Mesenchymal stem cell treatment alleviates seawater drowning induced lung injury by inhibiting the TNFα/Snail/EMT pathway
Highlight box
Key findings
• A mouse model of seawater drowning induced acute lung injury (SWD-ALI) was established by oral laryngoscopy endotracheal injection (LEI) method. The model conformed to the latest standard (the 2022 Official American Thoracic Society Workshop Report).
• In SWD-ALI, mesenchymal stem cells (MSCs) exert therapeutic effects by inhibiting the tumor necrosis factor alpha (TNFα)/Snail/epithelial-mesenchymal transition (EMT) pathway.
• MSCs improved long-term pulmonary fibrosis caused by SWD.
What is known and what is new?
• In previous studies, cervical tracheotomy or the direct drowning method have commonly been used to establish mouse model of SWD-ALI. MSCs are commonly injected into the tail vein for the treatment of ALI in mice.
• In this study, the LEI method was used to establish the mouse model. The atomization inhalation of MSCs achieved good therapeutic effects by inhibiting the TNFα/Snail/EMT pathway.
What is the implication, and what should change now?
• In this study, for the first time, a mouse model of SWD-ALI was established by LEI method of artificial seawater, and the pulmonary function of the ALI mice was examined. Our findings provide a valuable reference for the study of lung injury under special conditions.
• This study innovatively used the atomized inhalation of MSCs for treatment. Our findings may provide a basis for the development of convenient and practical MSCs nebulized dosage forms.
Introduction
With the development of the marine industry, the incidence of seawater drowning (SWD) continues to increase every year (1,2). The World Health Organization estimated that drowning leads to 372,000 deaths each year, and ranked drowning as the third leading cause of “unintentional injury deaths” worldwide (3-5). SWD is more severe than freshwater drowning, due to its low temperature, hypertonicity, and rich bacterial content (6). Common complications of SWD include acute lung injury (ALI) and acute respiratory distress syndrome (ARDS) (3). The pathogenic mechanisms of SWD-ALI are complex and may involve the inflammatory response, pulmonary edema, decreased alveolar surface-active substances, oxidative stress, apoptosis, and autophagy (7-11). The treatment of SWD-ALI is mainly symptomatic, and includes cardiopulmonary resuscitation, mechanical ventilation, and prophylactic antibiotics (12-14). An absence of previous report or study providing evidence of effective treatments for drowning-related lung injury is a concern (13). Therefore, there is an urgent need for study at the cellular and animal levels to minimize the lethality and disability of SWD-ALI (14).
Tumor necrosis factor (TNF), which is also known as TNFα, is a cellular signaling protein involved in systemic inflammation, is one of the cytokines involved in the acute-phase response, and plays a central role in orchestrating the inflammatory response in mammals (15). In addition, TNFα can disrupt epithelial barrier integrity (16). At the same time, epithelial cells may undergo epithelial-mesenchymal transition (EMT) following injury, further damaging the air-blood barrier and causing lung injury (17). Snail, or SNAI 1, a zinc-finger transcription factor, can bind to E-cadherin to promote EMT (18), and can be stabilized by TNFα, further promoting EMT (19).
Mesenchymal stem cells (MSCs) have antibacterial, anti-inflammatory, and anti-fibrotic properties that promote injury repair, maintain the integrity of the vascular endothelium and barrier epithelium, and have been widely used because of their powerful functions (20-22). MSCs can significantly reduce the level of inflammation in ALI or ARDS (23-27), and they have been shown to be safe and significantly attenuate lung injury in several clinical studies of ARDS (27-30).
In this study, we successfully established mice model of SWD-ALI. Intratracheal administration of MSCs alleviates SWD-ALI by inhibiting the TNFα/Snail/EMT pathway. Further, we found that this treatment managed to effectively limit the progression of pulmonary fibrosis. We present this article in accordance with the ARRIVE and MDAR reporting checklists (available at https://jtd.amegroups.com/article/view/10.21037/jtd-24-1471/rc).
Methods
Artificial seawater was prepared according to the main components of the East China Sea seawater provided by the Oceanic Administration of China (31).
The main reagents and antibody information are listed in Tables S1-S6.
Cell culture
Human umbilical cord MSCs were provided by the Stem Cell and Regenerative Medicine Lab (Beijing, China). The trilineage differentiation potential of the MSCs was confirmed. The expression (of CD29, CD105, CD73, and CD90) and the deletion (of CD45, CD34, CD79a, and HLA-DR) of surface antigens were detected using flow cytometry and analyzed using FlowJo X 10.6.2 software (BD, Franklin Lake, USA).
Human pulmonary alveolar epithelial cells (HPAEpiCs) were provided by the Dalian Institute of Physical Chemistry (Dalian, China) and were cultured in 1640 medium containing 10% fetal bovine serum. After a pre-experimental study, the cells exposed to 30% seawater for 4 h were used as the SWD-ALI model group, and six-generation endogenous MSCs medium was applied to the treatment group.
