Comparison of watershed analysis with indocyanine green fluorescence staining and modified inflation-deflation method in single-port thoracoscopic complex pulmonary segmentectomy
Highlight box
Key findings
• The watershed analysis with indocyanine green (ICG) fluorescence staining is associated with reduced operating time and fewer postoperative complications in complex pulmonary segmentectomy.
What is known and what is new?
• Arterial-ligation-only method effectively, accurately, and inexpensively identifies intersegmental planes (ISPs) in simple pulmonary segmentectomy.
• This study aims to compare the short-term clinical safety and efficacy of the watershed analysis with ICG fluorescence staining with the modified inflation-deflation method in single-port thoracoscopic complex pulmonary segmentectomy. These satisfactory results introduce a more promising approach for complex pulmonary segmentectomy.
What is the implication, and what should change now?
• For complex pulmonary segmentectomy, watershed analysis with ICG fluorescence staining demonstrates superior short-term clinical safety and efficacy. Further detailed studies on specific pulmonary segmentectomies are warranted.
Introduction
Lung cancer stands out as the most frequently diagnosed cancer and the primary cause of cancer-related deaths globally. As per the GLOBOCAN 2022 data, an estimated 2.48 million new cases of lung cancer (12.4%) and nearly 1.8 million lung cancer deaths (18.7%) were reported in 2022 (1). Lung cancer exceeds breast cancer, prostate cancer, and colorectal cancer combined in terms of mortality rates (2). The widespread adoption of computed tomography (CT) scans and increased health awareness have led to a notable upsurge in early lung cancer detection. The high resolution and accuracy of CT scans make it easier to detect early and tiny pulmonary nodules, a considerable part of which requires surgical intervention. This progress significantly enhances the survival rate of patients with lung cancer (3).
Historically, pulmonary lobectomy has been the gold standard procedure for lung cancer surgery due to its efficacy in completely removing tumors and adjacent lymph nodes, thereby reducing the risk of recurrence. However, findings from the JCOG0802/WJOG4607L study suggest that the 5-year overall survival rate of patients undergoing pulmonary segmentectomy is comparable to, and in some aspects may even surpass, that of patients with pulmonary lobectomy (4). Consequently, pulmonary segmentectomy is increasingly recognized as a viable alternative for non-small cell lung cancer (NSCLC) situated peripherally and measuring 2 cm or less. It is gradually establishing itself as the standard procedure (4,5). The key to successful pulmonary segmentectomy lies in accurately identifying tumors and intersegmental planes (ISPs) to ensure adequate resection margins (6). Various techniques have been developed to highlight target segments or emphasize preserved areas for the exposure of ISPs (7). The modified inflation-deflation method is widely accepted as a fundamental approach for visualizing ISPs. Yao et al. demonstrated its effectiveness in both complex and simple pulmonary segmentectomy groups by evaluating its success rate and demarcation clarity (8). Additionally, the watershed analysis with indocyanine green (ICG) fluorescence staining, which involves intravenous injection of ICG under near-infrared (NIR) light to visualize ISPs, has been employed when interrupting blood flow to the target pulmonary segment by ligating related vessels. Sun et al. confirmed consistent and matched ISPs in segmentectomy using both the modified inflation-deflation method and the watershed analysis with ICG fluorescence staining (9). Moreover, Xie et al. noted that the watershed analysis with ICG fluorescence staining reduced operating time and maintained similar perioperative parameters and postoperative pulmonary function in cases with simple pulmonary segmentectomy (10). However, the clinical outcomes of these methods in single-port thoracoscopic complex pulmonary segmentectomy remain insufficiently explored. This study assesses the clinical efficacy and safety of two techniques in uniportal thoracoscopic complex pulmonary segmentectomy by analyzing short-term outcomes, such as operative time, intraoperative blood loss, postoperative air leaks, drainage volume, and reduction in pulmonary function. By comparing these techniques with the conventional modified inflation-deflation method, we aim to identify a more effective and promising approach for identification of ISPs in single-port thoracoscopic complex pulmonary segmentectomy. We present this article in accordance with the STROBE reporting checklist (available at https://jtd.amegroups.com/article/view/10.21037/jtd-24-1075/rc).
