Neoadjuvant immunology therapy in patients with non-small cell lung cancer and chronic obstructive pulmonary disease
Highlight box
Key findings
• This study found that for resectable non-small cell lung cancer (NSCLC) patients with chronic obstructive pulmonary disease (COPD), neoadjuvant immunotherapy could achieve better pathological response, survival benefit, and improve patients’ lung function.
What is known and what is new?
• For resectable NSCLC patients, neoadjuvant immunotherapy could achieve better pathological remission and survival benefits.
• For resectable NSCLC patients with COPD, major pathological response, and pathological complete response of neoadjuvant immunotherapy were 55.6% and 38.9%, respectively. Neoadjuvant immunotherapy could influence patients’ lung function, the patient’s pulmonary ventilation improved and diffusion function decreased.
What is the implication, and what should change now?
• The results of the study may help inform physicians when evaluating whether neoadjuvant immunotherapy and surgery can be performed in NSCLC patients with comorbid COPD.
Introduction
Lung cancer is the leading cause of cancer incidence and mortality both in the world and China (1-3). Non-small cell lung cancer (NSCLC) accounts for 80–85% of all lung cancer cases, of which 30–40% are resectable cancers, including early stage (stage I–II) and locally advanced (stage IIIA–IIIB) (4,5).
In recent years, immunotherapy is rapidly moving forward from treating advanced NSCLC to earlier stages of the disease, and related research has explored extending from palliative care to neoadjuvant and adjuvant therapy (6-8). The results of several key clinical trials of neoadjuvant therapy (CheckMate-816, NADIM II, and Keynote 671) have shown that neoadjuvant immunotherapy can reduce the size of primary tumors [objective response rate (ORR): 54% vs. 37.4%], lower the staging [major pathological response (MPR): 30.2–53.0% vs. 8.9–14.0%; pathological complete response (pCR): 18.1–37.0% vs. 2.2–7.0%], increase the rate of complete surgical resection (R0: 83.2–82.0% vs. 77.8–85.0%) and prolong patient survival [24-month event-free survival (EFS) rate: 62.4–67.2% vs. 40.6–47.0%; 24-month overall survival (OS) rate: 8.09–83.0% vs. 63.6–77.6%] (9-11). The CheckMate-816 study showed that 83.2% and 75.4% of patients in the neoadjuvant immunotherapy combined with chemotherapy group and the chemotherapy group, respectively, underwent radical surgery, with similar rates of surgical delay between the two groups (20.8% vs. 17.8%). Although there was no significant difference statistically, the immune group had a shorter surgery time (185 vs. 213.5 min), a higher proportion of minimally invasive surgeries (29.5% vs. 21.5%), and a lower total lung resection rate (16.8% vs. 25.2%) compared to the chemotherapy group. In summary, neoadjuvant immunotherapy combined with chemotherapy provides more patients with surgical opportunities compared to chemotherapy alone, without increasing the difficulty and complexity of the surgery (9). Therefore, immunoadjuvant therapy has become a new hot spot in the treatment of NSCLC.
Chronic obstructive pulmonary disease (COPD) is the most common comorbidity in NSCLC patients and the prevalence is estimated between 32.0% and 62.9% (12-14). A systematic review based on seven case-control studies showed that COPD patients had a 6.35-fold increased risk of lung cancer compared with non-COPD patients (15). However, in the actual clinical work, doctors often pay insufficient attention to the diagnosis and treatment of lung cancer complicated with COPD, and the studies in this field are also insufficient. At present, it is generally believed that COPD is an independent risk factor affecting the OS of patients with lung cancer. With the wide application of immunotherapy in the field of lung cancer, some studies have explored the therapeutic effect of immunotherapy in patients with advanced lung cancer complicated with COPD, but for patients with early lung cancer complicated with COPD, the application effect and safety of immunotherapy are still unclear.
In this study, we summarized the clinical and therapeutic data of 57 patients with stage IB to IIIB NSCLC who received neoadjuvant immunotherapy, of which 18 (31.6%) were complicated with COPD. By comparing the two groups of patients, we evaluated the differences of neoadjuvant immunotherapy in the treatment efficacy, surgical difficulty, and safety between COPD patients and non-COPD patients. We present this article in accordance with the STROBE reporting checklist (available at https://jtd.amegroups.com/article/view/10.21037/jtd-24-811/rc).
Methods
Patients
We retrospectively collected and analyzed the data of NSCLC patients who had received neoadjuvant immunotherapy followed by surgery at Shanghai Chest Hospital between November 2018 and May 2021. All information of these patients was identified from our electronic medical records system. The patients who met the following criteria were included in the study: (I) pathologically or cytologically confirmed NSCLC; (II) confirmed with a diagnosis of stage IB to IIIB [the stage was performed according to the eighth edition of the tumor-node-metastasis (TNM) classification for NSCLC, phase IIIB includes only T3N2 and T4N2] (4); (III) underwent neoadjuvant immunotherapy followed by surgery; (IV) EGFR, ALK, and ROS1 mutation status of patients were confirmed negative by the amplification refractory mutation system-polymerase chain reaction (ARMS-PCR) or next-generation sequencing (NGS). The baseline clinical characteristics included age, gender, body mass index (BMI), smoking history, programmed cell death-ligand 1 (PD-L1) expression status, histology, and clinical stage.
The study was conducted in accordance with the Declaration of Helsinki (as revised in 2013). The study was approved by the independent ethics committee of Shanghai Chest Hospital (No. KS22002) and informed consent was obtained from all individual participants.
Pulmonary function assessment
Patients were asked to have pulmonary function testing at the time of diagnosis and prior to surgery using the same methods and instruments. Pulmonary function parameters included forced expiratory volume in 1 second (FEV1), the ratio of FEV1 to the predicted value (FEV1%pred), forced vital capacity (FVC), the ratio of FVC to the predicted value (FVC%pred), FEV1/FVC ratio, carbon monoxide diffusing capacity (DLCO), and DLCO adjusted by hemoglobin (DLCOc). FEV1 and FVC were measured by a differential pressure flow sensor after guiding the patient to complete a flow-volume curve, and DLCO was measured by a helium dilution method after guiding the patient to complete a single breath. The instrument used was a JAEGER Master Screen Body.
All the patients were divided into COPD or non-COPD subgroups according to their first pulmonary function results. The Global Initiative for COPD criteria were used to assess the presence of COPD, and the COPD group enrolled patients with the FEV1/FVC ratio <70% (16).
All patients with COPD were evaluated for symptom load by the COPD Assessment Test (CAT) at the time of diagnosis, before surgery and 1 month after operation.