SWD-ALI model and treatment in mice
Healthy 6–8-week-old male C57BL/6 mice were obtained from the Chinese PLA General Hospital’s Experimental Animal Center (specific pathogen free) (n=87). The random number method was used for grouping. Based on previous studies (9,32-34) or experimental needs, we first completed and characterized the modeling. A small animal nebulizing syringe was then used to nebulize the MSCs via the trachea. All the experimental procedures were approved by the Experimental Animal Welfare Ethics Committee of Chinese PLA General Hospital (No. 2024-X20-69), and adhered to the Guide for the Care and Use of Laboratory Animals (35). A protocol was prepared before the study without registration.
Experimental grouping
SWD-ALI model validation
The control (CON) group comprised normal mice without special intervention. The mice in SWD model (SW) group were treated with 4 mL/kg artificial seawater by laryngoscopy endotracheal injection (LEI) method. Before the experiment, mice were anaesthetized with isoflurane inhalation.
MSCs treatment of SWD induced lung injury
The control (CON) group comprised normal mice who did not undergo any special intervention. After 30 min of SWD, the mice in the SWD + MSC (SW-MSC) group were re-sedated and subsequently injected with 25 µL (5×105 cells) of MSCs by intratracheal inhalation with a nebulizer syringe. The mice in the SWD + phosphate buffer solution (PBS) (SW-PBS) group received an injection of with 25 µL of PBS.
After 6 h from SWD, the animals were euthanized by cervical dislocation and sample collected. If they died, the healthy mice were selected again by random number method (the mortality rate was about 1 in 10).
Micro-CT
The mice were anesthetized via the continuous delivery of isoflurane 2 h after SWD, and scanned using a Quantum FX micro-computed tomography (Micro-CT) imaging system (PerkinElmer, Waltham, USA). Each mouse was scanned for 4 min at 70 kV, 88 µA, and 36 mm field of view parameters. The data acquired from the scans were analyzed and subjected to three-dimensional (3D) reconstruction using Analyze 12.0 software (Analyze Direct, Overland Park, USA) at the same level settings.
Pulmonary function
Pulmonary function was examined 6, 12, 24, 48 h after SWD. The data were collected and analyzed using whole body plethysmography (WBP) produced by Data Sciences International (DSI, St. Paul, USA). Airflow changes (AC) curves were taken from the 2,000 consecutive data between the 5,000th and 7,000th consecutive data from the mice after stabilization, and the data were graphed and analyzed. The mean values of the measured data were used to calculate the minute ventilation (MV) and peak inspiratory flow rate (PIFb) changes in each group.
Wet/dry (W/D) weight ratio of lung samples
The samples were extracted after cervical dislocation, and the lung tissue samples were weighed immediately after being obtained to measure the wet (W) weight. The samples were then dried in an oven at 65 ℃, and the stable dry (D) weight was measured after 72 h of drying. The following formula was used to assess the level of lung edema: W/D × 100%.
Vascular permeability assessment
Evans blue was injected intravenously (30 mL/kg) through the tail vein 1 h before cervical dislocation. The samples were extracted after cervical dislocation and the removed lung tissue was placed into 1 mL of formamide at 37 ℃ for 24 hours. Evans blue was extracted by measuring the absorbance at 620 nm.
Histopathologic staining of lungs and scoring of lung injury
The samples were extracted after cervical dislocation, and the left lobe lung was taken for hematoxylin and eosin (HE) staining, and pictures were taken for lung injury scoring. Under the premise of blindness, Smith’s scoring method (36) was used to analyze the degree of lung injury.
Bronchoalveolar lavage fluid (BALF) collection
The mice were anesthetized to death with isoflurane, intubated via the cervical trachea. Bronchoalveolar lavage was performed with ice PBS. The recovered BALF was centrifuged at 4 ℃ for 10 min at 800 g.
Flow cytometry examination
BALF cells and lung tissue grindings were labeled with CD11b, F4/80, and Ly-6G antibodies for 30 min. Flow cytometry was conducted using a CytoFLEX (Beckman Coulter, Pasadena, USA) flow cytometer to detect macrophages (F4/80+ cells) and neutrophils (CD11b+ Ly-6G+ cells), and analyzed using FlowJo X 10.6.2 software. The antibodies used in the flow cytometry are listed in Table S1.
Enzyme-linked immunosorbent assay (ELISA)
An anti-mouse interleukin-1 beta (IL-1β) and TNFα ELISA Kit (Raybiotech, Guangzhou, China) were used to assess the concentration of inflammatory factors in the BALF of the SWD-ALI in mice.
Western blotting
Lung homogenates were collected, protein concentration was determined, and the proteins were separated by sodium dodecyl-sulfate polyacrylamide gel electrophoresis, and transferred to polyvinylidene fluoride membranes. The antibodies used are listed in Tables S2,S3.
Immunofluorescence analysis
The mouse lung tissues were deparaffinized, dehydrated, closed, and incubated with primary antibody. The tissues were stained using the TSAPLus kit (Servicebio, Wuhan, China). 4,6-Diamidino-2-phenylindole (DAPI) was used to stain cell nuclei, and images were captured using a fluorescence microscope and scanner (Nikon Eclipse C1, 3DHISTECH Pannoramic MIDI, Tokyo, Japan). The antibodies used for the immunofluorescence analysis are listed in Tables S4,S5.