Methods
Study population
The study was conducted in accordance with the Declaration of Helsinki (as revised in 2013), and received approval from the Institutional Review Board of The First Affiliated Hospital of Soochow University (Ethics Approval No. 2024186). Individual consent for this retrospective analysis was waived. It was a retrospective, single-center pilot study that collected clinical data from 101 cases of single-port thoracoscopic complex pulmonary segmentectomy conducted at The First Affiliated Hospital of Soochow University between January 2023 and December 2023. Inclusion criteria encompassed patients aged 18 years or older with CT-confirmed pulmonary nodules smaller than 20 mm who underwent single-port thoracoscopic complex pulmonary segmentectomy based on preoperative reconstructed three-dimensional (3D) images. Exclusion criteria included: (I) a history of prior lung surgery; (II) preoperative assessments suggesting inadequate tumor resection margins with pulmonary segmentectomy; (III) intraoperative frozen section biopsy confirmation necessitating further pulmonary lobectomy; (IV) patients deemed unsuitable for surgery due to coexisting malignancies or major organ dysfunction, such as heart, liver, or kidney diseases. Among these cases in single-port thoracoscopic complex pulmonary segmentectomy, 50 patients underwent the watershed analysis with ICG fluorescence staining, while 51 patients utilized the modified inflation-deflation method (Figure 1). All 101 patients in this study underwent surgery performed by the same senior surgeon. The complex pulmonary segmentectomy procedure targeted specific segments, including the right 1st, 2nd, 3rd, 7th, 8th, 9th, and 10th segments, as well as the left 1–2th, 3rd, 8th, 9th, and 10th segments (11,12). Moreover, cases involving combined segmentectomy, combined subsegmentectomy, and combined segmentectomy with subsegmentectomy were included (12). Data on patient demographics, nodule characteristics, intraoperative parameters, and postoperative conditions were documented. Follow-up assessments were conducted regularly for more than 3 months, and the results of postoperative pulmonary function tests were recorded. This study evaluates the clinical efficacy of the two groups by comparing short-term outcomes such as operative time, postoperative air leak incidence, and postoperative drainage volume. While the watershed analysis with ICG fluorescence staining theoretically simplifies the surgical procedure, supporting evidence from related studies is necessary.
Preoperative planning
Before surgery, patients underwent standard thin-slice chest CT scans. The acquired data were then processed through three-dimensional reconstruction using InferOperate Thorax Planning (Infervision Medical Technology Co., Ltd., Beijing, China). This process was designed to pinpoint the relevant arteries, veins, and bronchus linked to the targeted pulmonary segment, facilitating a thorough evaluation of the surgical approach and pathway.
Video-assisted uniportal thoracoscopic surgery procedure
All patients underwent surgery performed by the same senior surgeon. They were positioned in the lateral decubitus posture, and the procedure was conducted under general intravenous anesthesia with single-lung ventilation applied to the healthy side. An approximately 3 cm incision was made near the anterior axillary line at the fourth or fifth intercostal space. Following preoperative planning, precise dissection of the relevant target segment pulmonary arteries, veins, and bronchus was performed, and the ISPs was delineated using two methods. Then, dissected ISPs were processed using a stapler, followed by frozen section biopsy of tumors. Intraoperative lymph node dissection was guided by the intraoperative anatomy of pertinent vessels and bronchus, along with findings from frozen section biopsy. Following routine thoracic lavage, lung inflation was assessed for air leakage, and any detected leakage were sutured. Subsequently, a chest tube was inserted through the operating port. Postoperative monitoring entailed observing chest tube drainage and checking for air leakage. If lung re-expansion following surgery was satisfactory, with no air leakage and drainage volume below 100 mL/day, the drainage tube could be removed, allowing for discharge as early as the second day.
Modified inflation-deflation method
After disconnecting the target segment’s pulmonary arteries, veins, and bronchus, both lungs were ventilated with pure oxygen, with bronchial pressure maintained at 20 cmH2O. Once the operated lung was fully inflated, ventilation transitioned to single-lung ventilation on the healthy side. Approximately 15 to 20 minutes later, the monitor showed the inflated target segment that required excision, while the remaining lung appeared dark red (Figure 2).
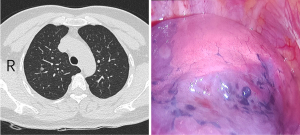
Watershed analysis with ICG fluorescence staining
When the related pulmonary arteries, pulmonary veins, or both vessels of the target pulmonary segment were obstructed, a 2 mL intravenous injection of ICG (25 mg; Dandong Yichuang Pharmaceutical Co., Ltd., China) was administered. After 5 to 10 seconds, the fluorescence mode on the VisionPro 26 LED Display (Stryker Endoscopy, USA) showed the target segment to be excised as colorless, while the preserved segments appeared green (Figure 3). When the pulmonary artery of the target segment was blocked, it hindered blood flow to the specific area. Consequently, after injecting ICG, no contrast enhancement was observed in this region in several seconds. This facilitates the precise localization of pulmonary nodules within this segment. In cases where pulmonary nodules are positioned either near the segmental or subsegmental veins, or at the junction of segments and subsegments, surgeons opted to temporarily occlude the pulmonary veins during surgery for impeding blood reflux into the target region. Due to the increased pressure in the distal end of the pulmonary artery, the entry of ICG from the peripheral blood into this region occurred slowly, resulting in a temporary absence of contrast enhancement in the target segment.
