Prediction of impaired lung diffusion capacity in COVID-19 pneumonia survivors
Highlight box
Key findings
• Pulmonary interstitial damage caused by severe acute respiratory syndrome-related coronavirus 2 (SARS-CoV-2) definitely contributes to reduced lung diffusion capacity for carbon monoxide (DLco) after a hospital discharge.
What is known and what is new?
• Some studies on pulmonary function following infection with SARS-CoV-2 demonstrated consistent results with decreased DLco 3, 6 months, and 1 year following infection.
• Our study indicates that analysis of computed tomography (CT) scans during the acute phase of coronavirus disease 2019 (COVID-19) may have prognostic relevance for abnormal DLco after a hospital discharge.
What is the implication, and what should change now?
• Odds ratio showed that with CTmax of 45% or more (according to the visual scale recommended by temporary methodological recommendations of prevention, diagnostics and treatment of new coronavirus infection (COVID-19), decreased DLco can be reliably expected after the acute phase of COVID-19, which makes it possible to identify patients with a high probability of abnormal DLco after a hospital discharge, prescribe appropriate treatment and control of DLco in the post-acute COVID-19 phase.
• These findings should be considered during follow-up COVID-19 survivors, and long-term patient management.
Introduction
The coronavirus disease 2019 (COVID-19) has been identified worldwide with over 704,753,890 known cases and over 7,010,681 million global deaths, 675,619,811 patients have recovered from the initial illness (1). Nevertheless, for many months following the infection with severe acute respiratory syndrome-related coronavirus 2 (SARS-CoV-2) a lot of discharged patients have ongoing symptoms including fatigue, insomnia, muscle weakness, and dyspnea (2). Some studies on pulmonary function following infection with SARS-CoV-2 demonstrated consistent results with decreased transfer-factor for lung diffusion capacity for carbon monoxide (DLco) 3, 6 months, and 1 year following infection (3-7).
Therefore, it is important to identify patients with a high probability of abnormal DLco after a hospital discharge.
The aim of our study was to identify important predictors of lung diffusion capacity impairment in COVID-19 survivors. We present this article in accordance with the STROBE reporting checklist (available at https://jtd.amegroups.com/article/view/10.21037/jtd-24-1118/rc).
Methods
Study design
The study was conducted in accordance with the Declaration of Helsinki (as revised in 2013). The study was approved by the Ethics Committee of the Pulmonology Scientific Research Institute of the Federal Medical and Biological Agency of Russia (protocol No. 01-21 of May 14, 2021) and the Ethics Committee of the Sechenov University (protocol No. 02-23 of January 26, 2023). Individual consent for this retrospective analysis was waived.
This was a retrospective multi-center cohort study performed at two medical centers: Sechenov First Moscow State Medical University (Sechenov University), and Pulmonology Scientific Research Institute, Moscow. All patients had subsequent laboratory confirmation of SARS-CoV-2 using real-time polymerase chain reaction (RT-PCR). The patients’ radiological, demographic, anthropometric data and lung function after the hospital discharge were recorded. Data were collected between May 2021 and April 2023.
In order to be included in the study, patients had to have COVID-19 with the radiographic evidence of bilateral pneumonia and chest high-resolution computed tomography (HRCT) findings in the acute phase of COVID-19, as well as follow-up pulmonary function tests (PFTs) (spirometry, body plethysmography, diffusion test) after the hospital discharge. For patients with several computed tomography (CT) scans during the acute phase of COVID-19, the greatest chest imaging abnormalities were retained (CTmax, %). Patients were excluded if they were younger than 18 years old and reported to have chronic pulmonary disease in past history or diseases affecting lung function or pregnancy (Figure 1).
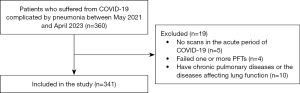
Lung function tests
Three hundred and forty-one patients were enrolled in the retrospective cohort study with diagnosis “Condition after COVID-19” according ICD-10 (U09.9). PFT data were obtained in Master Screen Body/Diff (Viasys Healthcare/Erich Jager, Vyaire Medical/Erich Jager, Germany). Both centers participating in this study used the same equipment, quality checks, and protocols. All procedures were performed according to the national and international guidelines (8-11) and recommendations of the Russian Respiratory Society for performing PFTs during the COVID-19 pandemic (12). PFTs results were expressed as a percentage of the predicted value (%pred) according to the equations of the European Coal and Steel Community (13).