Treatment and follow-up
Patients were administered at least two cycles of neoadjuvant immunotherapy with or without chemotherapy, and the regimen was determined according to the decision of oncologists. After neoadjuvant therapy, all patients must undergo standard preoperative staging workup that included contrast-enhanced computed tomography (CT) scan and brain magnetic resonance imaging (MRI), or positron emission tomography (PET)-CT. The response evaluation was assessed according to the Response Evaluation Criteria in Solid Tumors (RECIST; guideline 1.1), which defined complete response (CR), partial response (PR), stable disease (SD), progressive disease (PD), ORR, and disease control rate (DCR) (17).
Surgical information included extent of resection, style of surgery, surgical margin, operation time, estimated blood loss, interval time from the last neoadjuvant to surgery and complications. The pathological effect was assessed by pathologist according to the expert consensus on the pathological evaluation of neoadjuvant therapy efficacy for NSCLC (18). MPR was defined as the percentage of residual tumor cells in the tumor bed ≤10%. pCR was defined as tumor regression with no residual tumor on pathology. After surgery, different adjuvant treatment schemes were adopted according to the individual clinical condition of the patient. Adverse events were defined according to the National Cancer Institute Common Terminology Criteria for Adverse Events (CTCAE; version 5.0).
EFS was determined from the diagnosis date to any progression or recurrence of disease after surgery, progression of disease in the absence of surgery, or death from any cause. OS was defined as the time from the diagnosis date to the date of death or last follow-up visit (July 19, 2022).
Statistical analysis
Continuous variables were expressed as mean or median and were tested for the normal distribution, the normally distributed and abnormally distributed continuous variables were analyzed by the paired t-test and Wilcoxon rank sum test, respectively. Categorical variables were described by the means of absolute and percentage numbers and compared with Chi-squared test or Fisher’s exact test as appropriate. The survival curves of EFS and OS were estimated according to Kaplan-Meier method. ORR, DCR, MPR, and CPR for each group were compared using Chi-squared test or Fisher’s exact test when appropriate. Statistical significance was defined as P value <0.05. All analyses were performed with the SPSS software, version 25 (IBM, Armonk, NY, USA).
Results
Patient characteristic information
From November 2018 to May 2021, a total of 59 patients received neoadjuvant immunotherapy. However, one patient had NSCLC with an unclassifiable pathological type, and another patient had an indeterminate ROS1 gene mutation status. Therefore, a total of 57 patients were ultimately enrolled in our study. Characteristics of patients are summarized in Table 1. Mean age for all patients was 60.6 years, 54 (94.7%) of them were male, and 45 patients (78.9%) were current or former smokers. There were 41 patients (71.9%) of squamous cell carcinoma and 16 patients (28.1%) of adenocarcinoma. The primary clinical stage of patients ranges from IB to IIIB, majority of patients had stage IIIA disease (29/57, 50.9%), 19 patients (33.3%) had stage IIIB disease, 4 patients (7.0%) had stage IIB disease, three (5.3%) had stage IB disease and 2 (3.5%) had stage IIA.
Table 1
Characteristics | All patients (n=57) | With COPD (n=18) | No COPD (n=39) | P value |
---|---|---|---|---|
Age (years) | 60.6±7.9 | 64.8±5.4 | 58.7±8.2 | 0.005* |
Sex | 0.54 | |||
Male | 54 (94.7) | 18 (100.0) | 36 (92.3) | |
Female | 3 (5.3) | 0 | 3 (7.7) | |
BMI (kg/m2) | 24.4±3.1 | 23.7±3.0 | 24.7±3.1 | 0.28 |
Smoking history | 0.02* | |||
Never | 12 (21.1) | 0 | 12 (30.8) | |
Former | 7 (12.3) | 3 (16.7) | 4 (10.3) | |
Current | 38 (66.7) | 15 (83.3) | 23 (59.0) | |
PD-L1 expression | 0.90 | |||
<1% | 7 (12.3) | 3 (16.7) | 4 (10.3) | |
1–49% | 14 (24.6) | 5 (27.8) | 9 (23.1) | |
≥50% | 19 (33.3) | 5 (27.8) | 14 (35.9) | |
Unknown | 17 (29.8) | 5 (27.8) | 12 (30.8) | |
Histology | 0.23 | |||
Adenocarcinoma | 16 (28.1) | 3 (16.7) | 13 (33.3) | |
Squamous cell carcinoma | 41 (71.9) | 15 (83.3) | 26 (66.7) | |
Clinical stage | 0.50 | |||
IB | 3 (5.3) | 2 (11.1) | 1 (2.6) | |
IIA | 2 (3.5) | 0 | 2 (5.1) | |
IIB | 4 (7.0) | 2 (11.1) | 2 (5.1) | |
IIIA | 29 (50.9) | 9 (50.0) | 20 (51.3) | |
IIIB | 19 (33.3) | 5 (27.8) | 14 (35.9) | |
Gene test by NGS | 0.85 | |||
Yes | 39 (68.4) | 12 (66.7) | 27 (69.2) | |
No | 18 (31.6) | 6 (33.3) | 12 (30.8) | |
Adjuvant treatment | 0.55 | |||
Yes | 40 (70.2) | 13 (72.2) | 27 (69.2) | 0.33 |
Immunotherapy alone | 9 (22.5) | 1 (7.7) | 8 (29.6) | |
Chemotherapy alone | 10 (25.0) | 4 (30.8) | 6 (22.2) | |
ICIs and chemotherapy | 21 (52.5) | 8 (61.5) | 13 (48.1) | |
No | 17 (29.8) | 5 (27.8) | 12 (30.8) |
Values are presented as mean ± standard deviation or n (%). *, P<0.05. COPD, chronic obstructive pulmonary disease; BMI, body mass index; PD-L1, programmed death-ligand 1; NGS, next-generation sequencing; ICI, immune checkpoint inhibitor.
Among these patients, 18 (31.6%) were diagnosed with COPD. Patients with COPD were older (mean, 64.8 vs. 58.7 years, P=0.005) than patients without COPD. All COPD patients were male and all were former or current smokers. Among patients with COPD (n=18), 83.3% were squamous cell carcinoma, which is higher than that in the non-COPD group (66.7%), although it was not statistically significant (P=0.23). No differences in BMI, PD-L1 expression, and clinical stage were observed between the two groups (Table 1).