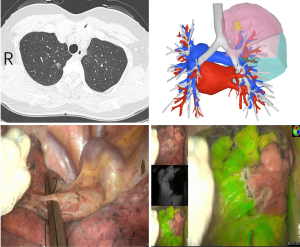
Statistical analysis
This study utilized SPSS software (version 26.0, Chicago, IL, USA) for statistical analysis. Continuous variables were analyzed using either the t-test or Mann-Whitney U test, while categorical variables were assessed employing the Chi-squared test or Fisher’s exact test. Statistical significance was set at a P value <0.05.
Results
Characteristics of the patients
A total of 101 patients underwent complex pulmonary segmentectomy via uniportal thoracoscopy in this research. Among them, 50 patients used watershed analysis with ICG fluorescence staining, and 51 patients used the modified inflation-deflation method. The clinical characteristics of the patients and pulmonary nodules are summarized in Table 1. Watershed analysis with ICG fluorescence staining group had an average age of 57.1±13.8 years with 20 males and 30 females. Four patients had a history of smoking, and 46 did not. In the modified inflation-deflation method group, there were 20 males and 31 females, with an average age of 54.5±11.9 years. Seven patients had a history of smoking, and 44 did not. The mentioned factors exhibited no statistically significant differences between the two cohorts in age (P=0.31), gender (P=0.94) and smoking history (P=0.36). The average distance from the pulmonary nodules to the pleural surface was 17.9±2.1 mm in the watershed analysis with ICG fluorescence staining group and 17.5±2.0 mm in the modified inflation-deflation method group, with no statistically significant difference (P=0.37). Similarly, the average size of the pulmonary nodules was 12.7±3.6 mm in the former group and 12.2±2.6 mm in the latter group, showing no statistically significant difference (P=0.49). The distribution of pulmonary nodule locations was not significantly different between the two groups (P=0.22). In the watershed analysis with ICG fluorescence staining group, 18 pulmonary nodules were observed in the left upper lobe, 3 in the left lower lobe, 25 in the right upper lobe, and 4 in the right lower lobe. Similarly, in the modified inflation-deflation group, 17 pulmonary nodules were located in the left upper lobe, 7 in the left lower lobe, 25 in the right upper lobe, and 2 in the right lower lobe. Detailed segmental information is provided in the Tables S1-S4.
Table 1
Variables | Watershed analysis with ICG fluorescence staining | Modified inflation-deflation method | P value |
---|---|---|---|
Total | 50 | 51 | |
Age (years) | 57.1±13.8 | 54.5±11.9 | 0.31 |
Gender | 0.94 | ||
Male | 20 [40] | 20 [39] | |
Female | 30 [60] | 31 [61] | |
Smoking history | 0.36 | ||
Yes | 4 [8] | 7 [14] | |
No | 46 [92] | 44 [86] | |
Nodule size (mm) | 12.7±3.6 | 12.2±2.6 | 0.49 |
Nodule depth (mm) | 17.9±2.1 | 17.5±2.0 | 0.37 |
Nodule location | 0.22 | ||
LUL | 18 [36] | 17 [34] | |
LLL | 3 [6] | 7 [14] | |
RUL | 25 [50] | 25 [49] | |
RLL | 4 [8] | 2 [3] |
Data are presented as n, mean ± SD, or n [%]. ICG, indocyanine green; LUL, left upper lobe; LLL, left lower lobe; RUL, right upper lobe; RLL, right lower lobe; SD, standard deviation.
Intraoperative and postoperative conditions
The detailed perioperative conditions of the patients are presented in Table 2. The surgical duration for the group utilizing watershed analysis with ICG fluorescence staining was 93.5±50.7 minutes, whereas it was 160.8±54.4 minutes for the modified inflation-deflation method group, highlighting significant differences between the two groups (P<0.001). Intraoperative visualization of the ISPs took much more time in the modified inflation-deflation method group (1,187.9±247.9 seconds) compared to the watershed analysis with ICG fluorescence staining group (10.3±2.0 seconds), demonstrating notable differences (P<0.001). Blood loss during surgery amounted to 26.5±36.0 mL in the watershed analysis with ICG fluorescence staining group and 54.3±39.3 mL in the modified inflation-deflation method group, indicating significant differences between the two groups (P<0.001). The number of lymph nodes removed did not show statistical differences between the two groups, with 0.8±0.9 for the watershed analysis with ICG fluorescence staining and 1.0±0.9 for the modified inflation-deflation method group (P=0.20). The concurrent use of the watershed analysis and ICG fluorescence staining did not lead to any complications associated with the latter. All surgical procedures were successfully performed, resulting in the complete excision of all pulmonary nodules.