Quantitative real-time polymerase chain reaction (qPCR)
Lung RNA was extracted using TRIzol reagent (Tiangen, Beijing, China) and complementary DNA was synthesized using a reverse transcription kit (Thermo Fisher Scientific, Waltham, USA). QPCR analysis was performed using SYBR (Thermo Fisher Scientific), and the primer sequences are listed in Table S7.
Batch RNA-sequencing
Lung tissue was homogenized using liquid nitrogen milling, avoiding inhomogeneity of damage. RNA from different mouse lungs was extracted separately with TRIzol reagent for evaluation and analysis. Libraries were sequenced and differentially expressed genes (DEGs) were identified with DESeq2 (Bioconductor, Boston, USA) by Annoroad Gene Technology (Beijing, China). Different gene sets were analyzed by Venn diagrams, volcano diagrams, and heat maps, and Gene Ontology (GO) and Kyoto Encyclopedia of Genes and Genomes (KEGG) enrichment analyses that conducted using a Microbiotics-Online Bioinformatics Analysis platform (bioinformatics.com.cn).
Statistical analysis
The data were presented as the mean ± standard error of mean. The between-group comparisons were assessed by the t-test and analysis of variance using SPSS 27 (IBM, Chicago, USA). Bonferroni’s test was used when the variance was homogeneous, and Tamhane’s test was used when the variance was not homogeneous. Correlations were analyzed by Pearson analysis. The significance levels were set as follows: P<0.05, P<0.01, and P<0.001.
Results
SWD-ALI mouse model was successfully established
Following the intratracheal injection of artificial seawater, pulmonary function tests (Figure 1A) showed significant decrease in the respiratory rate and tidal volume, and lung Micro-CT images (Figure 1B) revealed exudative changes (inside the red line). Further, the lung tissue appeared red and swollen at 6 hours post-modeling, and pathological examination revealed hemorrhage, edema, inflammatory cell infiltration, alveolar fracture, and atelectasis with elevated lung injury scores (Figure 1C,1D). Moreover, qPCR testing of the lung tissues revealed decrease in SP-B and SP-C, which maintain alveolar surface tension (Figure 1E), and increase in inflammatory factors IL-1β and IL-6 (Figure 1F). The protein concentration in BALF was also elevated (Figure 1G), and the difference between the CON and SW groups was statistically significant (P<0.01). Therefore, the mouse SWD-ALI model met the evaluation criteria for ALI models in experimental animals proposed by the official symposium of the American Thoracic Society (37), which indicated that the model had been successfully established and thus could be used for further study.
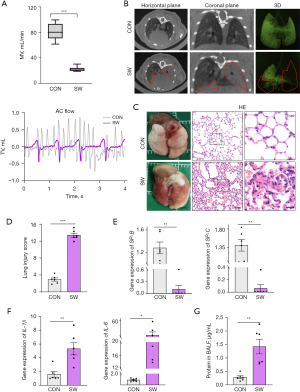
The SWD-ALI mechanism in mice was explored using transcriptome sequencing
The above experiments showed that SWD caused significant lung injury in mice. To understand the mechanism of SWD-ALI, we carried out a series of transcriptome sequencing experiments in the CON and SW mice (Figure 2). The two conditions were analyzed by principal component analysis (PCA), and the results revealed a significant difference in their expression profiles (Figure 2A). We then analyzed the genes that were up- or down-regulated in expression using a volcano plot (Figure 2B). This analysis revealed a significant increase in the transcription of the pro-inflammatory genes post-injury. The top 20 genes with the most significant changes in expression were clustered and analyzed as heat maps (Figure 2C). These genes were mainly involved in regulating inflammatory elements and chemokines.
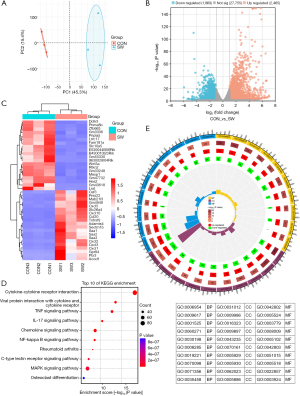
The KEGG pathway enrichment analysis of these genes (Figure 2D) revealed that the most enriched pathways were related to inflammation, including viral infection, and the TNFα, IL-17, NF-κB, and MAPK signaling pathways. Finally, we analyzed the GO terms associated with these genes using a circle plot (Figure 2E). The GO term circle plot analysis of the biological processes, cellular components, and molecular functions, showed that the inflammatory response was mainly concentrated in the cilia assembly, cell surface, and cell basement membrane, which directly led to epithelial cell damage and the alteration of the air-blood barrier, and was also associated with the developmental process of inhalation lung injury. In summary, the main pathology of SWD-ALI is the change in the epithelial cells and basement membrane caused by the inflammatory response in which the TNFα signaling pathway might play an important role.
The TNFα/Snail/EMT pathway mediates SWD-ALI development
The above omics analyses suggested that the TNFα signaling pathway was critical in SWD-ALI. For this study, we used ELISA to measure the TNFα content in BALF of the SWD-ALI mice, and found that the TNFα content was significantly elevated in the SWD-ALI group (Figure 3A).