Recorded parameters include: total lung capacity (TLC), vital capacity (VC), forced vital capacity (FVC), residual volume (RV), RV/TLC, forced expiratory volume in the first second (FEV1), FEV1/VC ratio (reported as the raw number ratio), DLco adjusted for hemoglobin, the modified Medical Research Council (mMRC) dyspnoea score and quality of life (QoL) using the EuroQoL Visual Analogue Scale.
Chest CT
Chest HRCT was performed on a 640-medium using the Canon Aquilion One CT scanner Genesis (Canon Medical Systems Corporation, Shimoishigami, Japan). HRCT was performed at maximum inspiration in a spiral mode, the thickness of the middle slice was in increments of 1 mm. Post-processing and image analysis were carried out by two independent radiologists. The following parameters were assessed:
- Ground-glass opacities (their intensity and prevalence);
- Areas of consolidation.
A semi-quantitative CT score was calculated based on the extent of lung involvement. The % of the lesion is assessed separately for each lung: CTmax 0: 0% involvement; CTmax 1, <25% involvement; CTmax 2: 25–49% involvement; CTmax 3: 50–75% involvement; CTmax 4: >75% involvement. The degree of the lesion is estimated by the lung with the greatest lesion according to the visual scale recommended by temporary methodological recommendations of prevention, diagnostics and treatment of new coronavirus infection (COVID-19) (14).
Statistical analysis
Statistical analysis was performed using SPSS version 21 and Microsoft Excel 2016. The normality of the distribution was analyzed using the Shapiro-Wilk test, the Lilliefors test, and the Kolmogorov-Smirnov test. Continuous variables of a non-normal distribution are expressed as median (Me) and the 25th and 75th centile values [Q1–Q3] and compared using the Mann-Whitney U-test. Categorial variables are expressed as frequency and percentage and compared using the Chi-squared test with correction for continuity in the case of a non-normal distribution. The Partial correlation (r) and the Spearman correlation (rs) were used to study relations between variables. A P value <0.05 was considered significant.
The multivariable logistic regression analysis was used to explore risk factors associated with lung diffusion capacity impairment.
The receiver operating characteristic (ROC) curve of multivariate observation and the area under the curve (AUC) were used to assess the performance of a model. Classification quality assessment was performed using standard metrics: sensitivity and specificity. The cut-off value was determined as a maximum of sum sensitivity and specificity. All tests were two-sided, and a P value less than 0.05 was considered statistically significant.
Results
The characteristics of patients are shown in Table 1. Median age was 48 years, 76.8% (262/341) were men, 57.2% (195/341) patients had severe COVID-19. The COVID-19 was clinically classified into severe disease in case of dyspnoea, respiratory frequency over 30/min, oxygen saturation less than 93%, PaO2/FiO2 ratio less than 300 and/or lung infiltrates more than 50% of the lung field within 24–48 hours.