Table 2
Variables | Watershed analysis with ICG fluorescence staining | Modified inflation-deflation method | P value |
---|---|---|---|
Total | 50 | 51 | |
Operating time (minutes) | 93.5±50.7 | 160.8±54.4 | <0.001** |
Intersegmental plane visualization time (seconds) | 10.3±2.0 | 1,187.9±247.9 | <0.001** |
Intraoperative bleeding volume (mL) | 26.5±36.0 | 54.3±39.3 | <0.001** |
Number of lymph nodes removed | 0.8±0.9 | 1.0±0.9 | 0.20 |
Postoperative hospitalization (days) | 3.8±1.4 | 6.2±3.4 | <0.001** |
Postoperative drainage time (days) | 2.7±1.4 | 5.0±3.2 | <0.001** |
Air leakage | 0.009* | ||
Yes | 8 [16] | 20 [39] | |
No | 42 [84] | 31 [61] | |
Pleural effusion volume (mL) | 230.5±204.9 | 442.4±272.6 | <0.001** |
Pathology | 0.39 | ||
AIS | 5 [10] | 7 [14] | |
MIA | 23 [46] | 26 [51] | |
IAC | 13 [26] | 10 [20] | |
Benign | 9 [18] | 8 [15] |
Data are presented as n, mean ± SD, or n [%]. *, P<0.01; **, P<0.001. ICG, indocyanine green; AIS, adenocarcinoma in situ; MIA, microinvasive adenocarcinoma; IAC, invasive adenocarcinoma; SD, standard deviation.
The postoperative hospitalization was 3.8±1.4 days for the watershed analysis with ICG fluorescence staining group and 6.2±3.4 days for the modified inflation-deflation method group, with significant intergroup differences (P<0.001). Postoperative drainage tube placement lasted 2.7±1.4 days for the watershed analysis with ICG fluorescence staining group, resulting in a total postoperative chest tube drainage of 230.5±204.9 mL. Eight patients (16%) experienced postoperative air leakage. In contrast, the modified inflation-deflation group had a postoperative drainage tube placement duration of 5.0±3.2 days, accompanied by a total chest tube drainage of 442.4±272.6 mL. Twenty patients (39%) experienced postoperative air leakage. Statistically significant differences were observed between the two groups regarding postoperative drainage tube placement duration (P<0.001), drainage volume (P<0.001), and postoperative air leakage rates (P=0.009). Pathological analysis of the watershed analysis with ICG fluorescence staining group showed 9 cases (18%) of benign lesions, 5 case (10%) of in situ adenocarcinoma, 23 cases (46%) of microinvasive adenocarcinoma, and 13 cases (26%) of invasive adenocarcinoma. Besides, postoperative pathology in the modified inflation-deflation method group revealed 8 cases (15%) of benign lesions, 7 cases (14%) of in situ adenocarcinoma, 26 cases (51%) of microinvasive adenocarcinoma, and 10 cases (20%) of invasive adenocarcinoma.
Postoperative short-term effects on pulmonary function
Two cohorts of patients underwent complex pulmonary segmentectomy based on ranges of identified ISPs during surgery. Preoperatively, the forced expiratory volume in one second (FEV1) for the watershed analysis with ICG fluorescence staining group was 2.61±0.67 L, whereas for the modified inflation-deflation method group, it was 2.59±0.64 L, showing no significant differences between the groups (P=0.83). At the three-month postoperative examination, the postoperative FEV1 for the watershed analysis with ICG fluorescence staining group was 2.10±0.54 L, and for the modified inflation-deflation method group, it was 2.08±0.52 L, with no significant differences observed between the groups (P=0.86). The postoperative decrease in FEV1 compared to preoperative values was not statistically significant in either group (P=0.89), with a reduction of 19.68%±3.59% for the watershed analysis with ICG fluorescence staining group and 19.58%±3.31% for the modified inflation-deflation method group. Preoperatively, the forced vital capacity (FVC) for the watershed analysis with ICG fluorescence staining group was 3.04±0.78 L, and postoperatively it was 2.51±0.65 L, representing an FVC decrease of 17.28%±2.21%. For the modified inflation-deflation method group, the preoperative FVC was 3.01±0.75 L, and postoperatively it was 2.51±0.62 L, with a postoperative decrease of 16.73%±2.47%. Similarly, there were no significant differences in preoperative FVC value (P=0.86), postoperative FVC values (P=0.95) and decreases (P=0.54) between the groups, indicating similar short-term pulmonary function impairments. Data of preoperative and postoperative pulmonary function for the two groups are summarized in Table 3.
Table 3
Variables | Watershed analysis with ICG fluorescence staining | Modified inflation-deflation method | P value |
---|---|---|---|
Preoperative pulmonary function | |||
FEV1 (L) | 2.61±0.67 | 2.59±0.64 | 0.83 |
FVC (L) | 3.04±0.78 | 3.01±0.75 | 0.86 |
Postoperative pulmonary function | |||
FEV1 (L) | 2.10±0.54 | 2.08±0.52 | 0.86 |
FVC (L) | 2.51±0.65 | 2.51±0.62 | 0.95 |
Loss of pulmonary function | |||
FEV1 (%) | 19.68±3.59 | 19.58±3.31 | 0.89 |
FVC (%) | 17.28±2.21 | 16.73±2.47 | 0.54 |
Data are presented as mean ± SD. ICG, indocyanine green; FEV1, forced expiratory volume in 1 second; FVC, forced vital capacity; SD, standard deviation.
