Assessment of tumor biomarkers for prognosis in interstitial lung disease associated with connective tissue disease: a prospective study
Highlight box
Key findings
• Circulating carcinoembryonic antigen (CEA) levels may be associated with pulmonary fibrosis progression and prognosis of connective tissue disease-associated interstitial lung disease (CTD-ILD).
What is known and what is new?
• Tumor markers are not exclusively elevated in patients with malignancy but also in some CTD-ILD.
• Elevated CEA level is an independent risk factor associated with fibrosis progression, all-cause mortality and acute exacerbation in CTD-ILD patients.
What is the implication, and what should change now?
• CEA may serve as a potential biomarker in early detection of rapid progression in CTD-ILD. However, the mechanisms underlying the elevation of CEA in CTD-ILD needs to be further explored.
Introduction
Connective tissue diseases (CTDs) constitute a broad group of systemic disorders characterized by dysfunction in multiple organ systems due to inflammation and fibrosis (1). Among patients with CTDs, respiratory complications are prevalent, with interstitial lung disease (ILD) emerging as the most common manifestation (1,2). ILD presents across various CTDs, including idiopathic inflammatory myopathies (IIMs) (3), primary Sjögren syndrome (pSS) (4), systemic lupus erythematosus (SLE) (5), systemic sclerosis (SSc) (6), mixed CTD (MCTD) (7), rheumatoid arthritis (RA) (8), undifferentiated CTD (UCTD) (9), and overlap connective tissue disorders (OCTD) (10). The disease course of CTD-ILD is known for its heterogeneity. Some ILDs within this group exhibit a clinical progression similar to idiopathic pulmonary fibrosis (IPF) and have been termed as progressive pulmonary fibrosis (PPF), marked by a growing extent of pulmonary fibrosis on high-resolution computed tomography (HRCT), a decline in lung function, exacerbation of respiratory symptoms, and high mortality rates despite available treatments (11-13). Our previous study revealed that 50% of RA-ILD patients exhibited progressive imaging findings over a median follow-up duration of 19.1 months (14). Additionally, a retrospective cohort analysis indicated that the use of antifibrotic medications was associated a decreased risk of developing PPF in patients with myositis-specific antibody-positive ILD (15). The early identification of patients who are prone to rapid progression holds the potential to significantly enhance clinical management (16).
Tumor markers play a crucial role in the screening and diagnosis of common cancers, indicating abnormal elevations in individuals with malignant diseases (17). However, these markers are not exclusively elevated in patients with malignancy but also in some benign lung diseases. The correlations between carcinoembryonic antigen (CEA), carbohydrate antigen 125 (CA125), carbohydrate antigen 19-9 (CA19-9) with disease severity, lung functional decline, and prognosis have been reported in IPF and non-IPF ILD patients (18-21).
The mechanisms leading to elevation of these markers’ levels in ILDs are unknown. One hypothesis is that excessive tumor markers are released by regenerating epithelial cells in damaged lungs (19). Mucus plays a crucial role in maintaining lung health and function, with mucin glycoproteins being its major components (21). These glycoproteins, including mucin 1 [cancer antigen 15-3 (CA15-3)], mucin 5 (MUC5), mucin 16 (CA125), and Lewis antigens (CA19-9), are secreted by surface epithelial goblet cells, submucosal gland mucous and serous cells, or are attached to cell membranes (21). CEA, a membrane-anchored immunoglobulin-related protein implicated in cell adhesion, differentiation, and various recognition processes, is also targeted to the apical membrane of polarized epithelial cells (22). Epithelial CEA-related cell adhesion molecule (CEACAM) activation triggers intracellular signaling which may contribute to fibrosis (23). However, the factors driving elevated tumor marker levels and their correlation with ILDs are currently speculative.
Despite previous studies, the clinical implication of tumor markers in relation to CTD-ILD is still ambiguous (24-27). In this prospective cohort study, we sought to evaluate the potential association between serum tumor markers and fibrosis progression in CTD-ILD patients. By shedding light on this relationship, our research may contribute to a better understanding of disease progression in CTD-ILD and provide valuable insights for clinical decision-making. We present this article in accordance with the STARD reporting checklist (available at https://jtd.amegroups.com/article/view/10.21037/jtd-24-922/rc).
Methods
Study design and patient selection
This was a single-center prospective cohort study. Patients greater than or equal to 18 years old with newly diagnosed CTD-ILD were consecutively recruited from January 1, 2018, and January 1, 2022, at Beijing Chao-Yang Hospital, China, a regional medical center specializing in ILDs. Enrolled patients were prospectively followed from diagnosis to death, loss of follow-up, or censoring date (January 2023). All patients were eligible for classification/diagnosis criteria of the corresponding disease through expert multidisciplinary discussion. RA diagnosis was based on the American College of Rheumatology (ACR) and European League against Rheumatism (EULAR) 2010 classification criteria (8), while pSS was diagnosed following the 2016 ACR/EULAR guidelines (4). IIM diagnosis adhered to the ACR/EULAR 2017 criteria-confirmed diagnosis (3), and SLE diagnosis was determined based on the Systemic Lupus International Collaborating Clinics-2012 (28) or 2019 ACR/EULAR guidelines (5). SSc diagnosis was defined by the ACR/EULAR 2013 classification criteria (6). Patients exhibiting features of more than two autoimmune diseases were classified as having OCTD (10). Patients were diagnosed with UCTD if they displayed at least one symptom associated with CTDs and at least one evidence of systemic inflammation, without meeting the accepted classification criteria for any defined CTDs (9). The presence of ILD was confirmed on HRCT examinations. Patients were excluded for the following reasons: (I) concurrent lung cancer or other malignancies at baseline and during follow-up; (II) concomitant other pulmonary diseases, such as pulmonary embolism and pneumoconiosis, asbestosis, ILD caused by inhalation of organic matter and other causes; (III) other concomitant diseases that could lead to elevated tumor markers, such as pancreatitis, biliary disease, cirrhosis, inflammatory bowel disease; (IV) incomplete baseline data or loss to follow-up (Figure 1). The healthy controls (HCs) group comprised 63 volunteers selected from the health examination center of Beijing Chao-Yang Hospital during the same time frame.