Neoadjuvant therapy and clinical response
Four patients (7.0%) received preoperative mono-immunotherapy, and 53 patients (93.0%) received immunotherapy plus chemotherapy (Table 2). The number of COPD patients who received immune checkpoint inhibitors (ICIs) combined with chemotherapy as neoadjuvant regimen was similar than that of non-COPD (94.4% vs. 92.3%, P=0.63).
Table 2
Characteristics | All patients (n=57) | With COPD (n=18) | No COPD (n=39) | P value |
---|---|---|---|---|
Neoadjuvant therapy regimens | 0.63 | |||
ICIs alone | 4 (7.0) | 1 (5.6) | 3 (7.7) | |
ICIs and chemotherapy | 53 (93.0) | 17 (94.4) | 36 (92.3) | |
Course of neoadjuvant treatment | 0.28 | |||
Two | 32 (56.1) | 10 (55.6) | 22 (56.4) | |
Three | 16 (28.1) | 7 (38.9) | 9 (23.1) | |
Four | 9 (15.8) | 1 (5.6) | 8 (20.5) | |
DCR | 57 (100.0) | – | – | – |
ORR | 32 (56.1) | 8 (44.4) | 24 (61.5) | 0.23 |
Values are presented as n (%). COPD, chronic obstructive pulmonary disease; ICI, immune checkpoint inhibitor; DCR, disease control rate; ORR, objective response rate.
All of the patients received at least two courses neoadjuvant therapy, and 16 patients (28.1%) and 9 patients (15.8%) received three and four courses of preoperative immunotherapy. There was no difference in neoadjuvant treatment course between patients with COPD and those without COPD (P=0.28; Table 2).
After neoadjuvant treatment, 32 patients (56.1%) had a PR and 25 patients (43.9%) had SD (Figure 1). ORRs (44.4% vs. 61.5%, P=0.23) were similar between patients with COPD and without COPD (Table 2). A total of 25 patients (43.9%) reached the radiological down-staging.
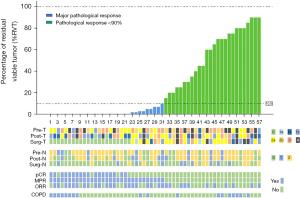
Surgery details
There were 30 patients (52.6%) and 27 patients (47.4%) underwent video-assisted thoracoscopic surgery (VATS) and open thoracotomy, respectively (Table 3), and there was no difference in VATS rates between COPD and non-COPD patients (55.6% vs. 51.3%, P=0.76). For 30 patients who underwent VATS, 3 patients (10.0%) experienced intraoperative conversion to open thoracotomy, included one patient with COPD and two patients without COPD.
Table 3
Characteristics | All patients (n=57) | With COPD (n=18) | No COPD (n=39) | P value |
---|---|---|---|---|
Complete resection (R0) | 52 (91.2) | 17 (94.4) | 35 (89.7) | >0.99 |
VATS | 30 (52.6) | 10 (55.6) | 20 (51.3) | 0.76 |
Conversion to open | 3 (10.0) | 1 (10.0) | 2 (10.0) | – |
Extent of resection | 0.009* | |||
Lobectomy | 41 (71.9) | 9 (50.0) | 32 (82.1) | |
Multiple lobectomy | 10 (17.5) | 4 (22.2) | 6 (15.4) | |
Pneumonectomy | 3 (5.3) | 2 (11.1) | 1 (2.6) | |
Sleeve lobectomy | 3 (5.3) | 3 (16.7) | 0 | |
Operative time (min) | 138.50±51.01 | 157.50±52.58 | 131.59±49.80 | 0.23 |
Estimated blood loss (mL) | 189.44±230.37 | 226.11±323.82 | 171.11±168.50 | 0.41 |
Interval time from the last neoadjuvant to surgery (days) | 35.58±13.39 | 39.44±14.41 | 33.79±12.69 | 0.14 |
Postoperative complications | – | |||
Chylothorax | 2 (3.5) | 1 (5.6) | 1 (2.6) | |
Transfusions | 1 (1.8) | 0 | 1 (2.6) | |
Pneumonia | 1 (1.8) | 0 | 1 (2.6) | |
Wound infection | 1 (1.8) | 0 | 1 (2.6) | |
Arrhythmia | 1 (1.8) | 1 (5.6) | 0 | |
The percentage of RVT cells (%) | 26.6±32.5 | 31.6±37.4 | 24.3±30.2 | 0.47 |
pCR | 18 (31.6) | 7 (38.9) | 11 (28.2) | 0.42 |
MPR | 31 (54.4) | 10 (55.6) | 21 (53.8) | 0.90 |
Values are presented as n (%) or mean ± standard deviation. *, P<0.05. COPD, chronic obstructive pulmonary disease; VATS, video-assisted thoracic surgery; RVT, residual viable tumor; pCR, pathological complete response; MPR, major pathological response.
A total of 52 patients (91.2%) achieved R0 resection, and the remaining 5 patients underwent R1 resection, included 1 (5.6%) patient with COPD and 4 (10.3%) patients without COPD (P>0.99, Table 3). The most common type of surgery was lobectomy (41/57, 71.9%), followed by multiple lobectomy (10/57, 17.5%), pneumonectomy (3/57, 5.3%) and sleeve lobectomy (3/57, 5.3%). COPD patients had fewer lobectomy (50.0% vs. 82.1%) and more sleeve lobectomy (16.7% vs. 0, P=0.009) compared to non-COPD patients.
The mean operative time was 138.50 min (Table 3). The mean estimated blood loss was 189.44 ml. The mean interval of time between the final neoadjuvant therapy and surgery was 35.58 days. Patients with COPD were slightly longer or more than those without COPD in terms of operation time (157.50 vs. 131.59 min), blood loss (226.11 vs. 171.11 mL), and interval time (39.44 vs. 33.79 days), but there was no statistical difference between the two groups (P=0.23, P=0.41, P=0.14, respectively).
No patient experienced perioperative death. Six patients (10.5%) developed postoperative complications, the most common complication was chylothorax (2/57, 3.5% patients). One person each with complications of blood transfusion, pneumonia, wound infection, and arrhythmia.
Pathologic response and adjuvant treatment
According to postoperative pathological results, pCR was achieved in 18 (31.6%) of 57 patients who underwent surgery, including 7 (38.9%) patients with COPD and 11 (28.2%, P=0.42) patients without COPD (Table 3). A total of 31 (54.4%) patients had MPR, and the MPR rates of COPD patients and non-COPD patients were 55.6% and 53.8%, respectively (P=0.90). The mean percentage of residual viable tumor (RVT) was 26.6%, and there was no difference between COPD or non-COPD patients (31.6% vs. 24.3%, P=0.47; Table 3). Details of RVT are shown in Figure 1.