Discussion
Lung cancer currently has the highest incidence and mortality rates among malignant tumors worldwide (1). The widespread use of high-resolution CT scans has increased the detection of pulmonary nodules, resulting in more patients undergoing surgical treatment. Recent studies have demonstrated pulmonary segmentectomy as a more advantageous option for peripherally located NSCLC measuring ≤2 cm (4,5). Weiss et al. reported that approximately 20% of pulmonary segmentectomies do not meet resection standards, emphasizing the necessity for stricter operational standards and review protocols (13).
The success of segmentectomy relies on accurately identifying and isolating critical blood vessels and bronchus, as well as clearly delineating ISPs. In cases where intersegmental fissures are well-developed, such as in the dorsal, lingual, or apical segments, natural anatomical structures can be used for identification of ISPs. Preoperative thin-slice CT scans that reveal the excellent presence of the intersegmental fissure in the target segment facilitate easier intraoperative identification. Currently, the modified inflation-deflation method is widely employed. After isolating the target segment’s artery, veins and bronchus, the entire lung is ventilated with pure oxygen under positive pressure, causing the target segment to fully inflate through Kohn’s pores. Ventilation to the operated side is then ceased, preventing air from escaping the target segment, which remains inflated and delineates the boundary with the collapsed adjacent segments. However, this method extends surgical time. Yang et al. reported 75% nitrous oxide with 25% oxygen ventilation could reduce the visualization time of the ISPs (14). The modified inflation-deflation method is straightforward and requires no dyes or special equipment. Misidentification and incorrect transection of target segment structures during surgery can compromise the display of the ISPs. Additionally, in patients with severe emphysema or pulmonary fibrosis, the interface delineation can be inaccurate. Post-inflation of the target segment can also reduce the operative space for thoracoscopic procedures, complicating specimen retrieval through minimally invasive incisions.
ICG is now extensively used for intraoperative identification of ISPs. Following the isolation of relevant target segmental vessels, peripheral injection of ICG prevents it from entering the target segment, causing the preserved lung to appear green while the target segment remains white. Another approach involves direct injection of ICG into the target segmental artery for immediate visualization. Additionally, ICG can be administered via a bronchoscope or directly into the isolated bronchus of the target segment, although this is significantly affected by bronchial obstruction and mucus (15). Using ICG to delineate ISPs can significantly reduce surgical time, but it is not suitable for patients with poor liver function or allergies to iodine or ICG (16,17). A systematic review of 18 studies, 11 of which reported potential adverse reactions to ICG, found no adverse reactions across any of the studies, irrespective of ICG dosage (18). The review also indicated that intravenous ICG injection successfully identified ISP in 94% of cases, with identification rates ranging from 30% to 100% (18). Notably, there was considerable variability in the administered ICG doses, which ranged from 5 to 25 mg or 0.05 to 0.5 mg/kg (18). Low-dose ICG appears to effectively identify ISP while minimizing the risks of anaphylactic shock and pharmacological toxicity. Consequently, ICG is generally considered safe. Misaki et al. reported that ICG successfully visualized the ISPs in only 30% of cases. This result was associated with a high prevalence of smokers and individuals with low FEV1 within the study cohort (19). Iizuka et al. demonstrated that a smoking index (SI) greater than 800 and a low attenuation area (LAA) greater than 1% on CT could predict insufficient ICG visualization of the ISPs, which is linked to impaired perfusion in emphysema patients (20). Moreover, ICG visualization is significantly more challenging in patients with extensive anthracosis and rapid washout of ICG (18). It is important to consider the constrained applicability of ICG within the imaging ISPs under the specified exceptional conditions.