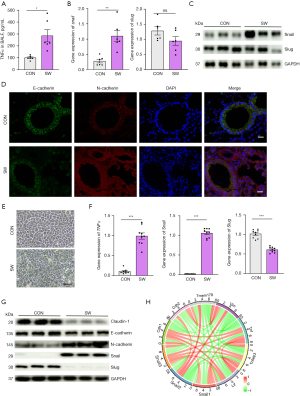
A previous study has shown that TNFα can promote the stabilization of Snail, a transcription factor that can bind to E-cadherin on the cell membrane surface to promote EMT (19). Therefore, we examined the expression changes of the Snail gene and protein levels in lung tissue. We found that the expression of Snail (SNAI 1) was significantly increased (P<0.01), while the expression of Slug (SNAI 2) was decreased in the injured group (P>0.05) (Figure 3B,3C).
To further investigate the role of TNFα in SWD-ALI, we performed immunofluorescence analysis of the lung tissue. We found that the expression of the epithelial cell marker, E-cadherin, was decreased, while the expression of the mesenchymal marker, N-cadherin, was increased (Figure 3D). These results suggest that the elevated TNFα content caused by drowning injury may affect the EMT process by regulating Snail family expression, which in turn may trigger severe lung injury.
To validate this mechanism at the cellular level, we constructed an HPAEpiC-level injury model using 30% artificial seawater stimulation. We found that the cells underwent obvious morphological changes after seawater stimulation (Figure 3E). The qPCR analysis showed that TNFα and Snail messenger RNA (mRNA) levels increased, while the expression of Slug mRNA decreased in cells after seawater stimulation (Figure 3F). Western blot analysis revealed that the protein levels of Snail and N-cadherin increased, while the protein levels of Slug, E-cadherin, and Claudin-1 decreased after seawater stimulation (Figure 3G), suggesting that the seawater stimulation induced the EMT.
To further investigate the correlation between the TNFα, Snail, and EMT signaling pathways, we performed correlation coefficient circle plot analysis of the main genes of these pathways using transcriptome sequencing data. We found a significant correlation between TNFα, Snail, and EMT (Figure 3H), which suggesting that the TNFα/Snail/EMT pathway plays an important role in SWD-ALI.
In conclusion, our results suggest that SWD may mediate the activation of the EMT pathway through the TNFα inflammatory response, leading to severe lung injury.
MSCs therapy effectively improves respiratory symptoms and pulmonary function
A previous study has suggested that MSCs have potent anti-inflammatory effects and might suppress lung injury via TNFα suppression (24). To examine the potential effect of MSCs on SWD-ALI, we identified the morphology and surface markers of MSCs (Figure S1A,S1B). We then nebulized the MSCs intratracheally using a mouse nebulizer syringe (Figure S1C). There was no difference in the MSCs activity before and after MSCs nebulization (Figure S1D). We monitored the respiratory function of the mice using WBP at 6, 12, 24, and 48 h post-treatment. Compared to the mice in the CON group, the mice in the SW-PBS group exhibited wheezing, and hypopnea. However, MSCs improved the symptoms of the SW-MSC mice [supplementary pulmonary function video (Video S1)].
From the 6 and 12 h curves, we derived AC flow diagrams (Figure 4A), which showed lower tidal volume in the model group. MSCs treatment improved respiratory function. In SW-PBS group, MV was worse after injury, but improved after MSCs treatment as shown in SW-MSC group. The improvement was more significant at 12 h in SW-MSC group, but was worse at 12 h in SW-PBS group (Figure 4B). MV was worse 24 and 48 h after SWD, significantly improved after MSCs treatment (Figure 4C,4D). In the SW-PBS group, PIFb worsened after injury, but improved after MSCs treatment as shown in the SW-MSC group. From 6 to 12 h, PIFb changes in line with MV (Figure 4E), and the difference was statistically significant.
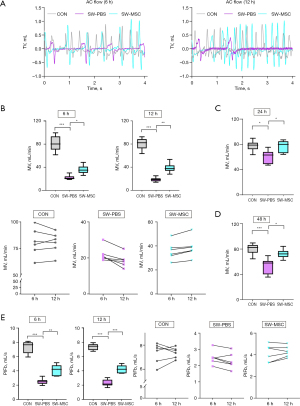
Therefore, our results suggest that the intratracheal nebulization of MSCs can improve respiratory symptoms and pulmonary function in mice with SWD-ALI.
MSCs treatment alleviates SWD-ALI by suppressing TNFα/Snail/EMT signaling
In vivo non-invasive methods confirmed that MSCs effectively treated the SWD-ALI mice. Further analysis at the cellular and tissue levels was performed to establish the therapeutic efficacy of MSCs in treating SWD-ALI. Nebulized inhalation of MSCs was found to significantly mitigate the pathological lung injury in the SWD mice (Figure 5A) and to reduce the systemic inflammatory response (Figure 5B and Figure S2A). The total protein concentration, cell counts, and inflammatory factor IL-1β in BALF of mice were all reduced (Figure S2B). The flow cytometry analysis of the BALF cells and lung tissue cells revealed decrease in neutrophils and macrophages after MSCs inhalation compared to that the mice of SW-PBS group (Figure 5C and Figure S2C). The lung tissue Evans blue passage rate showed decreasing trend (Figure S2D), but the difference between SW-PBS and SW-MSC was not statistically significant (P>0.05). The lungs W/D ratio in SW-MSC group was also reduced (Figure S2E; P<0.05).