After surgery, a total of 40 (70.2%) patients received adjuvant therapy, included 13 (72.2%) patients with COPD and 27 (69.2%, P=0.55) patients without COPD (Table 1). Among 18 patients who achieved pCR, 12 of them received adjuvant therapy (P=0.69; Table S1).
EFS and OS
As at 2 patients (10.5%) with stage IIIB underwent sleeve lobectomy, which seems to be more than in non-stage IIIB patients (1, 2.6%, P=0.57). July 2022, the median follow-up time was 454 days (range, 47–1,088 days). At the end of follow-up, nine patients (15.8%) had tumor relapse and three patients (5.3%) died of tumor recurrence. The median EFS and OS were not reached. EFS was 93.0% at 6 months, 93.0% at 12 months, 88.8% at 18 months, and 83.7% at 24 months (Figure 2A). OS was 98.2% at 6 months, 96.5% at 12 and 18 months, and 94.6% at 24 months (Figure 2B).
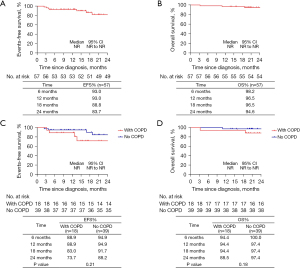
For 18 patients with COPD, 88.9% of patients were progression-free at 12 months and 73.7% at 24 months, and for 39 patients without COPD, EFS was 94.9% at 12 months and 88.2% at 24 months (P=0.21; Figure 2C). OS was 94.4% at 12 months and 88.5% at 24 months in COPD patients and 97.4% at 12 and 24 months in non-COPD patients (P=0.18; Figure 2D).
All 18 patients who achieved pCR had no disease progression. Among the 39 patients who did not achieve pCR, the EFS was 89.7% at 12 months and 76.7% at 24 months (P=0.04; Figure S1A), and the OS was 94.9% at 12 months and 92.2% at 24 months (P=0.24; Figure S1B).
For MPR patients (n=31), EFS at 12 and 24 months was 100% and 91.9%, respectively, and non-MPR patients (n=26) were 84.6% and 74.7%, respectively (P=0.07; Figure S1C). 24 months OS rate of MPR patients was 100%, OS rate was 92.3% at 12 months and 88.5% at 24 months in non-MPR patients (P=0.059; Figure S1D).
For 40 patients with adjuvant therapy, EFS was 97.5% at 12 months and 87.8% at 24 months, and appeared to be higher than in patients who did not receive adjuvant therapy (n=17, 82.4% at 12 months and 75.5% at 24 months), although the difference was not statistically significant (P=0.09; Figure S2A). OS was 97.5% at 12 and 24 months in patients with adjuvant therapy, 94.1% at 12 months, and 87.8% at 24 months in patients without adjuvant therapy (P=0.16; Figure S2B).
For the 18 pCR patients, none experienced disease progression. For 39 patients without pCR, there was 28 patients with adjuvant therapy and 11 patients without adjuvant therapy. Five and four patients were progression-free at the last follow-up, respectively, EFS at 12 and 24 months was 96.4% and 82.8% for patients with adjuvant therapy, and patients without adjuvant therapy was 72.7% and 62.3%, respectively (P=0.059; Figure S2C). OS was 96.4% at 12 and 24 months for patients with adjuvant therapy, 90.9% at 12 months, and 81.8% at 24 months for patients without adjuvant therapy (P=0.13; Figure S2D).
Results for stage IIIB patients
In this study, among 57 patients, a total of 19 (33.3%) patients had stage IIIB disease. There was no difference in neoadjuvant regimen for stage IIIB and non-stage IIIB patients (P=0.59). More patients with stage IIIB had three (31.6% vs. 26.3%) or four (21.1% vs. 13.2%) courses of neoadjuvant therapy than patients with non-stage IIIB, although the difference was not statistically significant (P=0.60). And there was no difference in the ORR between stage IIIB and non-stage IIIB patients (57.9% vs. 55.3%, P=0.85). All details were shown in Table S2.
About the details of surgery, there was no difference in the rate of R0, VATS, operative time, interval time from the last neoadjuvant to surgery and the percentage of RVT cells between stage IIIB and non-stage IIIB patients (Table S3). A total of three patients experienced intraoperative conversion to open thoracotomy, and all of these patients were stage IIIB and no patient was non-stage IIIB (25.0% vs. 0, P=0.054). Two patients (10.5%) with stage IIIB underwent sleeve lobectomy, which seems to be more than that in non-stage IIIB patients (1, 2.6%, P=0.57). And for patients with stage IIIB, the estimated blood loss was more than patients with non-stage IIIB, however, the difference was also not statistically significant (244.12 vs. 164.32, P=0.34).
In addition, stage IIIB patients had lower pCR (26.3% vs. 34.2%, P=0.55) and MPR (42.1% vs. 60.5%, P=0.12) rates than non-stage IIIB patients, but neither difference was statistically significant (Table S2).
For 19 stage IIIB patients, 84.2% of patients were progression-free at 12 and 24 months, and for 38 non-stage IIIB patients, EFS was 97.4% at 12 months and 82.3% at 24 months (P=0.84; Figure S3A). OS was 94.7% at 12 months and 89.2% at 24 months in stage IIIB patients and 97.4% at 12 and 24 months in non-stage IIIB patients (P=0.21; Figure S3B).
Pulmonary function assessment
A total of 31 patients underwent pulmonary function tests both before and after neoadjuvant therapy and the outcomes are shown in Table 4. After neoadjuvant immunotherapy, FEV1, FEV1%pred, FVC, and FVC%pred were all improved and the differences were statistically significant (P values: 0.008, 0.02, 0.02, 0.041, respectively). For 25 patients with DLCO assessment, mean DLCO decreased from 83.43 before treatment to 78.55, with a statistically significant difference (P=0.02), while the decrease in DLCOc was even more pronounced (P=0.007).