Previous studies have confirmed the efficacy of watershed analysis with arterial ligation in pulmonary simple segmentectomy. Fu et al. conducted a retrospective review of 104 patients and concluded that the arterial-ligation-only method effectively, accurately, and inexpensively identifies ISPs, particularly in patients with difficult-to-anatomize veins or bronchi (21). He et al. reported that the watershed analysis with arterial ligation method yields similar results in identifying ISPs as the ICG fluorescence method in simple segmentectomy (22). Pulmonary segmentectomy is traditionally categorized as either “simple” or “complex” based on the number of ISPs dissected (23). Recent studies have indicated that complex pulmonary segmentectomy targets specific segments, including the right 1st, 2nd, 3rd, 7th, 8th, 9th, and 10th, and the left 1-2nd, 3rd, 8th, 9th, and 10th segments (11,12). Additionally, cases involving combined segmentectomy, combined subsegmentectomy, and combined segmentectomy with subsegmentectomy have been included (12). Complex pulmonary segmentectomy can be safely performed under the guidance of three-dimensional reconstruction and does not increase the risk of postoperative complications when the ISPs are accurately identified and dissected (24). Kong et al. reviewed the records of 30 patients who underwent uniportal video-assisted thoracoscopic complex pulmonary segmentectomy and found it to be safe and effective for treating lung cancers, preserving more pulmonary parenchyma, and ensuring safe margins (25). Yao et al. reported that the modified inflation-deflation method is simple, safe, and effective in video-assisted thoracic surgery (VATS) segmentectomy and is well-suited for complex pulmonary segmentectomy (8). Okubo et al. evaluated patients undergoing simple or complex pulmonary segmentectomy for primary lung cancer and found that the perioperative outcomes of complex pulmonary segmentectomy were satisfactory and comparable to those of simple segmentectomy (26). Therefore, this article further examines the clinical safety and efficacy of watershed analysis with ICG fluorescence staining in complex pulmonary segmentectomy, comparing it to the modified inflation-deflation method.
In our study, the watershed analysis with ICG fluorescence staining group significantly reduced surgery time compared to the modified inflation-deflation method group (P<0.001). This watershed analysis required only the exposure and ligation of specific arteries or veins identified through preoperative three-dimensional reconstruction, thereby decreasing the time needed to identify and handle other vascular and bronchial structures. Additionally, the use of ICG substantially shortened the time required for the exposure of ISPs. Our findings indicated that intraoperative blood loss in the watershed analysis with ICG fluorescence staining group was less than in the modified inflation-deflation group (P<0.001). The watershed analysis’s simplification, which avoids isolating and handling other arteries, veins, bronchus, and surrounding structures, contributed to the reduced bleeding. Furthermore, due to the deep location of the bronchus and the presence of lymph nodes around some bronchial and vascular areas, bronchial exposure often risks damaging surrounding vessels. The watershed analysis, which does not involve bronchial dissection, mitigates these risks, resulting in significantly less intraoperative bleeding compared to the inflation-deflation method group.
Our results indicated that patients undergoing the watershed analysis with ICG fluorescence staining experienced significantly less postoperative air leakage compared to those treated with the modified inflation-deflation method (P=0.009). This reduction may be attributed to the watershed analysis’s less invasive nature and simplification of surgical procedure, thereby mitigating visceral pleural damage. Recent studies have identified several factors contributing to prolonged air leaks, including upper lobectomy, advanced age, reduced FEV1, low body mass index (BMI), pleural adhesions, smoking history, preoperative steroid use, non-fissureless techniques, pathological TNM stage III/IV, and the surgeon’s technical proficiency (27,28). These risk factors can be classified into patient status, intraoperative procedures, and postoperative management. Intraoperative predictors include pleural adhesions, incomplete fissures, and surgical strategy. Ponholzer et al. demonstrated that increased mobilization and frequent blunt dissection, leading to visceral pleural injury, significantly contributed to postoperative air leaks (29). Junko et al. found a correlation between the number of automatic staplers used and the incidence of intraoperative air leaks (30). Similarly, Zheng et al. reported that longer stapling lengths were associated with higher rates of postoperative air leaks (28). Furthermore, patients in the watershed analysis group had significantly less postoperative drainage time (P<0.001) and hospitalization (P<0.001). In contrast, patients in the modified inflation-deflation group exhibited greater postoperative pleural effusion volume (P<0.001). This was partly due to the higher incidence of postoperative air leakage and longer postoperative tube placement.
Our study showed no significant differences in postoperative FEV1 values (P=0.86) and FVC results (P=0.95) and the loss of pulmonary function (P=0.89 in FEV1 decline and P=0.542 in FVC decline) during the three-month postoperative follow-up between the two groups. This suggests that the exposure of ISPs achieved through watershed analysis is comparable to that achieved with the conventional modified inflation-deflation method, with similar ranges of pulmonary segmentectomy. He et al. demonstrated the equivalence of ISPs identified using watershed analysis and those identified using ICG fluorescence staining (22). It is crucial to emphasize that any differences in pulmonary function between the two groups require larger sample sizes and longer-term follow-up for further validation.
There are some limitations in this study. Firstly, it is a retrospective analysis conducted at a single center, highlighting the necessity for larger sample sizes and prospective studies across multiple centers. Secondly, this study evaluates the clinical efficacy of watershed analysis with ICG fluorescence staining in complex pulmonary segmentectomy. The location of the pulmonary nodules and the distribution of specific pulmonary segments influence the experimental outcomes. As a preliminary investigation with a limited sample size and short duration, this study highlights the need for more comprehensive research on related pulmonary segment subgroups, including challenging segments such as S9 and combined segments and subsegments, to further validate the advantages and safety of the watershed analysis method. Thirdly, longer follow-up periods are necessary to assess the long-term prognostic implications such as pulmonary function.