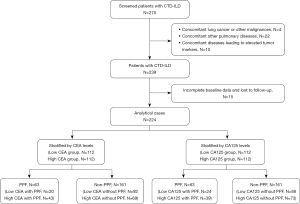
The study was registered with the Chinese Clinical Trial Registry (ChiCTR2100049247). It was conducted in accordance with the principles of the Declaration of Helsinki (as revised in 2013) and was approved by the Institutional Review Board of Beijing Chao-Yang Hospital (2022-KY-529). Written informed consent was obtained from all participants.
Data collection
Baseline data including age, sex, body mass index, smoking history, clinical manifestations, and comorbidities were collected at the time of diagnosis. All patients underwent a pulmonary function test (PFT) [percent predicted for forced vital capacity (FVC% pred.), percent predicted for diffusion capacity of the lung for carbon monoxide (DLCO% pred.) corrected for hemoglobin (Hb)] and HRCT examinations. The HRCT images were evaluated by two ILD experts (N.W. and D.S.) who were blinded to all patient information. The following HRCT findings were noted: honeycombing, bronchiectasis and emphysema. Circulating tumor markers at the time of diagnosis, including CEA, squamous cell carcinoma (SCC) antigen, neuron-specific enolase (NSE), cytokeratin fraction 21-1 (CYFRA21-1), serum ferritin (SF), alpha-fetoprotein (AFP), CA125, CA19-9, and carbohydrate antigen 724 (CA724) were documented. The concentration of tumor markers was measured by laboratory personnel who were blinded to other clinical information, using an electrochemiluminescence immunoassay on a Roche Hitachi Cobas instrument (Hitachi, Tokyo, Japan). The recommended normal ranges used were: CEA ≤5 ng/mL; SCC ≤1.5 ng/mL; NSE ≤16.3 ng/mL; CYFRA21-1 ≤2.08 ng/mL; SF ≤291 µg/L; AFP ≤8.1 ng/mL; CA125 ≤30.2 U/mL; CA19-9 ≤37 U/mL and CA724 ≤8.2 U/mL.
Follow-up strategy
Follow-up assessments were conducted at months 3, 6, 9 and 12 for PPF and every 6 months, thereafter (available online: https://cdn.amegroups.cn/static/public/jtd-24-922-1.docx). Symptomatic evaluations were based on self-reports from the patients. HRCT and PFT results were gathered during the follow-up period. The treatment choices consisted of corticosteroids, either as monotherapy or in combination with immunosuppressants and antifibrotic therapy (nintedanib or pirfenidone). The treatment was documented if it was administered for a duration exceeding 12 weeks.
The primary outcome of the study was the occurrence of PPF during the first 12 months. PPF was defined by meeting at least two of three criteria (worsening symptoms, radiological progression, and physiological progression) within a 12-month period according to the international clinical practice guideline (12). Physiological progression was defined as either of the following: (I) an absolute decline in FVC% pred. >5% or (II) an absolute decline in DLCO% pred. (corrected for Hb) >10% within 12 months. The experts exhibited a high degree of concordance in their assessments of radiological progression with kappa values of 0.81. Any disagreements between the experts were resolved through consensus. Secondary outcomes included acute exacerbation (AE), and all-cause mortality. AE was defined using the criteria proposed by the IPF: an acute clinically significant respiratory deterioration, typically less than 1month in duration, and could be categorized as extraparenchymal or parenchymal (29).
Statistical analysis
Mean (standard deviation) or median (first and third quartiles) were used to describe continuous variables as appropriate. Categorical variables were described as frequencies and percentages. Group differences for continuous variables were assessed using the Mann-Whitney U or t-test, and categorical variables were compared using the Chi-squared test or Fisher exact test. Missing data were imputed using the multiple imputation chained equations procedure (available online: https://cdn.amegroups.cn/static/public/jtd-24-922-1.docx).
To assess the discrimination of tumor markers in identify PPF, logistic regression analyses and receiver operating characteristic (ROC) curves were employed. The association of elevated tumor markers with PPF prevalence was explored by logistic regression model. Model 1 (the crude model) did not adjust for any potential confounders. Model 2 (the adjusted model) accounted for factors that were unevenly distributed between groups at baseline, including age, pulmonary hypertension (PH), honeycombing, emphysema, and DLCO% pred. Both a categorical and a continuous model were incorporated. In the continuous model, tumor markers concentrations were log2 transformed to minimize influential extrema. Spearman correlation coefficient was employed to assess correlations between tumor markers and clinical characteristics. Cox analyses were conducted to assess the associations between tumor markers and both PPF, AE and mortality. A weighted restricted cubic spline (RCS) model was constructed to explore the dose-response relationship of tumor markers with PPF, mortality, and AE.
Subgroup analyses were performed to examine the modification effects of age, sex, smoking, FVC% pred., DLCO% pred., honeycombing, emphysema, bronchiectasis and antifibrotic treatment on the association between tumor marker concentrations and PPF. Interaction effects were assessed by adding a multiplicative interaction term between tumor marker concentrations and the corresponding stratification variable. The relationships between CEA or CA125 concentrations and PPF in specific diagnoses were also analyzed separately. As for sensitivity analyses, firstly, the correlation between CEA and CA125 with PPF was reassessed by excluding PPFs that could be linked to AE. Secondly, recognizing the impact of emphysema on FVC, the correlation was reassessed by excluding patients without emphysema. Statistical analyses were performed with R version 4.2.2. Statistical significance of P<0.05 was assumed.