Table 1
Parameter | Value (n=341) |
---|---|
Gender, males | 262 (76.8) |
Age, years | 48 [41.5–57] |
BMI, kg/m2 | 29.9 [27–32.5] |
Smoking index, pack-years | 0 [0–5.13] |
CTmax, % | 50 [31–75] |
CTmax 1 | 47 (13.8) |
CTmax 2 | 99 (29.0) |
CTmax 3 | 129 (37.8) |
CTmax 4 | 66 (19.4) |
Time interval, days | 53 [28.5–111] |
Number of patients | |
<90 days | 221 (64.8) |
90–180 days | 80 (23.5) |
>180 days | 40 (11.7) |
VC, %pred. | 102 [87–111] |
VC <80%pred. | 63 (18.5) |
FVC, %pred. | 103 [88–114.2] |
FEV1, %pred. | 101 [89–113] |
FEV1 <80%pred. | 58 (17.0) |
FEV1/VC, % | 80.31 [76.4–84.3] |
FEV1/VC <0.7 | 25 (7.3) |
TLC, %pred. | 98 [83.2–108] |
TLC <80%pred. | 68 (19.9) |
RV, %pred. | 93 [78.2–108] |
RV <80%pred. | 88 (25.8) |
RV/TLC, %pred. | 92 [84–100] |
DLco, %pred. | 75 [61.7–88.3] |
DLco <80%pred. | 206 (60.4) |
mMRC score | 1 [1–2]* |
mMRC score ≥1 | 81 (89.0)* |
QoL score | 70 [60–75]* |
The data are presented as n (%) or median [Q1–Q3]. *, n=91. BMI, body mass index; CTmax, the total severity score of lung involvement in the acute period of COVID-19 in CT images; CTmax 1, 2, 3, 4, the extent of lung involvement <25%, 25–49%, 50–75%, >75%, respectively; time interval, the time interval between the onset of COVID-19 symptoms and follow-up pulmonary function tests; VC, vital capacity; FVC, forced vital capacity; FEV1, forced expiratory volume in 1 second; TLC, total lung capacity; RV, residual volume; DLco, diffusing capacity of the lung for carbon monoxide; mMRC, the modified Medical Research Council Dyspnoea Scale; QoL score, quality of life using the EuroQoL Visual Analogue Scale; %pred., % of the predicted value; CT, computed tomography; COVID-19, coronavirus disease 2019.
All patients had bilateral pneumonia, organizing pneumonia after acute phase of COVID-19 had 8% (27/341) patients; 5% (17/341) patients (all of them were critically ill) had superimposed bacterial pneumonia during hospitalization, 3.2% (11/341) patients were treated with invasive mechanical ventilation (IMV). The majority of patients (62%) had no smoking history, 26.7% were ever-smokers, and 80% were overweighted [body mass index (BMI) >25 kg/m2]. At the time of the analysis, 64.8% (221/341) patients participated in follow-up visits on <90 days, 23.5% (80/341) on 90–180 days, and 11.7% (40/341) on more than 180 days after the onset of COVID-19 symptoms. Seventy-three percent of patients had one or more underlying diseases, predominantly hypertension, diabetes, and hypothyroidism.
The median CTmax was 50% [31–75%]. Maximum lung involved peaked at 11 days from the onset of initial symptoms. The median time interval between the onset of initial symptoms and CTmax was 11 [6–13] days. There were 13.8% of patients with CTmax 1, 29% with CTmax 2, 37.8% with CTmax 3 and 19.4 with CTmax 4 in the study sample.
TLC was reduced (<80%pred.) in 19.9% (68/341) patients, 7.3% (25/341) patients had airway obstruction (FEV1/VC <0.7), 17% (58/341), 18.5% (63/341), and 25.8% (88/341) patients had reduced FEV1, VC, and RV (<80%pred.), respectively, while 60.4% (206/341) had abnormal DLco (<80%pred.).
Eighty nine percent of patients experienced dyspnoea (mMRC score ≥1). The median of mMRC score was 1 [1–2]. The median of QoL score was 70 [60–75]. The moderate negative correlation between DLco and mMRC dyspnoea (rs=−0.45; P<0.001) and weak positive correlation between DLco and QoL (rs=0.27; P<0.008) were found.
In order to differentiate normal and impaired DLco, the patients were classified into two groups depending on the DLco value: group 1, normal DLco (n=135) and group 2, reduced DLco (n=206). The groups were analyzed depending on CTmax, the time interval between the onset of COVID-19 symptoms and follow-up PFTs (time interval), gender, age, and BMI (Table 2).
Table 2
Parameter | Group 1, normal DLco (n=135) (39.6%) | Group 2, reduced DLco (n=206) (60.4%) | P value |
---|---|---|---|
Gender, male/female | 107 (79.3)/28 (20.7) | 155 (75.2)/51 (24.8) | 0.471 |
Age, years | 47 [40–58] | 49 [43–57] | 0.542 |
BMI, kg/m2 | 29.7 [26.9–32.3] | 30 [27–33] | 0.582 |
Time interval, days | 81 [42–145] | 39.5 [27–93] | <0.0012 |
CTmax, % | 35 [25–52] | 70 [48–80] | <0.0012 |
The data are presented as n (%) or median [Q1–Q3]. Group 1, patients with normal DLco at the time of pulmonary function tests; group 2, patients with reduced DLco at the time of pulmonary function tests. 1, Chi-squared test with correction for continuity; 2, the Mann-Whitney U-test. DLco, diffusing capacity of the lung for carbon monoxide; BMI, body mass index; time interval, the time interval between the onset of COVID-19 symptoms and follow-up pulmonary function tests; CTmax, the total severity score of lung involvement in the acute period of COVID-19 in CT images; CT, computed tomography; COVID-19, coronavirus disease 2019.