Conclusions
In summary, this study focused on the watershed analysis, which minimizes vascular dissection and avoids manipulation of the segmental bronchus. Furthermore, this research represents the first application of the watershed analysis technique in single-port thoracoscopic complex pulmonary segmentectomy. Watershed analysis with ICG fluorescence staining significantly reduced operative duration, intraoperative bleeding volume, risk of postoperative air leakage, and durations of postoperative tube placement and hospital stay. There were no significant differences observed in perioperative pulmonary function decline between the two groups. Our study introduces a more promising, safe, and effective surgical approach for complex pulmonary segmentectomy.
Acknowledgments
Funding: This study was supported by
Footnote
Reporting Checklist: The authors have completed the STROBE reporting checklist. Available at https://jtd.amegroups.com/article/view/10.21037/jtd-24-1075/rc
Data Sharing Statement: Available at https://jtd.amegroups.com/article/view/10.21037/jtd-24-1075/dss
Peer Review File: Available at https://jtd.amegroups.com/article/view/10.21037/jtd-24-1075/prf
Conflicts of Interest: All authors have completed the ICMJE uniform disclosure form (available at https://jtd.amegroups.com/article/view/10.21037/jtd-24-1075/coif). C.D. reports that the study was supported by Development Center for Medical Science & Technology National Health Commission of the People’s Republic of China (No. 202400660076). The other authors have no conflicts of interest to declare.
Ethical Statement: The authors are accountable for all aspects of the work in ensuring that questions related to the accuracy or integrity of any part of the work are appropriately investigated and resolved. The study was conducted in accordance with the Declaration of Helsinki (as revised in 2013), and received approval from the Institutional Review Board of The First Affiliated Hospital of Soochow University (Ethics Approval No. 2024186). Individual consent for this retrospective analysis was waived.
Open Access Statement: This is an Open Access article distributed in accordance with the Creative Commons Attribution-NonCommercial-NoDerivs 4.0 International License (CC BY-NC-ND 4.0), which permits the non-commercial replication and distribution of the article with the strict proviso that no changes or edits are made and the original work is properly cited (including links to both the formal publication through the relevant DOI and the license). See: https://creativecommons.org/licenses/by-nc-nd/4.0/.
References
- Bray F, Laversanne M, Sung H, et al. Global cancer statistics 2022: GLOBOCAN estimates of incidence and mortality worldwide for 36 cancers in 185 countries. CA Cancer J Clin 2024;74:229-63. [Crossref] [PubMed]
- Siegel RL, Miller KD, Wagle NS, et al. Cancer statistics, 2023. CA Cancer J Clin 2023;73:17-48. [Crossref] [PubMed]
- Adams SJ, Stone E, Baldwin DR, et al. Lung cancer screening. Lancet 2023;401:390-408. [Crossref] [PubMed]
- Saji H, Okada M, Tsuboi M, et al. Segmentectomy versus lobectomy in small-sized peripheral non-small-cell lung cancer (JCOG0802/WJOG4607L): a multicentre, open-label, phase 3, randomised, controlled, non-inferiority trial. Lancet 2022;399:1607-17. [Crossref] [PubMed]
- Altorki N, Wang X, Kozono D, et al. Lobar or Sublobar Resection for Peripheral Stage IA Non-Small-Cell Lung Cancer. N Engl J Med 2023;388:489-98. [Crossref] [PubMed]
- Kim K, Jeon OH, Choi BH, et al. Simultaneous Visualization of Lung Tumor and Intersegmental Plane during Pulmonary Segmentectomy by Intravenous Injection of Indocyanine Green. Cancers (Basel) 2024;16:1439. [Crossref] [PubMed]
- Zhang X, Li C, Jin R, Li H. Intraoperative Identification of the Intersegmental Plane: From the Beginning to the Future. Front Surg 2022;9:948878. [Crossref] [PubMed]
- Yao F, Wu W, Zhu Q, et al. Thoracoscopic Pulmonary Segmentectomy With Collateral Ventilation Method. Ann Thorac Surg 2021;112:1814-23. [Crossref] [PubMed]
- Sun Y, Zhang Q, Wang Z, et al. Is the near-infrared fluorescence imaging with intravenous indocyanine green method for identifying the intersegmental plane concordant with the modified inflation-deflation method in lung segmentectomy? Thorac Cancer 2019;10:2013-21. [Crossref] [PubMed]