Results
Demographics and clinical characteristics
A total of 224 patients and 63 healthy participants were ultimately included in the analysis; the median follow-up duration for CTD-ILD patients was 40 (first and third quartiles, 29–52) months (Table 1). A total of 63 (28.1%) patients experienced PPF during the first year; 58 (25.9%) patients experienced AE, and 34 (15.2%) patients died at the end of the follow-up. Regarding specific diagnoses, the proportions of IIM-ILD, pSS-ILD, UCTD-ILD, OCTD-ILD, RA-ILD, SSc-ILD, and SLE-ILD in the entire cohort were 36.6%, 20.5%,17.0%, 10.7%, 8,5%, 5.8%, and 0.9% respectively (available online: https://cdn.amegroups.cn/static/public/jtd-24-922-1.docx). The HCs and CTD-ILD patients were matched in sex, age and smoking status (available online: https://cdn.amegroups.cn/static/public/jtd-24-922-1.docx). Serum CEA, CA19-9, SF, CYFRA21-1, CA12-5 and CA724 levels were higher in patients with CTD-ILD than in healthy participants (Figure 2). Notably, patients with PPF only presented significantly higher serum CEA (median level 2.09 versus 1.48 ng/mL, P=0.002) and CA125 (median level 17.4 versus 13.5 U/mL, P=0.03) than those without PPF (available online: https://cdn.amegroups.cn/static/public/jtd-24-922-1.docx).
Table 1
Variables | All | CEA | CA125 | |||||
---|---|---|---|---|---|---|---|---|
≤1.70 ng/mL | >1.70 ng/mL | P value | ≤14.95 U/mL | >14.95 U/mL | P value | |||
N | 224 | 112 | 112 | 112 | 112 | |||
Male | 80 (35.7) | 36 (32.1) | 44 (39.3) | 0.54 | 38 (33.9) | 42 (37.5) | 0.58 | |
Age, years | 60.1 (11.6) | 57.6 (11.1) | 62.6 (11.7) | 0.001 | 58.0 (10.8) | 62.2 (12.1) | 0.007 | |
BMI, kg/m2 | 25.5 (3.5) | 25.2 (3.4) | 25.8 (3.7) | 0.16 | 25.4 (3.5) | 25.6 (3.5) | 0.72 | |
Smoking status | ||||||||
Ever-smokers | 58 (25.9) | 27 (24.1) | 31 (27.7) | 0.38 | 25 (22.3) | 33 (29.5) | 0.22 | |
Pack-years | 32.29 [14.8–40] | 32.5 [14.8–40] | 31.28 [15.5–47.5] | 0.38 | 30 [10.9–40] | 40 [20–46] | 0.58 | |
Clubbing of fingers | 61 (27.2) | 30 (26.8) | 31 (27.7) | 0.88 | 29 (25.9) | 32 (28.6) | 0.65 | |
ANA | 131 (58.5) | 67 (59.8) | 64 (57.1) | 0.68 | 66 (58.9) | 65 (58.0) | 0.89 | |
Lung function | ||||||||
FVC% pred. | 86.7 [71.6–102.5] | 86.7 [70.1–103.4] | 86.10 [72.7–100.9] | 0.77 | 87.2 [73.4–104.7] | 84.3 [71.3–97.2] | 0.09 | |
DLCO% pred. | 58.1 [45.9–70.3] | 59.65 [49.2–72.65] | 54.85 [44.0–67.25] | 0.01 | 60.3 [50.8–74.4] | 52.6 [42.4–65.2] | <0.001 | |
HRCT | ||||||||
Honeycombing | 51 (22.8) | 17 (15.2) | 34 (30.4) | 0.007 | 20 (17.9) | 31 (27.7) | 0.08 | |
Emphysema | 22 (9.8) | 10 (8.9) | 12 (10.7) | 0.65 | 6 (5.4) | 16 (14.3) | 0.03 | |
Bronchiectasis | 108 (48.2) | 49 (43.8) | 59 (52.7) | 0.18 | 47 (42.0) | 61 (54.5) | 0.06 | |
Comorbidities | ||||||||
Gastroesophageal reflux | 53 (23.7) | 26 (23.2) | 27 (24.1) | 0.88 | 24 (21.4) | 29 (25.9) | 0.43 | |
Pulmonary hypertension | 30 (13.4) | 10 (8.9) | 20 (17.9) | 0.050 | 4 (3.6) | 26 (23.2) | <0.001 | |
Diabetes mellitus | 55 (24.6) | 24 (21.4) | 31 (27.7) | 0.28 | 29 (25.9) | 26 (23.2) | 0.64 | |
Coronary heart disease | 27 (12.1) | 10 (8.9) | 17 (15.2) | 0.15 | 14 (12.5) | 13 (11.6) | 0.84 | |
Osteoporosis | 77 (34.4) | 34 (30.4) | 43 (38.4) | 0.21 | 40 (35.7) | 37 (33.0) | 0.67 | |
Treatment | ||||||||
Glucocorticoids | 203 (90.6) | 103 (92.0) | 100 (89.3) | 0.49 | 99 (88.4) | 104 (92.9) | 0.25 | |
Immunosuppressants | 178 (79.5) | 90 (80.4) | 88 (78.6) | 0.74 | 88 (78.6) | 90 (80.4) | 0.74 | |
Anti-fibrotic treatment | 41 (18.3) | 15 (13.4) | 26 (23.2) | 0.06 | 15 (13.4) | 26 (23.2) | 0.057 | |
Fibrosis progression | 63 (28.1) | 20 (17.9) | 43 (38.4) | 0.001 | 24 (21.4) | 39 (34.8) | 0.03 | |
Death | 34 (15.2) | 7 (6.3) | 27 (24.1) | <0.001 | 15 (13.4) | 19 (17.0) | 0.89 | |
Acute exacerbation | 58 (25.9) | 17 (15.2) | 41 (36.6) | <0.001 | 20 (17.9) | 38 (33.9) | 0.005 | |
Follow-up duration, months | 40 [29–52] | 40 [31–52] | 38 [21–52] | 0.32 | 41 [31–53] | 38 [27–50] | 0.07 |
Data are presented as mean (standard deviation), median [first and third quartiles], number (%), or number. CTD, connective tissue disease; ILD, interstitial lung disease; CEA, carcinoembryonic antigen; CA125, carbohydrate antigen 125; BMI, body mass index; ANA, antinuclear antibodies; FVC% pred., percent predicted for forced vital capacity; DLCO% pred., percent predicted for diffusion capacity of the lung for carbon monoxide; HRCT, high resolution computed tomography.