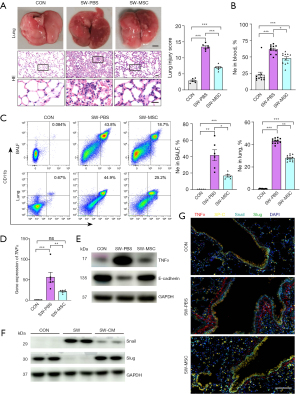
Further, qPCR analysis of the lung tissue revealed reduction in the levels of inflammatory factors IL-1β and IL-6 mRNA after MSCs treatment compared to that post-injury (Figure S2F). The reduction in levels of SP-B and SP-C, and the epithelial sodium channel ENaC were also improved (Figure S2G). These results suggest that the mice of SWD-ALI were ameliorated after MSCs inhalation.
Moreover, the TNFα mRNA and protein levels in mice lung tissues were significantly reduced after MSCs treatment, and E-cadherin protein level was elevated compared with that of SW-PBS mice (Figure 5D,5E). The MSCs-culture medium (CM) treatment decreased the alveolar epithelial cell injury Snail protein level and increased the Slug protein level after seawater stimulation (Figure 5F), indicating that the epithelial cell Snail pathway was inhibited after MSCs treatment. Immunofluorescence detection revealed that TNFα (red) was elevated and SP-C (yellow) was decreased in the SWD-ALI group, which was also accompanied by increase in Snail (cyan) and decrease in Slug (green). However, after MSCs treatment, the opposite effects were observed (Figure 5G). Therefore, it was concluded that MSCs inhibit the TNFα/Snail/EMT pathway and play a therapeutic role in SWD-ALI.
MSCs nebulized inhalation can effectively improve SWD-ALI and chronic pulmonary fibrosis
We investigated the mechanism of MSCs treatment in SWD-ALI through transcriptome sequencing analysis. PCA of transcriptome data indicated significant grouping differences among the groups (i.e., the CON, SW-PBS, and SW-MSC groups) (Figure 6A). The Wayne plot showed 846 common genes in the CON/SW-PBS and SW-PBS/SW-MSC intersections. These genes were primarily inflammatory response genes (Figure 6B). The volcano plot provided additional evidence of the substantial changes in gene expression between the SW-MSC group and SW-PBS group (Figure 6C). The clustering heat map and trend analysis (Figure 6D) showed that MSCs treatment restored extracellular membrane-associated protein, ciliary protein, and viral infection gene expression. It suggested that MSCs improved SWD-ALI at the transcriptome expression level. The first 10 pathways analyzed by KEGG enrichment analysis suggested that MSCs treatment ameliorated SWD-ALI through signaling pathways such as TNFα (Figure 6E).
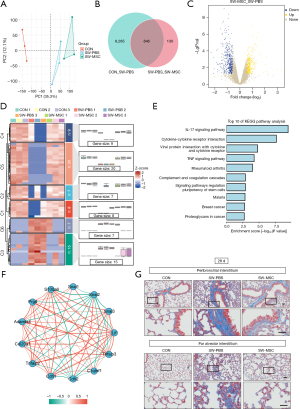
Subsequently, a correlation network diagram (Figure 6F) analysis was performed, which revealed that the major genes of TNFα, Snail, and EMT were correlated with some fibrosis genes. To observe the chronic changes of mouse lung tissue caused by SWD, we performed Masson staining of mouse lung tissue slides after 28 days (Figure 6G), and found that SWD could indeed cause lung tissue fibrosis in mice, and MSCs treatment could alleviate lung interstitial fibrosis in SWD mice.
Thus, these results indicated that MSCs nebulized inhalation can alleviate ALI and chronic pulmonary fibrosis in SWD mice.
Discussion
SWD is a serious medical condition with high morbidity and mortality rates, however, the scientific understanding of the pathogenesis of SWD is limited, as is the development of effective treatment methods. In our study, SWD induced severe acute and chronic lung injury through the activation of the EMT pathway mediated by the TNFα inflammatory response. The inhalation of MSCs via nebulization significantly attenuated ALI and alleviated long-term pulmonary fibrosis in the SWD mice (Figure 7).
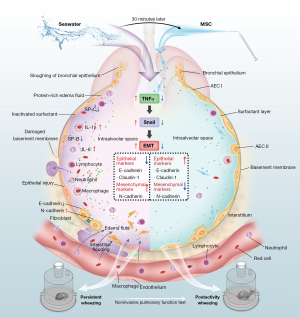
First, we constructed a SWD-ALI mouse model of 4 mL/kg seawater by LEI method for 6 h. After modeling, we successfully validated the model according to the evaluation criteria for ALI models in experimental animals proposed by the ATS Official Symposium (37). The criteria include histological evidence of tissue injury, alteration of the alveolar-capillary barrier, the presence of the inflammatory response, and physiological dysfunction. In terms of the functional evaluation, we innovatively applied the non-invasive pulmonary function test to the mice. The non-invasive pulmonary function test is not commonly used to evaluate ALI animal models (38). However, this method allowed for greater visualization of the activity and respiratory performance of the awake mice. The test data provided insights into mouse respiratory function, including the respiratory curve, tidal volume, and respiratory frequency. In addition, it consistently kept track of lung damage alterations in the mice. More importantly, the SWD-ALI model also revealed that mice lungs developed long-term pulmonary fibrosis after modeling. This suggests that we should pay attention to the longer-term complications of SWD.