- Xie M, Wang G, Xu M, et al. Comparison of Short-term Results of Preoperative Planning Combined with Fluorescence Video-assisted Thoracoscopic Precision Segmentectomy and Traditional Thoracoscopic Segmentectomy in the Treatment of Early Lung Adenocarcinoma. Zhongguo Fei Ai Za Zhi 2021;24:483-9. [PubMed]
- Handa Y, Tsutani Y, Mimae T, et al. Oncologic Outcomes of Complex Segmentectomy: A Multicenter Propensity Score-Matched Analysis. Ann Thorac Surg 2021;111:1044-51. [Crossref] [PubMed]
- Yao F, Wu W, Zhu Q, et al. Difficulty of Video-Assisted Thoracoscopic Surgery Segmentectomy: Proposal for a New Classification. Ann Surg Oncol 2023;30:6707-16. [Crossref] [PubMed]
- Weiss KD, Deeb AL, Wee JO, et al. When a segmentectomy is not a segmentectomy: Quality assurance audit and evaluation of required elements for an anatomic segmentectomy. J Thorac Cardiovasc Surg 2023;165:1919-25. [Crossref] [PubMed]
- Yang W, Liu Z, Yang C, et al. Combination of nitrous oxide and the modified inflation-deflation method for identifying the intersegmental plane in segmentectomy: A randomized controlled trial. Thorac Cancer 2021;12:1398-406. [Crossref] [PubMed]
- Wada H, Yamamoto T, Morimoto J, et al. Near-Infrared-Guided Pulmonary Segmentectomy After Endobronchial Indocyanine Green Injection. Ann Thorac Surg 2020;109:396-403. [Crossref] [PubMed]
- Pischik VG, Kovalenko AI, Molkova AV, et al. Indocyanine green fluorescence in thoracoscopic segmentectomy: indications and benefits. Khirurgiia (Mosk) 2024;(2. Vyp. 2):13-23.
- Yotsukura M, Okubo Y, Yoshida Y, et al. Indocyanine green imaging for pulmonary segmentectomy. JTCVS Tech 2021;6:151-8. [Crossref] [PubMed]
- Peeters M, Jansen Y, Daemen JHT, et al. The use of intravenous indocyanine green in minimally invasive segmental lung resections: a systematic review. Transl Lung Cancer Res 2024;13:612-22. [Crossref] [PubMed]
- Misaki N, Tatakawa K, Chang SS, et al. Constant-rate intravenous infusion of indocyanine green leading to high fluorescence intensity in infrared thoracoscopic segmentectomy. JTCVS Tech 2020;3:319-24. [Crossref] [PubMed]
- Iizuka S, Kuroda H, Yoshimura K, et al. Predictors of indocyanine green visualization during fluorescence imaging for segmental plane formation in thoracoscopic anatomical segmentectomy. J Thorac Dis 2016;8:985-91. [Crossref] [PubMed]
- Fu HH, Feng Z, Li M, et al. The arterial-ligation-alone method for identifying the intersegmental plane during thoracoscopic anatomic segmentectomy. J Thorac Dis 2020;12:2343-51. [Crossref] [PubMed]
- He H, Zhao H, Ma L, et al. Identification of the intersegmental plane by arterial ligation method during thoracoscopic segmentectomy. J Cardiothorac Surg 2022;17:281. [Crossref] [PubMed]
- Handa Y, Tsutani Y, Mimae T, et al. Surgical Outcomes of Complex Versus Simple Segmentectomy for Stage I Non-Small Cell Lung Cancer. Ann Thorac Surg 2019;107:1032-9. [Crossref] [PubMed]
- Ohtaki Y, Yajima T, Nagashima T, et al. Complex vs. simple segmentectomy: comparing surgical outcomes in the left upper division. Gen Thorac Cardiovasc Surg 2022;70:962-70. [Crossref] [PubMed]
- Kong XL, Lu J, Li PJ, et al. Technical aspects and early results of uniportal video-assisted thoracoscopic complex segmentectomy: a 30 case-series study. J Cardiothorac Surg 2022;17:63. [Crossref] [PubMed]
- Okubo Y, Yoshida Y, Yotsukura M, et al. Complex segmentectomy is not a complex procedure relative to simple segmentectomy. Eur J Cardiothorac Surg 2021;61:100-7. [Crossref] [PubMed]
- Bertolaccini L, Cara A, Bardoni C, et al. Should we use a biophysical approach in the classification and management of air leakage after lung resection? J Thorac Dis 2023;15:845-8. [Crossref] [PubMed]
- Zheng Q, Ge L, Zhou J, et al. Risk factors for prolonged air leak after pulmonary surgery: A systematic review and meta-analysis. Asian J Surg 2022;45:2159-67. [Crossref] [PubMed]
- Ponholzer F, Ng C, Maier H, et al. Risk factors, complications and costs of prolonged air leak after video-assisted thoracoscopic surgery for primary lung cancer. J Thorac Dis 2023;15:866-77. [Crossref] [PubMed]
- Yusa J, Suzuki H, Yoshino I. EP01.01-22 Risk Factors of Intraoperative Air Leakage in Anatomical Pulmonary Resection. J Thorac Oncol 2023;18:S422. [Crossref]