Table 4
Characteristics | All patients (n=31) | With COPD (n=11) | |||||
---|---|---|---|---|---|---|---|
Before neoadjuvant therapy | After neoadjuvant therapy | P value | Before neoadjuvant therapy | After neoadjuvant therapy | P value | ||
FEV1 (L) | 2.44±0.62 | 2.58±0.63 | 0.008* | 2.00±0.50 | 2.17±0.44 | 0.047* | |
FEV1%pred | 80.83±16.47 | 85.45±15.63 | 0.02* | 66.16±12.82 | 71.90±10.37 | 0.052 | |
FVC (L) | 3.41±0.81 | 3.57±0.77 | 0.02* | 3.33±0.87 | 3.46±0.73 | 0.17 | |
FVC%pred | 83.08±20.71 | 89.42±14.32 | 0.041* | 75.55±28.28 | 85.68±15.31 | 0.19 | |
FEV1/FVC ratio (%) | 71.97±10.75 | 72.48±8.87 | 0.63 | 60.89±8.83 | 63.42±8.22 | 0.15 | |
DLCO (%) | 83.43±12.86 | 78.55±13.58 | 0.02* | 74.56±12.46 | 71.38±3.68 | 0.46 | |
DLCOc (%) | 80.83±12.33 | 75.65±12.26 | 0.007* | 71.61±10.53 | 69.28±4.20 | 0.50 |
Values are presented as mean ± standard deviation. *, P<0.05. COPD, chronic obstructive pulmonary disease; FEV1, forced expiratory volume in 1 second; FEV1%pred, the ratio of FEV1 to the predicted value; FVC, forced vital capacity; FVC%pred, the ratio of FVC to the predicted value; DLCO, carbon monoxide diffusing capacity; DLCOc, DLCO adjusted by hemoglobin.
For 11 patients with COPD and twice pulmonary function tests, the alteration in lung function was manifested only in FEV1, where the mean value increased from 2.00 to 2.17 (P=0.047). Other indicators, including FEV1%pred, FVC, FVC%pred, FEV1/FVC ratio, and DLCO, showed no significant improvement before and after immunotherapy. Details showed in Table 4.
Of the 18 COPD patients, only three regularly used bronchodilator. The mean CAT of patients was 7.17, 7.06, and 7.44 at the time of diagnosis, before surgery and 1 month after operation, respectively. The changes of CAT before and after immunotherapy and surgery were not statistically significant (P=0.50 and P=0.11, respectively).
Adverse events during neoadjuvant therapy
For neoadjuvant therapy, the most common adverse event of grade 1–2 was alopecia (26/57, 45.6%), followed by fatigue (22/57, 38.6%), leukopenia (17/57, 29.8%), myalgia (13/57, 22.8%), and rash (12/57, 21.1%; Table 5). The adverse events of grade 3–4 included leukopenia (6/57, 10.5%), alopecia (2/57, 3.5%), rash (2/57, 3.5%), thrombocytopenia (1/57, 1.8%), hypohepatia (1/57, 1.8%), and fatigue (1/57, 1.8%). No patients discontinued treatment due to adverse reactions.
Table 5
Characteristics | Neoadjuvant therapy (n=57) | Adjuvant therapy (n=40) | |||
---|---|---|---|---|---|
Grade 1–2 | Grade 3–4 | Grade 1–2 | Grade 3–4 | ||
Leukopenia | 17 (29.8) | 6 (10.5) | 9 (22.5) | 5 (12.5) | |
Thrombocytopenia | 7 (12.3) | 1 (1.8) | 4 (10.0) | 0 | |
Anemia | 3 (5.3) | 0 | 2 (5.0) | 1 (2.5) | |
Hypohepatia | 4 (7.0) | 1 (1.8) | 3 (7.5) | 1 (2.5) | |
Fatigue | 22 (38.6) | 1 (1.8) | 17 (42.5) | 2 (5.0) | |
Nausea | 5 (8.8) | 0 | 3 (7.5) | 0 | |
Constipation | 10 (17.5) | 0 | 4 (10.0) | 0 | |
Diarrhoea | 4 (7.0) | 0 | 3 (7.5) | 0 | |
Alopecia | 26 (45.6) | 2 (3.5) | 5 (12.5) | 0 | |
Myalgia | 13 (22.8) | 0 | 7 (17.5) | 0 | |
Arthralgia | 5 (8.8) | 0 | 3 (7.5) | 0 | |
Rash | 12 (21.1) | 2 (3.5) | 4 (10.0) | 0 | |
Pneumonia | 2 (3.5) | 0 | 1 (2.5) | 0 | |
Neurotoxicity | 5 (8.8) | 0 | 2 (5.0) | 0 |
Values are presented as n (%).
Fatigue (17/40, 42.5%) and leukopenia (9/40, 22.5%) were common adverse events during adjuvant therapy, other adverse events were similar to neoadjuvant treatment.
Biomarkers
A total of 39 patients underwent gene testing by NGS method, the common mutation types were TP53, FGFR1, CDKN2A, DPYD, KEAP1, PIK3CA, STK11, BRCA1, and BRAF. Details were shown in Figure 3A.
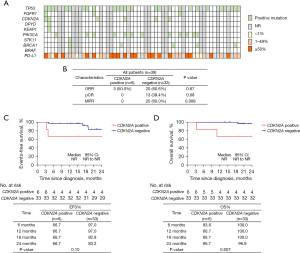
It was worth noting that among these mutation sites, a total of six patients had CDKN2A homozygous deletion, and none of these 6 patients achieved pCR and MPR (P=0.08 and P=0.008, respectively; Figure 3B). For 6 patients with CDKN2A positive mutation, 66.7% of patients were progression-free at 12 and 24 months, and for 33 patients with CDKN2A negative mutation, EFS was 97.0% at 12 months and 83.2% at 24 months (P=0.10; Figure 3C). OS was 66.7% at 12 and 24 months in CDKN2A positive mutation patients and 100.0% at 12 months and 96.6% at 24 months in patients with CDKN2A negative mutation (P=0.007; Figure 3D).
A total of 40 patients had the level of PD-L1, and there was no correlation between PD-L1 expression level and ORR, pCR, and MPR rate (P=0.47, P=0.81, and P>0.99, respectively).
Discussion
Firstly, this study confirmed the safety and efficacy of neoadjuvant immunotherapy in patients with resectable NSCLC. Secondly, this study focused for the first time on the efficacy and safety of neoadjuvant immunotherapy in NSCLC patients combined with COPD and the effect on patients’ lung function.
Smoking, chronic inflammatory response and airway remodeling are common mechanisms in COPD combined with lung cancer, however, clinicians and patients tend to focus on the tumor, which has a higher morbidity and mortality rate, and tend to blame the tumor for the exacerbation of respiratory symptoms during treatment, often ignoring the role played by the combined underlying disease such as COPD. In fact, compared to the NSCLC alone group, patients with COPD were older, had a higher proportion of male, with a history of smoking in more than 80% of patients, lower BMI, a higher proportion of combined emphysema and more severe airway obstruction, and all of which are often independent risk factors for OS in NSCLC patients (19,20). COPD and lung cancer are homologous diseases, which should be diagnosed and treated as early as possible.