Table 2 shows that CTmax was higher and time interval was lower in group 2, and the differences between the groups were statistically significant whereas there were no significant differences in age, gender, and BMI between the groups.
The partial correlation coefficient demonstrated the significant negative correlation of DLco with CTmax (r=−0.601; P<0.01) with the effect of gender, age, BMI, and time interval removed (Figure 2).
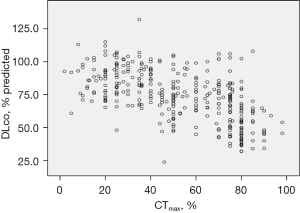
The above-mentioned predictors with either conceptual importance (age, gender, BMI) or a statistically significant relationship to reduced DLco according to Table 2 were encapsulated in the logistic regression analysis to explore the prediction of reduced DLco in the training sample.
Backward stepwise regression was applied to eliminate insignificant predictors. The following logistic regression equation was obtained:
where x means CTmax (%).
In this way, it was found that CTmax was important predictor of impaired DLco.
ROC analysis was used to assess the quality of the obtained logistic regression to differentiate between normal and abnormal DLco (<80%pred.). AUC value was 0.780 [95% confidential interval (CI): 0.723–0.837, P<0.001] (Figure 3). The sensitivity and specificity in the training group were 80% and 67%, respectively. Then the obtained rule was checked on the testing sample, the sensitivity and specificity were 79% and 70%, respectively.
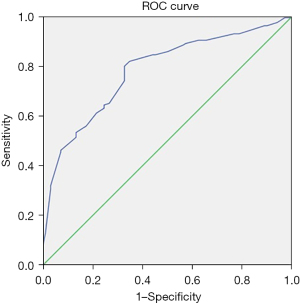
Then using obtained logistic regression equation the odds ratio (OR) was calculated. The values of CTmax =45% (OR 1.21, 95% CI: 1.095–1.334) and more will be significantly associated with reduced DLco.
Discussion
In line with the previous observation (15), this study did not reveal age, gender, and BMI differences between the groups with normal and abnormal DLco in COVID-19 survivors. Besides, Qin et al. (15), applying univariable logistic analysis, showed that the severity of COVID-19, total severity score (TSS) >10.5 [TSS was evaluated by the percentage of involvement in each lobe and overall lung in accordance with Li et al. (16)], the main pulmonary arterial (MPA) diameter at admission and acute respiratory distress syndrome (ARDS) were significantly associated with impaired DLco. Then after putting age, MPA diameter, TSS >10.5, and ARDS into the multivariable logistic regression model, the authors found that TSS >10.5 (OR 10.4; 95% CI: 2.5–44.1; P=0.001) and ARDS (OR 4.6; 95% CI: 1.4–15.5; P=0.014) were significantly associated with abnormal DLco.
In the study by Huang et al. (17), PFTs were performed in 349 patients 6 months after acute COVID-19. The authors showed that participants requiring high-flow nasal cannula for oxygen therapy or non-invasive ventilation or IMV had a significant risk of abnormal DLco (OR 4.6; 95% CI: 1.85–11.48; P<0.001).
Steinbeis et al. (18) analyzed reductions in pulmonary function and respiratory-related QoL for up to 12 months in 180 patients after acute COVID-19. Univariate logistic regression showed an association of reduced DLco with initial disease severity, sex (male), the St. George’s Respiratory Questionnaire outcome (score >25), Charlson Comorbidity Index, and cardiovascular disease. The odds of reduced DLco increased with acute COVID-19 disease severity whereas logistic regression showed no association reduced DLCO with initial pulmonary involvement as determined by the CT score (P=0.11).