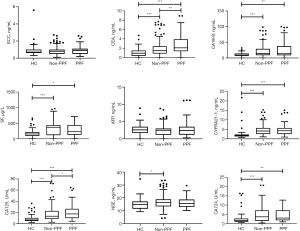
Patients were stratified into low-titre and high-titre groups based on the median levels of CEA or CA125 of CTD-ILD. Patients with elevated CEA levels were older (P=0.001) and exhibited a lower baseline DLCO% pred. (P=0.01) than those with lower CEA levels. The percentage of honeycombing on HRCT (P=0.007) was higher in the high CEA group compared to the low CEA group. In the low CEA group, 20 (17.9%) patients experienced PPF, whereas 43 (38.4%) patients in the high CEA group did (P=0.001). In the high CEA group, the percentages for all-cause mortality and AE were 29.3% and 37.6%, respectively, compared to 8.2% and 15.6% in the low CEA group (both P<0.001). Patients in the high CA125 group, compared to those with low CA125 levels, were older (P=0.007), had a lower baseline DLCO% pred. (P<0.001), more emphysema on HRCT (P=0.03), and higher percentages of PH (P<0.001). Patients in the high CA125 group experienced worse fibrosis progression (P=0.03) and AE (P=0.005) compared to those in the low CA125 group.
Correlations between serum tumor markers and lung function values
Spearman analysis revealed that serum CEA level was negatively correlated with DLCO% pred. (r=−0.213, P=0.001). Serum CA125 level was negatively correlated with DLCO% pred. (r=−0.282, P<0.001) (available online: https://cdn.amegroups.cn/static/public/jtd-24-922-1.docx).
Baseline tumor markers and the diagnostic value for progressive fibrosis
The area under the ROC curve (AUC) for CEA was 0.64 [95% confidence interval (CI): 0.56–0.72], the highest among the tested tumor markers, with an estimated cut-off of 1.79 ng/mL (Figure 3). For CA125, the AUC was 0.59 (95% CI: 0.51–0.68), with an estimated cut-off of 23.8 U/mL. At these cut-off values, CEA demonstrated a sensitivity of 61.90% and a specificity of 62.11% for diagnosing PPF, while CA125 showed a sensitivity of 63.49% and a specificity of 52.17% for diagnosing PPF. Logistic regression analysis was conducted to assess the ability of CEA and CA125 to identify PPF in CTD-ILD patients (Table 2). In the categorical Model 2, patients in the high CEA group, compared to the low reference group, had an odds ratio (OR) of 2.58 (95% CI: 1.36–4.92, P=0.004) for PPF. In the continuous Model 2, doubling of CEA was linked to a 1.74-fold (95% CI: 1.13–2.68) adjusted increase in the risk of PPF (P=0.01). In the RCS model, a linear dose-response relationship of CEA with PPF risk was established (Pnon-linear=0.16, Figure 4). A higher CA125 concentration was significantly associated with PPF in the categorical Model 1 (OR 1.96, 95% CI: 1.08–3.55, P=0.03) and continuous Model 1 (OR 1.41, 95% CI: 1.01–1.97, P=0.043). However, the high CA125 group had an OR of 1.88 (95% CI: 0.98–3.63) for PPF with a non-significant trend (P=0.059) in the categorical Model 2, and an OR of 1.39 (95% CI: 0.97–2.00) with a non-significant trend (P=0.08) in the continuous Model 2.
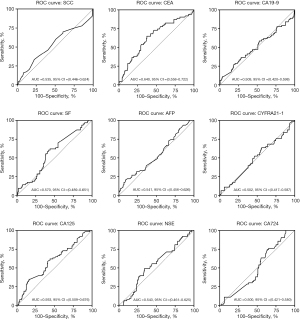
Table 2
Variables | Categorical | Continuous | |||||||||
---|---|---|---|---|---|---|---|---|---|---|---|
Model 1 | Model 2 | Model 1 | Model 2 | ||||||||
OR (95% CI) | P value | OR (95% CI) | P value | OR (95% CI) | P value | OR (95% CI) | P value | ||||
CEA | 3.42 (1.74–6.72) | <0.001 | 2.58 (1.36–4.92) | 0.004 | 1.87 (1.19–2.93) | 0.006 | 1.74 (1.13–2.68) | 0.01 | |||
CA125 | 1.96 (1.08–3.55) | 0.03 | 1.88 (0.98–3.63) | 0.059 | 1.41 (1.01–1.97) | 0.043 | 1.39 (0.97–2.00) | 0.08 |
Model 1: crude; Model 2: adjusted for DLCO% pred., age, pulmonary hypertension, honeycombing, emphysema. CEA, carcinoembryonic antigen; CA125, carbohydrate antigen 125; PPF, progressive pulmonary fibrosis; OR, odds ratio; CI, confidence Interval; DLCO% pred., percent predicted for diffusion capacity of the lung for carbon monoxide.
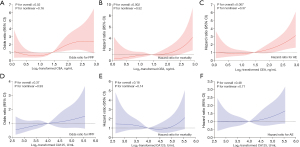
Serum tumor markers associated with prognosis
Stratified survival analysis by CEA and CA125 levels was performed. Kaplan-Meier curves demonstrated a significant difference between the low and high CEA group (P<0.001) (available online: https://cdn.amegroups.cn/static/public/jtd-24-922-1.docx). Furthermore, cumulative hazard curves showed AE rates among different CEA (P<0.001) and CA125 (P=0.005) levels differed significantly (available online: https://cdn.amegroups.cn/static/public/jtd-24-922-1.docx). Cox analysis revealed that CEA was a risk factor for both all-cause mortality and AE in both categorical and continuous Model 1 and Model 2 (Tables 3,4). Regarding CA125, in the continuous Model 1, doubling of CA125 was associated with a 1.46-fold (95% CI: 1.01–2.12, P=0.046) increased risk of mortality, but this association was not observed in other models. Nevertheless, CA125 continued to be a risk factor for AE in the categorical and continuous Model 1, but this association was not observed in the Model 2.