However, previous studies have shown that the immune microenvironment in COPD patients is favorable for immunotherapy and that the more severe the COPD is, the higher ratio of CD8+ T cells, Th1 cells, and M1/M2 macrophages is associated, which is more conducive to enhancing the immune effect (19-21). As mentioned previously, in recent years, the efficacy of immunotherapy in patients with advanced NSCLC combined with COPD has been reported to be better than that in patients with non-COPD, without a significant increase in the incidence of adverse events (19-21). However, the value of immunotherapy in patients with early-stage lung cancer combined with COPD is unknown. In addition, surgical treatment is invasive and has higher safety requirements compared to immunotherapy and chemotherapy, so the efficacy and safety of neoadjuvant immunotherapy are particularly important for this group of patients.
In China, neoadjuvant immunotherapy started a little later, and a total of 57 immune neoadjuvant patients were enrolled in this study until May 2021, of whom 18 patients had combined COPD, all were male and all had history of smoking, and the mean age of patients with COPD was greater than that of the non-COPD group (64.8 vs. 58.7 years, P=0.005). Similar studies had previously shown that patients with lung cancer combined with COPD and a history of smoking were older than those without combined COPD (19,20).
Previous explorations had been done about immune monotherapy, dual immune combination treatment, and immune combination chemotherapy as neoadjuvant treatment modalities, and finally the NADIM study showed that immune combination chemotherapy had the highest ORR, DCR, and MPR rate, therefore, immune combination chemotherapy is currently the main strategy for neoadjuvant immunotherapy (9,22-26). In this study, the majority (53/57, 93%) of patients used immune-combination chemotherapy as a treatment option for neoadjuvant therapy. All patients achieved shrinkage of tumor lesions after neoadjuvant therapy, with ORR and DCR rates of 56.1% and 100%, respectively. DCR was achieved in all COPD patients and non-COPD patients, and ORR was similar in both groups (44.4% vs. 61.5%, P=0.23).
The highest MPR achieved in previous small sample immune neoadjuvant studies was that from the NADIM study and with nivolumab in combination with paclitaxel and/or carboplatin as neoadjuvant regimen, with an MPR of 83.0% (9,22-26). pCR was significantly higher in the immune combination chemotherapy group than in the chemotherapy group in the CheckMate-816 study (24.4% vs. 2.2%, P<0.0001) (9). In our study, the MPR and pCR of all patients were 54.4% and 31.6%, respectively, which are similar to the results of previous prospective clinical studies. Very good pathological response was also achieved with neoadjuvant immunotherapy in NSCLC patients with combined COPD, with MPR and pCR of 55.6% and 38.9%, respectively, compared with 53.8% and 28.2% in non-COPD patients, but the differences in MPR and pCR between the two subgroups did not reach statistical significance (P=0.90 and P=0.42, respectively).
There had been controversy in the past about whether MPR and pCR could be used as valid alternative endpoints for neoadjuvant immunotherapy, however, the CheckMate-816 study has demonstrated that MPR and pCR could translate into prolonged EFS time and are independent of PD-L1 expression status (9). We specifically updated our follow-up data 1-year after the last patient was enrolled, however, the median EFS and OS of the study remained unmet. One-year EFS and OS rates were 93.0% and 96.5%, respectively, and 2-year rates were 83.7% and 94.6%, respectively. Numerically similar to the CheckMate-816 study, however our study included a higher number of stage-IIIB patients, demonstrated the survival benefit of neoadjuvant therapy with immune combination chemotherapy again. And we also saw significantly higher 1- and 2-year EFS rates obtained in pCR patients (100% vs. 89.7%; 100% vs. 76.7%, P=0.04). One- and 2-year EFS rates appeared to be lower in the COPD patients than in non-COPD patients (88.9% vs. 94.9%; 73.7% vs. 88.2%), but the difference did not reach statistical significance (P=0.21). Previous studies had shown that in patients with advanced NSCLC combined with COPD, immunotherapy was more effective in the COPD group than in the non-COPD group (19-21). In our study, there seemed to be little difference between the two when immunotherapy was used in early-stage patients, but due to the trauma of surgery, lung function impairment, etc., whether the later EFS and OS will be affected needs to be verified by longer follow-up and more samples.
Patients with stage IIIB were not included in the previous neoadjuvant immunotherapy studies, but our study was a real-world study in which some patients with stage IIIB were evaluated for downstaging after neoadjuvant immunotherapy and had the possibility of radical resection and were therefore included. During the neoadjuvant therapy, investigators gave more courses of treatment (3–4 courses) to this group of patients. The pCR and MPR were relatively low in patients with stage IIIB (26.3% vs. 34.2%; 42.1% vs. 60.5%), but differences did not reach statistical significance (P=0.55 and P=0.12, respectively). Two-year EFS rate and OS rate did not appear to be lower than those of earlier patients (84.2% vs. 82.3%, P=0.84; 89.2% vs. 97.4%, P=0.21). This suggested that some stage IIIB patients might be considered for radical surgical resection after neoadjuvant immunotherapy if they could reach the descending stage. Related prospective clinical study is also already underway and hopefully will yield encouraging results and bring survival benefits to more patients (27).
The CheckMate-816 study evaluated surgical difficulty and complications after neoadjuvant immunotherapy and showed that ICIs had a small impact on surgical resection rates and surgical complexity, with no adverse effect on perioperative outcome (9). In our study, patients had a complete resection rate of 91.2% (52/57), and one of the patients who did not achieve complete resection was a patient with COPD. Our study also showed no significant increase in surgical difficulty, mean operative time (138.50 min) and bleeding (189.44 mL) with neoadjuvant immunotherapy. 10.5% of patients developed postoperative complications such as: chylothorax, transfusion, pneumonia, wound infection, and arrhythmias, all of which did not result in patient death. There was no difference between patients in the COPD and non-COPD groups in terms of complete resection rate (94.4% vs. 89.7%, P>0.99), VATs surgery (55.6% vs. 51.3%, P=0.76), operative time (mean, 157.50 vs. 131.59 min, P=0.23), and bleeding (mean, 226.11 vs. 171.11 mL, P=0.41). In COPD patients with already poor lung function, it was crucial that the procedure was successful and without additional associated complications.