On the contrary, according to our model, the largest contribution to reduced DLco in the post-acute COVID-19 phase is made by CTmax. For the training sample the sensitivity and specificity of the model were 80% and 67%, respectively. OR showed that with CTmax of 45% or more (according to the visual scale recommended by Temporary methodological recommendations of prevention, diagnostics and treatment of new coronavirus infection (COVID-19) (14), reduced DLco can be reliably expected after the acute phase of COVID-19, which makes it possible to identify patients with a high probability of abnormal DLco after a hospital discharge, prescribe appropriate treatment and control of DLco in the post-acute COVID-19 phase.
In addition, we have analyzed mMRC dyspnoea and QoL in 91 patients during follow-up visits. Our results are consistent with the data of Huang et al. (19) and Sanchez-Ramirez et al. (20), which are respiratory symptoms and decreased QoL up to 6 months or more. In our opinion, the moderate correlation DLco and mMRC and the weak correlation DLco and QoL shows that there are other reasons, not only reduced DLco, affecting the QoL in COVID-19 survivors.
The present study has several limitations. First, the lack of baseline PFT results prior to COVID-19 makes it difficult to make a comparison with the results after the illness. However, owing to the absence of patients with chronic respiratory diseases in this study, it should be acceptable to speculate that the pulmonary function before COVID-19 had been normal. Second, a shortcoming of the model is the small sample size more than 180 days after COVID-19 symptoms onset. Third, the resulting model provides a binary shift of DLco (normal or reduced). In this regard, the proposed model does not provide a full-fledged replacement of the diffusion test, as it does not allow quantification of the DLco value. In addition, it is worth encapsulating the predictors such as the parameters of coagulation abnormalities during acute phase of COVID-19 in the logistic regression analysis to explore the impact of pulmonary angiopathy on DLco.
Conclusions
Pulmonary interstitial damage caused by SARS-CoV-2 definitely contributes to reduced DLco after a hospital discharge. This indicates that analysis of CT scans during the acute phase of COVID-19 may have prognostic relevance for abnormal DLco after a hospital discharge.
Acknowledgments
The authors would like to thank M.R. Zaitov, an engineer for ZAO “Meditsinskiye Sistemy”, for his technical support.
Funding: None.
Footnote
Reporting Checklist: The authors have completed the STROBE reporting checklist. Available at https://jtd.amegroups.com/article/view/10.21037/jtd-24-1118/rc
Data Sharing Statement: Available at https://jtd.amegroups.com/article/view/10.21037/jtd-24-1118/dss
Peer Review File: Available at https://jtd.amegroups.com/article/view/10.21037/jtd-24-1118/prf
Conflicts of Interest: All authors have completed the ICMJE uniform disclosure form (available at https://jtd.amegroups.com/article/view/10.21037/jtd-24-1118/coif). The authors have no conflicts of interest to declare.
Ethical Statement: The authors are accountable for all aspects of the work in ensuring that questions related to the accuracy or integrity of any part of the work are appropriately investigated and resolved. The study was conducted in accordance with the Declaration of Helsinki (as revised in 2013). The study was approved by the Ethics Committee of the Pulmonology Scientific Research Institute of the Federal Medical and Biological Agency of Russia (protocol No. 01-21 of May 14, 2021) and the Ethics Committee of the Sechenov University (protocol No. 02-23 of January 26, 2023). Individual consent for this retrospective analysis was waived.
Open Access Statement: This is an Open Access article distributed in accordance with the Creative Commons Attribution-NonCommercial-NoDerivs 4.0 International License (CC BY-NC-ND 4.0), which permits the non-commercial replication and distribution of the article with the strict proviso that no changes or edits are made and the original work is properly cited (including links to both the formal publication through the relevant DOI and the license). See: https://creativecommons.org/licenses/by-nc-nd/4.0/.