Table 3
Variables | Categorical | Continuous | |||||||||
---|---|---|---|---|---|---|---|---|---|---|---|
Model 1 | Model 2 | Model 1 | Model 2 | ||||||||
HR (95% CI) | P value | HR (95% CI) | P value | HR (95% CI) | P value | HR (95% CI) | P value | ||||
CEA | 3.92 (1.70–9.05) | 0.001 | 2.59 (1.10–6.12) | 0.03 | 3.30 (2.07–5.27) | <0.001 | 2.38 (1.45–3.90) | 0.001 | |||
CA125 | 1.36 (0.68–2.71) | 0.38 | 0.71 (0.33–1.55) | 0.39 | 1.46 (1.01–2.12) | 0.046 | 1.43 (0.72–1.81) | 0.57 |
Model 1: crude; Model 2: adjusted for DLCO% pred., age, pulmonary hypertension, honeycombing, emphysema. CEA, carcinoembryonic antigen; CA125, carbohydrate antigen 125; HR, hazard ratio; CI, confidence interval; DLCO% pred., percent predicted for diffusion capacity of the lung for carbon monoxide.
Table 4
Variables | Categorical | Continuous | |||||||||
---|---|---|---|---|---|---|---|---|---|---|---|
Model 1 | Model 2 | Model 1 | Model 2 | ||||||||
HR (95% CI) | P value | HR (95% CI) | P value | HR (95% CI) | P value | HR (95% CI) | P value | ||||
CEA | 2.66 (1.51–4.69) | 0.001 | 2.00 (1.12–3.59) | 0.02 | 2.10 (1.49–2.95) | <0.001 | 1.77 (1.22–2.56) | 0.003 | |||
CA125 | 2.12 (1.23–3.64) | 0.007 | 1.44 (0.79–2.65) | 0.24 | 1.50 (1.12–1.99) | 0.006 | 1.24 (0.89–1.72) | 0.21 |
Model 1: crude; Model 2: adjusted for DLCO% pred., age, pulmonary hypertension, honeycombing, emphysema. AE, acute exacerbation; CA125, carbohydrate antigen 125; CEA, carcinoembryonic antigen; CI, confidence interval; HR, hazard ratio; DLCO% pred., percent predicted for diffusion capacity of the lung for carbon monoxide.
Subgroup analyses
In exploratory subgroup analyses, the proportional effect of elevated CEA concentrations on PPF remained consistent across all pre-specified subgroups, with a P value for interaction >0.05 (available online: https://cdn.amegroups.cn/static/public/jtd-24-922-1.docx). Subgroup analysis of diagnoses indicated a potential association between elevated CEA levels and PPF within the IIM (P=0.045) and UCTD (P=0.01) subgroups (available online: https://cdn.amegroups.cn/static/public/jtd-24-922-1.docx).
Sensitivity analyses
After excluding acute worsening (available online: https://cdn.amegroups.cn/static/public/jtd-24-922-1.docx), a higher CEA concentration was associated with PPF (OR 1.67, 95% CI: 1.05–2.66, P=0.03). After excluding patients with emphysema (available online: https://cdn.amegroups.cn/static/public/jtd-24-922-1.docx), a higher CEA concentration was still associated with PPF (OR 1.76, 95% CI: 1.12–2.76, P=0.02).
Discussion
The early detection of PPF in patients with CTD-ILDs is of paramount importance, given its detrimental impact on prognostic outcomes. Early anti-fibrosis therapy may benefit these patients. The identification of PPF necessitates long-term monitoring, involving various factors such as clinical symptoms, lung function tests, and HRCT images over a period of at least 12 months (12). However, the current assessment tools, namely HRCT and PFT, may not be universally optimal for all patients. Certain factors, such as potential radiation exposure, healthcare costs, and the presence of severe comorbidities, may hinder the widespread application of HRCT and PFT in clinical practice, particularly in elderly, critically ill, or prolonged monitoring patients. Thus, essential and easily accessible biomarkers are needed for early PPF identification and prognostic assessment.
Previous research on RA demonstrated heightened levels of CA19-9, CA125, and CEA, all of which correlated with onset and disease severity of ILD (30). Likewise, in pSS-ILD, elevated levels of NSE, CEA, CA125, and CA15-3 were observed and these markers were significantly related to the increased risk of ILD in patients with pSS (31). Patients with IIM-ILD also exhibited elevated CEA, CA15-3, CA19-9 and ferritin levels (32). CEA and ferritin could be useful indicators to recognize patients with rapidly progressive ILD from dermatomyositis–associated ILD (33). These studies suggested that tumor markers might play an important role in rheumatic disease and serve as a biomarker to describe the disease severity. Notably, no prior study has explored these differences in tumor marker expression in the context of PPF in CTD-ILD. Our study identified a significant elevation in CEA levels among patients with CTD-associated PPF and elevated CEA concentration was an independent risk factor associated with PPF. Subsequently, we examined the potential relationship between CEA and mortality or AE in patients with CTD-ILD. The serum CEA level was found to be significantly higher in 82 CTD-ILD patients compared to 82 controls without ILD or CTD, and it correlated with disease severity and poor survival (26). Moreover, in waitlisted transplant patients with IPF, elevated serum CEA showed a significant association with an increased risk of mortality (33). Another single-center retrospective study involving 304 hospitalized coronavirus disease 2019 (COVID-19) patients identified CEA as the sole laboratory indicator showing correlation with mortality (34). In our study, an elevated CEA level exhibited an association with prognosis, suggesting its potential as a valuable prognostic marker.