Our study showed that the inflammatory response played an important role in model construction, similar to other causes of ALI, such as lipopolysaccharides (LPS), viruses (39-42). The genomics results suggested that the TNFα/Snail/EMT pathway plays an important role in the inflammatory response. The changes in the cell surface and basement membrane suggested the possibility of EMT (43-45), and that EMT may also occur in the acute phase, which is consistent with previous report (17). The question of whether its role is related to the abnormal repair of acute injury requires further study. At the same time, it has been suggested that TNFα drives the stabilization of the Snail protein and suppresses its degradation (19), while the zinc-finger transcription factor Snail protein can bind to E-cadherin and promote EMT (46), which may cause long-term pulmonary fibrosis. Interestingly, elevated Slug has been shown to promote EMT in most studies (47,48). However, Slug expression was decreased in our experiments. The exact relationship between our findings and alveolar collapse, fracture, or specific repair after alveolar cell injury remains unclear and requires further investigation.
Many studies have shown that MSCs can effectively treat ALI caused by LPS and viral infection (24-26,49). To examine whether MSCs were effective in treating SWD-ALI, we investigated the efficacy of administering MSCs via intratracheal nebulized inhalation. The route of administration can help circumvent the pulmonary embolism risks associated with intravenous stem cell administration. MSCs may also play several roles via direct cell-to-cell contact mechanisms and indirectly through the secretion of several anti-inflammatory factors to ALI. Further, we found that MSCs limited the occurrence of late lung fibrosis.
Conclusions
Taken together, early intervention in SWD-ALI events is crucial to reduce the severity of SWD-ALI and the risk of late complications. Our results suggested that MSCs treatment for SWD induced could alleviate ALI and delay later fibrosis. Future research could focus on developing spray devices to deliver highly effective genetically modified MSCs after clearing the airways of SWD patients. To further enhance the therapeutic efficacy of treatments and reduce complications.
Acknowledgments
The authors would like to thank Beijing Institute of Radiation Medicine and Chinese PLA General Hospital for their technical support.
Funding: None.
Footnote
Reporting Checklist: The authors have completed the ARRIVE and MDAR reporting checklists. Available at https://jtd.amegroups.com/article/view/10.21037/jtd-24-1471/rc
Data Sharing Statement: Available at https://jtd.amegroups.com/article/view/10.21037/jtd-24-1471/dss
Peer Review File: Available at https://jtd.amegroups.com/article/view/10.21037/jtd-24-1471/prf
Conflicts of Interest: All authors have completed the ICMJE uniform disclosure form (available at https://jtd.amegroups.com/article/view/10.21037/jtd-24-1471/coif). The authors have no conflicts of interest to declare.
Ethical Statement: The authors are accountable for all aspects of the work in ensuring that questions related to the accuracy or integrity of any part of the work are appropriately investigated and resolved. All the experimental procedures were approved by the Experimental Animal Welfare Ethics Committee of Chinese PLA General Hospital (No. 2024-X20-69), and adhered to the Guide for the Care and Use of Laboratory Animals.
Open Access Statement: This is an Open Access article distributed in accordance with the Creative Commons Attribution-NonCommercial-NoDerivs 4.0 International License (CC BY-NC-ND 4.0), which permits the non-commercial replication and distribution of the article with the strict proviso that no changes or edits are made and the original work is properly cited (including links to both the formal publication through the relevant DOI and the license). See: https://creativecommons.org/licenses/by-nc-nd/4.0/.
References
- Depellegrin D, Venier C, Kyriazi Z, et al. Exploring Multi-Use potentials in the Euro-Mediterranean sea space. Sci Total Environ 2019;653:612-29. [Crossref] [PubMed]
- Kevrekidis DP, Brousa E, Mastrogianni O, et al. Risk factors for fatal drowning in a Greek region: a retrospective case-control study. Inj Prev 2021;27:316-23. [Crossref] [PubMed]
- Jin F, Li C. Seawater-drowning-induced acute lung injury: From molecular mechanisms to potential treatments. Exp Ther Med 2017;13:2591-8. [Crossref] [PubMed]
- Szpilman D, Morgan PJ. Management for the Drowning Patient. Chest 2021;159:1473-83. [Crossref] [PubMed]
- World Health Organization (WHO). Global report on drowning: preventing a leading killer. World Health Organization; 2014.