Our data showed that the rate of complete surgical resection for NSCLC patients following neoadjuvant immunotherapy was as high as 91.2%. In the three prospective clinical studies (CheckMate-816, NADIM II, and Keynote 671) mentioned in the introduction, the R0 rates for the neoadjuvant chemotherapy groups ranged from 77.8% to 85.0% (9-11). Another prospective study comparing neoadjuvant chemoradiotherapy to neoadjuvant chemotherapy reported R0 rates of 91% and 81%, respectively (28). These results suggest that the R0 rate for neoadjuvant immunochemotherapy seems to be the highest compared to neoadjuvant chemotherapy or neoadjuvant chemoradiotherapy, but this result requires further clinical data for validation. By comparing 31 patients who completed pulmonary function tests both before and after having received neoadjuvant immunotherapy, we found an interesting phenomenon: FEV1, FEV1%pred, FVC, and FVC%pred improved significantly after receiving neoadjuvant immunotherapy, while DLCO and DLCOc decreased significantly after treatment. The indicators FEV1, FEV1%pred, FVC, and FVC%pred reflected the patient’s pulmonary ventilatory function, while the two indicators DLCO and DLCOc reflect the patient’s pulmonary diffusion function (29). This shows that immune neoadjuvant therapy improve pulmonary ventilation function but decrease pulmonary diffusion function. The possible reason for the improvement in ventilation function is that in patients with bronchopulmonary cancer, FEV1 and FEV1%pred are reduced due to local obstruction of the bronchi by the tumor, and the reduction in tumor size after neoadjuvant therapy improved pulmonary ventilation function. However, there is a potential for lung injury with immunotherapy and chemotherapy, which could lead to a significant decrease in DLCO (30). The mechanism by which chemotherapy and immunotherapy lead to lung injury is unclear. Another point worth noting was that for COPD patients, FEV1 improved after immunotherapy, but no significant changes were found in any other index, suggested that lung function in COPD patients improved less than in non-COPD patients after immunotherapy, possibly related to his own poorer lung function. However, our sample size was small and more data are needed to validate this and further basic research is needed to explore the mechanisms involved.
We analyzed the genetic test results of 39 patients who underwent NGS testing of tissue samples in this study, and found that the common mutated loci were TP53, FGFR1, CDKN2A, DPYD, KEAP1, PIK3CA, STK11, BRCA1, and BRAF. We analyzed the possible relationship between each of these mutated loci and ORR, MPR, pCR, EFS, and OS, and the results showed that, among these mutations, CDKN2A deletion was correlated with pathological response and survival time. In this study, none of the six patients with CDKN2A deletion obtained MPR and pCR, and the presence of CDKN2A deletion seemed to be associated with shorter EFS and OS times. CDKN2A is an oncogene located on chromosome 9 and the most common somatic mutated gene in metastatic tumors, and is frequently absent, mutated or hypermethylated in many tumors, leading to cell growth and uncontrolled proliferation, carcinogenesis, and is considered the second most frequently inactivated gene in cancer after p53 (31). Previous studies had shown that the OS of NSCLC patients with high CDKN2A expression was significantly lower than that of the low expression group [hazard ratio (HR) =1.3; P=0.02] (32). And whether there is some correlation between the presence of CDKN2A mutation and the efficacy of immunotherapy needs to be explored by larger sample size or even more basic studies.
There are some limitations in this study. Firstly, the sample size of the study was small, which may be related to the fact that the study was a retrospective one conducted shortly after the introduction of neoadjuvant immunotherapy in China and that the population eligible for neoadjuvant immunotherapy was relatively small. Secondly, the COPD group exhibited imbalanced baseline characteristics, such as a higher proportion of smokers, males, elderly individuals and squamous cell types, which might be a confounding factor. However, due to the small sample size, we were unable to conduct multivariate analysis. Thirdly, although the median follow-up time was 454 days, the median EFS and OS times had still not been reached. Finally, the exact cause of the effect of neoadjuvant therapy on lung function is still unknown. Therefore, we will continue to conduct long-term follow-up in more patients and conduct some mechanistic explorations to answer the above questions.
Conclusions
This study reported the efficacy and safety of neoadjuvant immunotherapy in patients with resectable NSCLC in the real world, and provided the first targeted analysis of the outcomes associated with neoadjuvant immunotherapy in NSCLC patients with COPD, which is of good clinical reference.
Acknowledgments
We thank the patients and their families for their contributions to our study.
Funding: The study was supported by
Footnote
Reporting Checklist: The authors have completed the STROBE reporting checklist. Available at https://jtd.amegroups.com/article/view/10.21037/jtd-24-811/rc
Data Sharing Statement: Available at https://jtd.amegroups.com/article/view/10.21037/jtd-24-811/dss
Peer Review File: Available at https://jtd.amegroups.com/article/view/10.21037/jtd-24-811/prf
Conflicts of Interest: All authors have completed the ICMJE uniform disclosure form (available at https://jtd.amegroups.com/article/view/10.21037/jtd-24-811/coif). The authors have no conflicts of interest to declare.
Ethical Statement: The authors are accountable for all aspects of the work in ensuring that questions related to the accuracy or integrity of any part of the work are appropriately investigated and resolved. The study was conducted in accordance with the Declaration of Helsinki (as revised in 2013). The study was approved by the independent ethics committee of Shanghai Chest Hospital (No. KS22002) and informed consent was obtained from all individual participants.
Open Access Statement: This is an Open Access article distributed in accordance with the Creative Commons Attribution-NonCommercial-NoDerivs 4.0 International License (CC BY-NC-ND 4.0), which permits the non-commercial replication and distribution of the article with the strict proviso that no changes or edits are made and the original work is properly cited (including links to both the formal publication through the relevant DOI and the license). See: https://creativecommons.org/licenses/by-nc-nd/4.0/.