References
- COVID-Coronavirus Statistics-Worldometer. [cited 2024 June 25]. Available online: https://www.worldometers.info/coronavirus
- Shivani F, Kumari N, Bai P, et al. Long-Term Symptoms of COVID-19: One-Year Follow-Up Study. Cureus 2022;14:e25937. [Crossref] [PubMed]
- Huang Y, Tan C, Wu J, et al. Impact of coronavirus disease 2019 on pulmonary function in early convalescence phase. Respir Res 2020;21:163. [Crossref] [PubMed]
- Zhao YM, Shang YM, Song WB, et al. Follow-up study of the pulmonary function and related physiological characteristics of COVID-19 survivors three months after recovery. EClinicalMedicine 2020;25:100463. [Crossref] [PubMed]
- Savushkina OI, Cherniak AV, Kryukov EV, et al. Follow-up pulmonary function of COVID-19 patients 4 months after hospital discharge. Pulmonologiya 2021;31:580-6. [Crossref]
- Karchevskaya NA, Skorobogach IM, Cherniak AV, et al. Long-term follow-up study of post-COVID-19 patients. Ter Arkh 2022;94:378-88. [Crossref] [PubMed]
- Suppini N, Fira-Mladinescu O, Traila D, et al. Longitudinal Analysis of Pulmonary Function Impairment One Year Post-COVID-19: A Single-Center Study. J Pers Med 2023;13:1190. [Crossref] [PubMed]
- Graham BL, Steenbruggen I, Miller MR, et al. Standardization of Spirometry 2019 Update. An Official American Thoracic Society and European Respiratory Society Technical Statement. Am J Respir Crit Care Med 2019;200:e70-e88. [Crossref] [PubMed]
- Graham BL, Brusasco V, Burgos F, et al. 2017 ERS/ATS standards for single-breath carbon monoxide uptake in the lung. Eur Respir J 2017;49:1600016. [Crossref] [PubMed]
- Wanger J, Clausen JL, Coates A, et al. Standardisation of the measurement of lung volumes. Eur Respir J 2005;26:511-22. [Crossref] [PubMed]
- Kameneva MYu, Cherniak AV, Aisanov ZR, et al. Spirometry: national guidelines for the testing and interpretation of results. Pulmonologiya 2023;33:307-40. [Crossref]
- Recommendations of the Russian Respiratory Society on conducting lung function tests during the COVID-19 pandemic Version 1.1. from 05/19/2020. Available online: https://spulmo.ru
- Quanjer PH, Tammeling GJ, Cotes JE, et al. Lung volumes and forced ventilatory flows. Report Working Party Standardization of Lung Function Tests, European Community for Steel and Coal. Official Statement of the European Respiratory Society. Eur Respir J Suppl 1993;16:5-40. [Crossref] [PubMed]
- Temporary methodological recommendations of prevention, diagnostics and treatment of new coronavirus infection (COVID-19). Versiya 18 (2023.10.18). (In Russian). Available online: https://static-0.minzdrav.gov.ru/system/attachments/attaches/000/064/610/original/%D0%92%D0%9C%D0%A0_COVID-19_V18.pdf
- Qin W, Chen S, Zhang Y, et al. Diffusion capacity abnormalities for carbon monoxide in patients with COVID-19 at 3-month follow-up. Eur Respir J 2021;58:2003677. [Crossref] [PubMed]
- Li K, Fang Y, Li W, et al. CT image visual quantitative evaluation and clinical classification of coronavirus disease (COVID-19). Eur Radiol 2020;30:4407-16. [Crossref] [PubMed]
- Huang C, Huang L, Wang Y, et al. 6-month consequences of COVID-19 in patients discharged from hospital: a cohort study. Lancet 2021;397:220-32. [Crossref] [PubMed]
- Steinbeis F, Thibeault C, Doellinger F, et al. Severity of respiratory failure and computed chest tomography in acute COVID-19 correlates with pulmonary function and respiratory symptoms after infection with SARS-CoV-2: An observational longitudinal study over 12 months. Respir Med 2022;191:106709. [Crossref] [PubMed]
- Huang L, Yao Q, Gu X, et al. 1-year outcomes in hospital survivors with COVID-19: a longitudinal cohort study. Lancet 2021;398:747-58. [Crossref] [PubMed]
- Sanchez-Ramirez DC, Normand K, Zhaoyun Y, et al. Long-Term Impact of COVID-19: A Systematic Review of the Literature and Meta-Analysis. Biomedicines 2021;9:900. [Crossref] [PubMed]