- Reizine F, Delbove A, Dos Santos A, et al. Clinical spectrum and risk factors for mortality among seawater and freshwater critically ill drowning patients: a French multicenter study. Crit Care 2021;25:372. [Crossref] [PubMed]
- Chen M, Lin H, Gao Y, et al. Ghrelin attenuates drowning injury via dual effects on damage protection and immune repression. Ann Transl Med 2021;9:920. [Crossref] [PubMed]
- Sun XQ, Wu C, Qiu YB, et al. Heme oxygenase-1 attenuates seawater drowning-induced acute lung injury through a reduction in inflammation and oxidative stress. Int Immunopharmacol 2019;74:105634. [Crossref] [PubMed]
- Qiu YB, Wan BB, Liu G, et al. Nrf2 protects against seawater drowning-induced acute lung injury via inhibiting ferroptosis. Respir Res 2020;21:232. [Crossref] [PubMed]
- Legaz I, Barrera-Pérez E, Sibón A, et al. Combining Oxidative Stress Markers and Expression of Surfactant Protein A in Lungs in the Diagnosis of Seawater Drowning. Life (Basel) 2023;13:159. [Crossref] [PubMed]
- Bo L, Li Y, Liu W, et al. Selective inhibition of JNK mitochondrial location is protective against seawater inhalation‑induced ALI/ARDS. Mol Med Rep 2021;24:515. [Crossref] [PubMed]
- Pellegrino F, Raffaldi I, Rossi R, et al. Epidemiology, clinical aspects, and management of pediatric drowning. Ital J Pediatr 2023;49:74. [Crossref] [PubMed]
- Thom O, Roberts K, Devine S, et al. Treatment of the lung injury of drowning: a systematic review. Crit Care 2021;25:253. [Crossref] [PubMed]
- Bierens J, Abelairas-Gomez C, Barcala Furelos R, et al. Resuscitation and emergency care in drowning: A scoping review. Resuscitation 2021;162:205-17. [Crossref] [PubMed]
- Huyghe J, Priem D, Bertrand MJM. Cell death checkpoints in the TNF pathway. Trends Immunol 2023;44:628-43. [Crossref] [PubMed]
- Takeuchi O, Akira S. Pattern recognition receptors and inflammation. Cell 2010;140:805-20. [Crossref] [PubMed]
- Xiao K, He W, Guan W, et al. Mesenchymal stem cells reverse EMT process through blocking the activation of NF-κB and Hedgehog pathways in LPS-induced acute lung injury. Cell Death Dis 2020;11:863. [Crossref] [PubMed]
- Lu Y, Liang Y, Zheng X, et al. EVI1 promotes epithelial-to-mesenchymal transition, cancer stem cell features and chemo-/radioresistance in nasopharyngeal carcinoma. J Exp Clin Cancer Res 2019;38:82. [Crossref] [PubMed]
- Wu Y, Deng J, Rychahou PG, et al. Stabilization of snail by NF-kappaB is required for inflammation-induced cell migration and invasion. Cancer Cell 2009;15:416-28. [Crossref] [PubMed]
- Wang F, Li Y, Wang B, et al. The safety and efficacy of mesenchymal stromal cells in ARDS: a meta-analysis of randomized controlled trials. Crit Care 2023;27:31. [Crossref] [PubMed]
- Wang F, Fang B, Qiang X, et al. The efficacy of mesenchymal stromal cell-derived therapies for acute respiratory distress syndrome-a meta-analysis of preclinical trials. Respir Res 2020;21:307. [Crossref] [PubMed]
- Chen W, Zhu Y, Liu R, et al. Screening Therapeutic Effects of MSC-EVs to Acute Lung Injury Model on A Chip. Adv Healthc Mater 2024;13:e2303123. [Crossref] [PubMed]
- Valiukevičius P, Mačiulaitis J, Pangonytė D, et al. Human Placental Mesenchymal Stem Cells and Derived Extracellular Vesicles Ameliorate Lung Injury in Acute Respiratory Distress Syndrome Murine Model. Cells 2023;12:2729. [Crossref] [PubMed]
- Zhu J, Zhou J, Feng B, et al. MSCs alleviate LPS-induced acute lung injury by inhibiting the proinflammatory function of macrophages in mouse lung organoid-macrophage model. Cell Mol Life Sci 2024;81:124. [Crossref] [PubMed]
- Cui E, Lv L, Wang B, et al. Umbilical cord MSC-derived exosomes improve alveolar macrophage function and reduce LPS-induced acute lung injury. J Cell Biochem 2024;125:e30519. [Crossref] [PubMed]
- Wang C, Hezam K, Fu E, et al. In vivo tracking of mesenchymal stem cell dynamics and therapeutics in LPS-induced acute lung injury models. Exp Cell Res 2024;437:114013. [Crossref] [PubMed]
- Lv H, Chen W, Xiang AP, et al. Mesenchymal stromal cells as a salvage treatment for confirmed acute respiratory distress syndrome: preliminary data from a single-arm study. Intensive Care Med 2020;46:1944-7. [Crossref] [PubMed]
- Dutra Silva J, Su Y, Calfee CS, et al. Mesenchymal stromal cell extracellular vesicles rescue mitochondrial dysfunction and improve barrier integrity in clinically relevant models of ARDS. Eur Respir J 2021;58:2002978. [Crossref] [PubMed]
- Zhang X, Wei X, Deng Y, et al. Mesenchymal stromal cells alleviate acute respiratory distress syndrome through the cholinergic anti-inflammatory pathway. Signal Transduct Target Ther 2022;7:307. [Crossref] [PubMed]
- Wick KD, Leligdowicz A, Zhuo H, et al. Mesenchymal stromal cells reduce evidence of lung injury in patients with ARDS. JCI Insight 2021;6:e148983. [Crossref] [PubMed]
- Ma L, Chen X, Wang R, et al. 3,5,4'-Tri-O-acetylresveratrol decreases seawater inhalation-induced acute lung injury by interfering with the NF-κB and i-NOS pathways. Int J Mol Med 2016;37:165-72. [Crossref] [PubMed]
- Cui Y, Wang X, Lin F, et al. MiR-29a-3p Improves Acute Lung Injury by Reducing Alveolar Epithelial Cell PANoptosis. Aging Dis 2022;13:899-909. [Crossref] [PubMed]
- Zhu Y, Han Q, Wang L, et al. Jinhua Qinggan granules attenuates acute lung injury by promotion of neutrophil apoptosis and inhibition of TLR4/MyD88/NF-κB pathway. J Ethnopharmacol 2023;301:115763. [Crossref] [PubMed]
- Xiang GA, Zhang YD, Su CC, et al. Dynamic changes of mononuclear phagocytes in circulating, pulmonary alveolar and interstitial compartments in a mouse model of experimental silicosis. Inhal Toxicol 2016;28:393-402. [Crossref] [PubMed]
- National Research Council (US) Committee for the Update of the Guide for the Care and Use of Laboratory Animals. The National Academies Collection: Reports funded by National Institutes of Health. Washington (DC): National Academies Press (US); 2011.