In this study, we also observed a negative correlation between serum CEA levels and DLCO% predicted, suggesting a connection between pulmonary fibrosis tissue and elevated CEA concentrations. Abnormal epithelial proliferation has been linked to pulmonary fibrosis (35). During fibrosis, epithelial cells can undergo epithelial-mesenchymal transition, morphologically changing from regular cuboidal, columnar, or squamous forms to fibroblast-like states, altering cytoskeletal structure and adhesion molecule expression (26,36). CEA, produced by the CEACAM5 gene, mediates homotypic and heterotypic cell-to-cell adhesion (23). Interestingly, CEACAM-positive monocytes increased in peripheral blood of SSc-ILD patients and correlated with higher serum KL-6 and reduced lung function (23). CEA concentration also increased in IPF patients, correlating with disease severity (18). In a bleomycin-induced lung fibrosis model, CEACAM1-positive monocytes infiltrated lung interstitium (23). A lung biopsy from an IPF patient displayed strong CEA staining in metaplastic epithelium lining honeycombed cysts and respiratory bronchioles (23). CEA also rose in bronchoalveolar lavage fluid from idiopathic interstitial pneumonia patients, correlating with neutrophil proportions in bronchoalveolar lavage fluid, suggesting an association with inflammatory activity (37). Collectively, these findings imply CEA’s potential role in pulmonary fibrosis pathogenesis. CEA’s involvement in adhesion, relation with monocytes and epithelial injury, suggests a role in cell-to-cell interaction dysregulation and tissue remodeling characteristic of pulmonary fibrosis.
Another elevated marker detected is CA125 in our study. A higher CA125 concentration was associated with PPF and AE in the Model 1, but this association was not observed in the Model 2. A previous study identified elevated baseline levels of CA19-9 and CA125 in a prospective cohort of 312 IPF patients (38). Furthermore, an increase in CA125 concentrations over three months showed a correlation with an elevated mortality risk. Immunohistochemical analysis localized CA125 and CA19-9 to the bronchial epithelium in healthy lung tissue, with increased presence in fibrotic lung tissue and heightened mucous secretion (38). Additionally, the MUC5B gene stands out as a prominent genetic risk factor for IPF (39). One explanation is that mucin-related biomarkers may represent proxies for the MUC5B genotype, with both CA19-9 and CA125 identifying mucous-associated antigens (40). Nonetheless, our analysis did not observe strong correlations between CA125 and prognosis. A possible explanation for this discrepancy is because of the small sample size of the CTD-ILD group. Another potential explanation is that CA125 is released from the pleura and peritoneum according to immunohistochemical studies, which could account for the inadequacy in predicting the severity of IPF (40).
Additionally, a positive correlation between CA125 levels and PH was observed. Previous studies have linked CA125 to various aspects of PH, including functional status, echocardiography findings, and hemodynamics (41). It has been identified as an independent predictor of 1-year clinical worsening in PH (42). Increased venous system pressure and inflammation induced in the pulmonary bed are common in the pathophysiology of PH (43). Mechanical stress and inflammation are recognized as primary triggers for the release of CA125 from epithelial surfaces, potentially explaining its elevation in PH patients (42). However, conclusive evidence necessitates long-term prospective studies.
Several limitations warrant consideration when interpreting the results. Firstly, the study was based in a single center with a small sample size, including relatively few RA-ILD patients. As a result, our data may not fully represent the broader CTD-ILD population, potentially limiting the generalizability of our findings. Secondly, our cohort exhibited a relatively high proportion of censored data due to the substantial number of patients still alive at the end of follow-up. This imbalance may impact the statistical power of the study. Thirdly, our study primarily focused on investigating the relationship between baseline tumor marker concentrations and prognosis. Only a small number of patients provided data on longitudinal markers; in the PPF group, CEA had a tendency to increase, but the difference was not statistically significant (available online: https://cdn.amegroups.cn/static/public/jtd-24-922-1.docx). The limited longitudinal data may hinder our ability to detect evolving trends and lead to potentially the missing of additional associations. Moreover, although we observed a potential link between CEA levels and fibrosis, the underlying mechanisms remain unclear and warrant further investigation. Additionally, as our study focuses on CTD-ILD patients, the significance of tumor markers in patients with CTD but no ILD remains uncertain. Some patients also exhibited combined signs of emphysema and fibrosis on HRCT, which could affect lung function measurements (44). The compensatory effects of emphysema and fibrosis might result in normal spirometry outcomes and no significant change in FVC (45). Acknowledging these limitations, we seek a balanced interpretation, encouraging further investigations with an expanded sample size and inclusion of multiple study centers to fortify scientific knowledge in this field.
Conclusions
This study aimed to identify non-invasive serum tumor markers as potential biomarkers in CTD-ILD, enabling early detection of PPF and improved disease management. Significant correlations emerged between CEA levels, pulmonary fibrosis progression, AE, and mortality in CTD-ILD patients, suggesting CEA’s potential as a valuable biomarker (available online: https://cdn.amegroups.cn/static/public/jtd-24-922-1.docx). However, further investigations are required to fully understand the underlying mechanisms driving these associations.
Acknowledgments
We thank all participants in this study.
Funding: The work was supported by
Footnote
Reporting Checklist: The authors have completed the STARD reporting checklist. Available at https://jtd.amegroups.com/article/view/10.21037/jtd-24-922/rc
Data Sharing Statement: Available at https://jtd.amegroups.com/article/view/10.21037/jtd-24-922/dss
Peer Review File: Available at https://jtd.amegroups.com/article/view/10.21037/jtd-24-922/prf
Conflicts of Interest: All authors have completed the ICMJE uniform disclosure form (available at https://jtd.amegroups.com/article/view/10.21037/jtd-24-922/coif). The authors have no conflicts of interest to declare.
Ethical Statement: The authors are accountable for all aspects of the work in ensuring that questions related to the accuracy or integrity of any part of the work are appropriately investigated and resolved. This study was conducted in accordance with the principles of the Declaration of Helsinki (as revised in 2013). The protocol of this study was approved by the Institutional Review Board of Beijing Chao-Yang Hospital (2022-KY-529) and written informed consent was obtained from all participants.