References
- Siegel RL, Miller KD, Jemal A. Cancer statistics, 2020. CA Cancer J Clin 2020;70:7-30. [Crossref] [PubMed]
- Chen W, Zheng R, Baade PD, et al. Cancer statistics in China, 2015. CA Cancer J Clin 2016;66:115-32. [Crossref] [PubMed]
- Bray F, Ferlay J, Soerjomataram I, et al. Global cancer statistics 2018: GLOBOCAN estimates of incidence and mortality worldwide for 36 cancers in 185 countries. CA Cancer J Clin 2018;68:394-424. [Crossref] [PubMed]
- Goldstraw P, Chansky K, Crowley J, et al. The IASLC Lung Cancer Staging Project: Proposals for Revision of the TNM Stage Groupings in the Forthcoming (Eighth) Edition of the TNM Classification for Lung Cancer. J Thorac Oncol 2016;11:39-51. [Crossref] [PubMed]
- Bagcchi S. Lung cancer survival only increases by a small amount despite recent treatment advances. Lancet Respir Med 2017;5:169. [Crossref] [PubMed]
- Liang W, Cai K, Chen C, et al. Expert consensus on neoadjuvant immunotherapy for non-small cell lung cancer. Transl Lung Cancer Res 2020;9:2696-715. [Crossref] [PubMed]
- Chen S, Zhao Z, Long H. Application of Neoadjuvant Immuno-chemotherapy in NSCLC. Zhongguo Fei Ai Za Zhi 2021;24:284-92. [Crossref] [PubMed]
- Friedlaender A, Naidoo J, Banna GL, et al. Role and impact of immune checkpoint inhibitors in neoadjuvant treatment for NSCLC. Cancer Treat Rev 2022;104:102350. [Crossref] [PubMed]
- Forde PM, Spicer J, Lu S, et al. Neoadjuvant Nivolumab plus Chemotherapy in Resectable Lung Cancer. N Engl J Med 2022;386:1973-85. [Crossref] [PubMed]
- Provencio M, Nadal E, González-Larriba JL, et al. Perioperative Nivolumab and Chemotherapy in Stage III Non-Small-Cell Lung Cancer. N Engl J Med 2023;389:504-13. [Crossref] [PubMed]
- Wakelee H, Liberman M, Kato T, et al. Perioperative Pembrolizumab for Early-Stage Non-Small-Cell Lung Cancer. N Engl J Med 2023;389:491-503. [Crossref] [PubMed]
- Loganathan RS, Stover DE, Shi W, et al. Prevalence of COPD in women compared to men around the time of diagnosis of primary lung cancer. Chest 2006;129:1305-12. [Crossref] [PubMed]
- Abal Arca J, Parente Lamelas I, Almazán Ortega R, et al. Lung cancer and COPD: a common combination. Arch Bronconeumol 2009;45:502-7. [Crossref] [PubMed]
- Henschke CI, Yip R, Boffetta P, et al. CT screening for lung cancer: Importance of emphysema for never smokers and smokers. Lung Cancer 2015;88:42-7. [Crossref] [PubMed]
- Butler SJ, Ellerton L, Goldstein RS, et al. Prevalence of lung cancer in chronic obstructive pulmonary disease: A systematic review. Respir Med X 2019;1:100003.
- Vogelmeier CF, Criner GJ, Martinez FJ, et al. Global Strategy for the Diagnosis, Management, and Prevention of Chronic Obstructive Lung Disease 2017 Report. GOLD Executive Summary. Am J Respir Crit Care Med 2017;195:557-82. [Crossref] [PubMed]
- Eisenhauer EA, Therasse P, Bogaerts J, et al. New response evaluation criteria in solid tumours: revised RECIST guideline (version 1.1). Eur J Cancer 2009;45:228-47. [Crossref] [PubMed]
- Expert consensus on the pathological evaluation of neoadjuvant therapy efficacy for non-small cell lung cancer. Zhonghua Bing Li Xue Za Zhi 2021;50:1002-7. [Crossref] [PubMed]
- Zhou J, Chao Y, Yao D, et al. Impact of chronic obstructive pulmonary disease on immune checkpoint inhibitor efficacy in advanced lung cancer and the potential prognostic factors. Transl Lung Cancer Res 2021;10:2148-62. [Crossref] [PubMed]
- Shin SH, Park HY, Im Y, et al. Improved treatment outcome of pembrolizumab in patients with nonsmall cell lung cancer and chronic obstructive pulmonary disease. Int J Cancer 2019;145:2433-9. [Crossref] [PubMed]
- Mark NM, Kargl J, Busch SE, et al. Chronic Obstructive Pulmonary Disease Alters Immune Cell Composition and Immune Checkpoint Inhibitor Efficacy in Non-Small Cell Lung Cancer. Am J Respir Crit Care Med 2018;197:325-36. [Crossref] [PubMed]
- Forde PM, Chaft JE, Smith KN, et al. Neoadjuvant PD-1 Blockade in Resectable Lung Cancer. N Engl J Med 2018;378:1976-86. [Crossref] [PubMed]
- Hui R, Özgüroğlu M, Villegas A, et al. Patient-reported outcomes with durvalumab after chemoradiotherapy in stage III, unresectable non-small-cell lung cancer (PACIFIC): a randomised, controlled, phase 3 study. Lancet Oncol 2019;20:1670-80. [Crossref] [PubMed]
- Cascone T, William WN Jr, Weissferdt A, et al. Neoadjuvant nivolumab or nivolumab plus ipilimumab in operable non-small cell lung cancer: the phase 2 randomized NEOSTAR trial. Nat Med 2021;27:504-14. [Crossref] [PubMed]
- Provencio M, Nadal E, Insa A, et al. Neoadjuvant chemotherapy and nivolumab in resectable non-small-cell lung cancer (NADIM): an open-label, multicentre, single-arm, phase 2 trial. Lancet Oncol 2020;21:1413-22. [Crossref] [PubMed]
- Peters S, Kim AW, Solomon B, et al. IMpower030: Phase III study evaluating neoadjuvant treatment of resectable stage II-IIIB non-small cell lung cancer (NSCLC) with atezolizumab (atezo)+ chemotherapy. Ann Oncol 2019;30:ii30.
- Qiu B, Cai K, Chen C, et al. Expert consensus on perioperative immunotherapy for local advanced non-small cell lung cancer. Transl Lung Cancer Res 2021;10:3713-36. [Crossref] [PubMed]
- Pless M, Stupp R, Ris HB, et al. Induction chemoradiation in stage IIIA/N2 non-small-cell lung cancer: a phase 3 randomised trial. Lancet 2015;386:1049-56. [Crossref] [PubMed]
- Brunelli A, Refai MA, Salati M, et al. Carbon monoxide lung diffusion capacity improves risk stratification in patients without airflow limitation: evidence for systematic measurement before lung resection. Eur J Cardiothorac Surg 2006;29:567-70. [Crossref] [PubMed]
- Zhu Y, Li JQ, Chang Q, et al. Impact of neoadjuvant immunotherapy on pulmonary function and perioperative outcomes in patients with resectable non-small cell lung cancer. Zhonghua Yi Xue Za Zhi 2022;102:393-8. [Crossref] [PubMed]
- Toyokuni S. Mysterious link between iron overload and CDKN2A/2B. J Clin Biochem Nutr 2011;48:46-9. [Crossref] [PubMed]
- Fang H. Bioinformatics analysis of CDKN2A gene expression and biological function in NSCLC. Journal of Clinical Pulmonary Medicine 2020;25:196-200.