0.1 of 1.4 (IQR, 1.0–2.1) cmH2O, which may explain the lower incidence of BD in our study.
Reverser triggering and outcomes
Patients with RT exhibited a trend towards shorter duration of mechanical ventilation and a reduced lengths of stay in the ICU, possibly attributable to their relatively higher P0.1 values. Respiratory drive and rhythm are controlled by neurons in the brain stem, including the rhythmicity center in the medulla and the pontine pneumotaxic center, which adjusts the rate and depth of respiration (23). Patients with acute brain injuries, especially those with infratentorial lesions, are more susceptible to impairments of the respiratory center. Approximately 43.4% of the patients in this study had infratentorial lesions, and decreased respiratory drive was a primary reason for prolonged mechanical ventilation in this population (24). Mellado Artigas et al. enrolled 39 patients with 56% having a pulmonary cause of admission but not necessarily ARDS and analyzed a 1-h recording obtained after 24 h after intubation. They reported that patients exhibiting more RT were more likely to be extubated within the next 24 hours, proposing that RT might indicate the recovery of spontaneous breathing (16). Rodriguez et al. found RT was associated with reduced hospital mortality (9). However, our study did not find significant differences in mortality between patients with and without RT. The clinical effects of RT may depend on several factors, such as phenotypes, the magnitude of inspiratory effort, lung condition, and duration of RT, all of which deserve further investigation.
Limitations
There are some limitations in this study. First, as a single-center study, the clinical procedures employed here might differ from those in other settings, potentially limiting the generalizability of our findings. Second, we analyzed only a brief period in the early phase of mechanical ventilation; thus, the short observation period may underestimate the incidence of RT. Continuous monitoring of asynchrony manually throughout the entire ventilation phase is challenging, and automatic software and algorithms could provide more precise data. Third, inspiratory efforts that occurred after the initiation of ventilator insufflation but did not follow a repetitive pattern were not identified as RT. There was no clear definition of irregular RT, which does not require a fixed repetitive pattern. Due to the retrospective nature of this study, we could not perform an expiratory occlusion to verify the association between these irregular inspiratory efforts and ventilator insufflations, potentially underestimating the incidence of RT. Finally, given the small sample size, definitive conclusions about the associations between RT and clinical outcomes remain elusive, highlighting the need for further research.
Conclusions
RT is frequently observed in patients with acute brain injury and who are undergoing controlled ventilation. The most prevalent phenotype of RT is mid-cycle RT, followed by BD. Factors such as deep sedation, lower airway delta pressure, and a closely matched respiratory rate between the ventilator and the patient are associated with the occurrence of RT. Additionally, respiratory drive, as indicated by P0.1, demonstrated robust predictive ability for the occurrence of BD.
Acknowledgments
Funding: This work was supported by a grant from
Footnote
Reporting Checklist: The authors have completed the STROBE reporting checklist. Available at https://jtd.amegroups.com/article/view/10.21037/jtd-24-694/rc
Data Sharing Statement: Available at https://jtd.amegroups.com/article/view/10.21037/jtd-24-694/dss
Peer Review File: Available at https://jtd.amegroups.com/article/view/10.21037/jtd-24-694/prf
Conflicts of Interest: All authors have completed the ICMJE uniform disclosure form (available at https://jtd.amegroups.com/article/view/10.21037/jtd-24-694/coif). The authors have no conflicts of interest to declare.
Ethical Statement: The authors are accountable for all aspects of the work in ensuring that questions related to the accuracy or integrity of any part of the work are appropriately investigated and resolved. The study was conducted in accordance with the Declaration of Helsinki (as revised in 2013). The use of anonymized data was approved by the Institutional Review Board of Beijing Tiantan Hospital, Capital Medical University (approval No. KY 2017-028-02) and the informed consent was waived due to the retrospective nature of this study.
Open Access Statement: This is an Open Access article distributed in accordance with the Creative Commons Attribution-NonCommercial-NoDerivs 4.0 International License (CC BY-NC-ND 4.0), which permits the non-commercial replication and distribution of the article with the strict proviso that no changes or edits are made and the original work is properly cited (including links to both the formal publication through the relevant DOI and the license). See: https://creativecommons.org/licenses/by-nc-nd/4.0/.