Open Access Statement: This is an Open Access article distributed in accordance with the Creative Commons Attribution-NonCommercial-NoDerivs 4.0 International License (CC BY-NC-ND 4.0), which permits the non-commercial replication and distribution of the article with the strict proviso that no changes or edits are made and the original work is properly cited (including links to both the formal publication through the relevant DOI and the license). See: https://creativecommons.org/licenses/by-nc-nd/4.0/.
References
- Storrer KM, Müller CS, Pessoa MCA, et al. Connective tissue disease-associated interstitial lung disease. J Bras Pneumol 2024;50:e20230132. [Crossref] [PubMed]
- Kondoh Y, Bando M, Kawahito Y, et al. Identification and management of interstitial lung disease associated with systemic sclerosis (SSc-ILD), rheumatoid arthritis (RA-ILD), and polymyositis/dermatomyositis (PM/DM-ILD): development of expert consensus-based clinical algorithms. Expert Rev Respir Med 2024;18:447-56. [Crossref] [PubMed]
- Lundberg IE, Tjärnlund A, Bottai M, et al. 2017 European League Against Rheumatism/American College of Rheumatology classification criteria for adult and juvenile idiopathic inflammatory myopathies and their major subgroups. Ann Rheum Dis 2017;76:1955-64. [Crossref] [PubMed]
- Shiboski CH, Shiboski SC, Seror R, et al. 2016 American College of Rheumatology/European League Against Rheumatism classification criteria for primary Sjögren's syndrome: A consensus and data-driven methodology involving three international patient cohorts. Ann Rheum Dis 2017;76:9-16. [Crossref] [PubMed]
- Aringer M, Costenbader K, Daikh D, et al. 2019 European League Against Rheumatism/American College of Rheumatology classification criteria for systemic lupus erythematosus. Ann Rheum Dis 2019;78:1151-9. [Crossref] [PubMed]
- van den Hoogen F, Khanna D, Fransen J, et al. 2013 classification criteria for systemic sclerosis: an American college of rheumatology/European league against rheumatism collaborative initiative. Ann Rheum Dis 2013;72:1747-55. [Crossref] [PubMed]
- Silvério-António M, Martins-Martinho J, Melo AT, et al. Predictors of Interstitial Lung Disease in Mixed Connective Tissue Disease. J Clin Med 2023;12:7481. [Crossref] [PubMed]
- Aletaha D, Neogi T, Silman AJ, et al. 2010 Rheumatoid arthritis classification criteria: an American College of Rheumatology/European League Against Rheumatism collaborative initiative. Arthritis Rheum 2010;62:2569-81. [Crossref] [PubMed]
- Tani C, Trentin F, Parma A, et al. Disease evolution and organ damage accrual in patients with stable UCTD: a long-term monocentric inception cohort. RMD Open 2024;10:e003967. [Crossref] [PubMed]
- Iaccarino L, Gatto M, Bettio S, et al. Overlap connective tissue disease syndromes. Autoimmun Rev 2013;12:363-73. [Crossref] [PubMed]
- Chang SE, Jia G, Gao X, et al. Pursuing Clinical Predictors and Biomarkers for Progression in ILD: Analysis of the Pulmonary Fibrosis Foundation (PFF) Registry. Lung 2024;202:269-73. [Crossref] [PubMed]
- Raghu G, Remy-Jardin M, Richeldi L, et al. Idiopathic Pulmonary Fibrosis (an Update) and Progressive Pulmonary Fibrosis in Adults: An Official ATS/ERS/JRS/ALAT Clinical Practice Guideline. Am J Respir Crit Care Med 2022;205:e18-47. [Crossref] [PubMed]
- Wang Y, Guo Z, Ma R, et al. Prognostic Predictive Characteristics in Patients With Fibrosing Interstitial Lung Disease: A Retrospective Cohort Study. Front Pharmacol 2022;13:924754. [Crossref] [PubMed]
- Chai D, Sun D, Wang Y, et al. Progression of radiographic fibrosis in rheumatoid arthritis-associated interstitial lung disease. Front Med (Lausanne) 2023;10:1265355. [Crossref] [PubMed]
- Wang H, Wang Y, Sun D, et al. Progressive pulmonary fibrosis in myositis-specific antibody-positive interstitial pneumonia: a retrospective cohort study. Front Med (Lausanne) 2023;10:1325082. [Crossref] [PubMed]
- Mira-Avendano I, Kaye M. Key learnings from the INBUILD trial in patients with progressive pulmonary fibrosis. Ther Adv Respir Dis 2024;18:17534666241266343. [Crossref] [PubMed]
- Yang S, Zhan X, Wang J, et al. Serum Oncomarkers in Patients with MPO-ANCA-Positive Vasculitis: Diagnostic and Prognostic Predictive Values for Interstitial Lung Disease. Lung 2022;200:331-8. [Crossref] [PubMed]
- Fahim A, Crooks MG, Wilmot R, et al. Serum carcinoembryonic antigen correlates with severity of idiopathic pulmonary fibrosis. Respirology 2012;17:1247-52. [Crossref] [PubMed]
- Hirose H, Higuchi T, Takagi K, et al. Association of elevated serum carbohydrate antigen 19-9 levels with extensive interstitial lung disease in patients with systemic sclerosis: A cross-sectional study. Int J Rheum Dis 2024;27:e14978. [Crossref] [PubMed]
- Zhang XY, Hong LL, Ling ZQ. MUC16: clinical targets with great potential. Clin Exp Med 2024;24:101. [Crossref] [PubMed]
- Wang Y, Zhang J, Liu Y, et al. Realveolarization with inhalable mucus-penetrating lipid nanoparticles for the treatment of pulmonary fibrosis in mice. Sci Adv 2024;10:eado4791. [Crossref] [PubMed]