- Smith KM, Mrozek JD, Simonton SC, et al. Prolonged partial liquid ventilation using conventional and high-frequency ventilatory techniques: gas exchange and lung pathology in an animal model of respiratory distress syndrome. Crit Care Med 1997;25:1888-97. [Crossref] [PubMed]
- Kulkarni HS, Lee JS, Bastarache JA, et al. Update on the Features and Measurements of Experimental Acute Lung Injury in Animals: An Official American Thoracic Society Workshop Report. Am J Respir Cell Mol Biol 2022;66:e1-e14. [Crossref] [PubMed]
- Glaab T, Braun A. Noninvasive Measurement of Pulmonary Function in Experimental Mouse Models of Airway Disease. Lung 2021;199:255-61. [Crossref] [PubMed]
- Bai X, Yang W, Zhao Y, et al. The extracellular cyclophilin A-integrin β2 complex as a therapeutic target of viral pneumonia. Mol Ther 2024;32:1510-25. [Crossref] [PubMed]
- Shad A, Rewell SSJ, Macowan M, et al. Modelling lung infection with Klebsiella pneumoniae after murine traumatic brain injury. J Neuroinflammation 2024;21:122. [Crossref] [PubMed]
- Guo W, Luo J, Zhao S, et al. The critical role of RAGE in severe influenza infection: A target for control of inflammatory response in the disease. Clin Immunol 2024;262:110178. [Crossref] [PubMed]
- Anwar HM, Salem GEM, Abd El-Latief HM, et al. Therapeutic potential of proteases in acute lung injury and respiratory distress syndrome via TLR4/Nrf2/NF-kB signaling modulation. Int J Biol Macromol 2024;267:131153. [Crossref] [PubMed]
- Sieber P, Schäfer A, Lieberherr R, et al. NF-κB drives epithelial-mesenchymal mechanisms of lung fibrosis in a translational lung cell model. JCI Insight 2023;8:e154719. [Crossref] [PubMed]
- Wang K, Wang Y, Cao Y, et al. Lumican is elevated in the lung in human and experimental acute respiratory distress syndrome and promotes early fibrotic responses to lung injury. J Transl Med 2022;20:392. [Crossref] [PubMed]
- Yu Y, Zhu T, Li Y, et al. Repeated intravenous administration of silica nanoparticles induces pulmonary inflammation and collagen accumulation via JAK2/STAT3 and TGF-β/Smad3 pathways in vivo. Int J Nanomedicine 2019;14:7237-47. [Crossref] [PubMed]
- Liu J, Wu Z, Han D, et al. Mesencephalic Astrocyte-Derived Neurotrophic Factor Inhibits Liver Cancer Through Small Ubiquitin-Related Modifier (SUMO)ylation-Related Suppression of NF-κB/Snail Signaling Pathway and Epithelial-Mesenchymal Transition. Hepatology 2020;71:1262-78. [Crossref] [PubMed]
- Kim H, Lee SB, Myung JK, et al. SLUG is a key regulator of epithelial-mesenchymal transition in pleomorphic adenoma. Lab Invest 2022;102:631-40. [Crossref] [PubMed]
- Khan AQ, Hasan A, Mir SS, et al. Exploiting transcription factors to target EMT and cancer stem cells for tumor modulation and therapy. Semin Cancer Biol 2024;100:1-16. [Crossref] [PubMed]
- Yu T, Cui Y, Xin S, et al. Mesenchymal stem cell conditioned medium alleviates acute lung injury through KGF-mediated regulation of epithelial sodium channels. Biomed Pharmacother 2023;169:115896. [Crossref] [PubMed]
(English Language Editor: L. Huleatt)