References
- Akoumianaki E, Lyazidi A, Rey N, et al. Mechanical ventilation-induced reverse-triggered breaths: a frequently unrecognized form of neuromechanical coupling. Chest 2013;143:927-38. [Crossref] [PubMed]
- Graves C, Glass L, Laporta D, et al. Respiratory phase locking during mechanical ventilation in anesthetized human subjects. Am J Physiol 1986;250:R902-9. [Crossref] [PubMed]
- Simon PM, Zurob AS, Wies WM, et al. Entrainment of respiration in humans by periodic lung inflations. Effect of state and CO(2). Am J Respir Crit Care Med 1999;160:950-60. [Crossref] [PubMed]
- Rodrigues A, Telias I, Damiani LF, et al. Reverse Triggering during Controlled Ventilation: From Physiology to Clinical Management. Am J Respir Crit Care Med 2023;207:533-43. [Crossref] [PubMed]
- Delisle S, Charbonney E, Albert M, et al. Patient-Ventilator Asynchrony due to Reverse Triggering Occurring in Brain-Dead Patients: Clinical Implications and Physiological Meaning. Am J Respir Crit Care Med 2016;194:1166-8. [Crossref] [PubMed]
- Baedorf Kassis E, Su HK, Graham AR, et al. Reverse Trigger Phenotypes in Acute Respiratory Distress Syndrome. Am J Respir Crit Care Med 2021;203:67-77. [Crossref] [PubMed]
- Damiani LF, Engelberts D, Bastia L, et al. Impact of Reverse Triggering Dyssynchrony during Lung-Protective Ventilation on Diaphragm Function: An Experimental Model. Am J Respir Crit Care Med 2022;205:663-73. [Crossref] [PubMed]
- Pellegrini M, Hedenstierna G, Roneus A, et al. The Diaphragm Acts as a Brake during Expiration to Prevent Lung Collapse. Am J Respir Crit Care Med 2017;195:1608-16. [Crossref] [PubMed]
- Rodriguez PO, Tiribelli N, Fredes S, et al. Prevalence of Reverse Triggering in Early ARDS: Results From a Multicenter Observational Study. Chest 2021;159:186-95. [Crossref] [PubMed]
- Shimatani T, Yoon B, Kyogoku M, et al. Frequency and Risk Factors for Reverse Triggering in Pediatric Acute Respiratory Distress Syndrome during Synchronized Intermittent Mandatory Ventilation. Ann Am Thorac Soc 2021;18:820-9. [Crossref] [PubMed]
- Luo XY, He X, Zhou YM, et al. Patient-ventilator asynchrony in acute brain-injured patients: a prospective observational study. Ann Intensive Care 2020;10:144. [Crossref] [PubMed]
- Baydur A, Behrakis PK, Zin WA, et al. A simple method for assessing the validity of the esophageal balloon technique. Am Rev Respir Dis 1982;126:788-91. [Crossref] [PubMed]
- Chiumello D, Consonni D, Coppola S, et al. The occlusion tests and end-expiratory esophageal pressure: measurements and comparison in controlled and assisted ventilation. Ann Intensive Care 2016;6:13. [Crossref] [PubMed]
- Telias I, Damiani F, Brochard L. The airway occlusion pressure (P0.1) to monitor respiratory drive during mechanical ventilation: increasing awareness of a not-so-new problem. Intensive Care Med 2018;44:1532-5. [Crossref] [PubMed]
- Murias G, de Haro C, Blanch L. Does this ventilated patient have asynchronies? Recognizing reverse triggering and entrainment at the bedside. Intensive Care Med 2016;42:1058-61. [Crossref] [PubMed]
- Mellado Artigas R, Damiani LF, Piraino T, et al. Reverse Triggering Dyssynchrony 24 h after Initiation of Mechanical Ventilation. Anesthesiology 2021;134:760-9. [Crossref] [PubMed]
- Pham T, Montanya J, Telias I, et al. Automated detection and quantification of reverse triggering effort under mechanical ventilation. Crit Care 2021;25:60. [Crossref] [PubMed]
- Yoshida T, Nakamura MAM, Morais CCA, et al. Reverse Triggering Causes an Injurious Inflation Pattern during Mechanical Ventilation. Am J Respir Crit Care Med 2018;198:1096-9. [Crossref] [PubMed]
- Su HK, Loring SH, Talmor D, et al. Reverse triggering with breath stacking during mechanical ventilation results in large tidal volumes and transpulmonary pressure swings. Intensive Care Med 2019;45:1161-2. [Crossref] [PubMed]
- Lin Z, Zhou J, Lin X, et al. Reverse Trigger in Ventilated Non-ARDS Patients: A Phenomenon Can Not Be Ignored! Front Physiol 2021;12:670172. [Crossref] [PubMed]
- He X, Luo XY, Chen GQ, et al. Detection of reverse triggering in a 55-year-old man under deep sedation and controlled mechanical ventilation. J Thorac Dis 2018;10:E682-5. [Crossref] [PubMed]
- Jochmans S, Mazerand S, Mercier des Rochettes E, et al. Reverse triggering with breath stacking in ARDS patients: the "optimum" can be the enemy of the "good". Minerva Anestesiol 2018;84:871-2. [Crossref] [PubMed]
- Ciumas C, Rheims S, Ryvlin P. fMRI studies evaluating central respiratory control in humans. Front Neural Circuits 2022;16:982963. [Crossref] [PubMed]
- Luo XY, He X, Zhou YM, et al. Ineffective Effort in Patients With Acute Brain Injury Undergoing Invasive Mechanical Ventilation. Respir Care 2023;68:1202-12. [Crossref] [PubMed]