- Lefebvre AM, Adam J, Nicolazzi C, et al. The search for therapeutic targets in lung cancer: Preclinical and human studies of carcinoembryonic antigen-related cell adhesion molecule 5 expression and its associated molecular landscape. Lung Cancer 2023;184:107356. [Crossref] [PubMed]
- Yokoyama K, Mitoma H, Kawano S, et al. CEACAM 1, 3, 5 and 6 -positive classical monocytes correlate with interstitial lung disease in early systemic sclerosis. Front Immunol 2022;13:1016914. [Crossref] [PubMed]
- Bao Y, Zhang W, Shi D, et al. Correlation Between Serum Tumor Marker Levels and Connective Tissue Disease-Related Interstitial Lung Disease. Int J Gen Med 2021;14:2553-60. [Crossref] [PubMed]
- Kwon BS, Kim ES, Lim SY, et al. The significance of elevated tumor markers among patients with interstitial lung diseases. Sci Rep 2022;12:16702. [Crossref] [PubMed]
- Jin Q, Zheng J, Xu X, et al. Value of Serum Carbohydrate Antigen 19-9 and Carcinoembryonic Antigen in Evaluating Severity and Prognosis of Connective Tissue Disease-Associated Interstitial Lung Disease. Arch Rheumatol 2018;33:190-7. [Crossref] [PubMed]
- Hu Y, Wang LS, Wei YR, et al. Clinical Characteristics of Connective Tissue Disease-Associated Interstitial Lung Disease in 1,044 Chinese Patients. Chest 2016;149:201-8. [Crossref] [PubMed]
- Petri M, Orbai AM, Alarcón GS, et al. Derivation and validation of the Systemic Lupus International Collaborating Clinics classification criteria for systemic lupus erythematosus. Arthritis Rheum 2012;64:2677-86. [Crossref] [PubMed]
- Koshy K, Barnes H, Farrand E, et al. Steroid therapy in acute exacerbation of fibrotic interstitial lung disease. Respirology 2024;29:795-802. [Crossref] [PubMed]
- Zheng M, Lou A, Zhang H, et al. Serum KL-6, CA19-9, CA125 and CEA are Diagnostic Biomarkers for Rheumatoid Arthritis-Associated Interstitial Lung Disease in the Chinese Population. Rheumatol Ther 2021;8:517-27. [Crossref] [PubMed]
- Shi L, Han XL, Guo HX, et al. Increases in tumor markers are associated with primary Sjögren's syndrome-associated interstitial lung disease. Ther Adv Chronic Dis 2020;11:2040622320944802. [Crossref] [PubMed]
- Wang Q, Gao C, Zhang C, et al. Tumor markers are associated with rapidly progressive interstitial lung disease in adult-dermatomyositis. Clin Rheumatol 2022;41:1731-9. [Crossref] [PubMed]
- Yu WS, Lee JG, Paik HC, et al. Carcinoembryonic antigen predicts waitlist mortality in lung transplant candidates with idiopathic pulmonary fibrosis. Eur J Cardiothorac Surg 2018;54:847-52. [Crossref] [PubMed]
- Huang R, Meng T, Zha Q, et al. The predicting roles of carcinoembryonic antigen and its underlying mechanism in the progression of coronavirus disease 2019. Crit Care 2021;25:234. [Crossref] [PubMed]
- Zuo H, Zhou W, Zhou B, et al. CCDC59 alleviates bleomycin-induced inflammation and pulmonary fibrosis by increasing SP-B and SP-C expression in mice. Int Immunopharmacol 2024;138:112645. [Crossref] [PubMed]
- Lee YJ, Kim M, Kim HS, et al. Administration of Gas6 attenuates lung fibrosis via inhibition of the epithelial-mesenchymal transition and fibroblast activation. Cell Biol Toxicol 2024;40:20. [Crossref] [PubMed]
- Jang HJ, Park MS, Kim YS, et al. The relationship between the severity of pulmonary fibrosis and the lung cancer stage. J Cancer 2021;12:2807-14. [Crossref] [PubMed]
- Maher TM, Oballa E, Simpson JK, et al. An epithelial biomarker signature for idiopathic pulmonary fibrosis: an analysis from the multicentre PROFILE cohort study. Lancet Respir Med 2017;5:946-55. [Crossref] [PubMed]
- Adegunsoye A, Kropski JA, Behr J, et al. Genetics and Genomics of Pulmonary Fibrosis: Charting the Molecular Landscape and Shaping Precision Medicine. Am J Respir Crit Care Med 2024;210:401-23. [Crossref] [PubMed]
- Rusanov V, Kramer MR, Raviv Y, et al. The significance of elevated tumor markers among patients with idiopathic pulmonary fibrosis before and after lung transplantation. Chest 2012;141:1047-54. [Crossref] [PubMed]
- Zhang Y, Jin Q, Zhao Z, et al. Carbohydrate Antigen 125 Is a Biomarker of the Severity and Prognosis of Pulmonary Hypertension. Front Cardiovasc Med 2021;8:699904. [Crossref] [PubMed]
- Sahin A, Kaya H, Avci O. Cancer antigen-125 is a predictor of mortality in patients with pulmonary arterial hypertension. Clin Biochem 2021;89:58-62. [Crossref] [PubMed]
- Stoicescu L, Crişan D, Morgovan C, et al. Heart Failure with Preserved Ejection Fraction: The Pathophysiological Mechanisms behind the Clinical Phenotypes and the Therapeutic Approach. Int J Mol Sci 2024;25:794. [Crossref] [PubMed]
- Calaras D, Mathioudakis AG, Lazar Z, et al. Combined Pulmonary Fibrosis and Emphysema: Comparative Evidence on a Complex Condition. Biomedicines 2023;11:1636. [Crossref] [PubMed]
- Nemoto M, Koo CW, Scanlon PD, et al. Combined Pulmonary Fibrosis and Emphysema: A Narrative Review. Mayo Clin Proc 2023;98:1685-96. [Crossref] [PubMed]