A single-center retrospective study of the molecular epidemiological characteristics of different Klebsiella pneumoniae infections in northern China
Highlight box
Key findings
• Multidrug-resistant sequence type (ST)11 and hypervirulent ST23 were identified as the local prevalent Klebsiella pneumoniae (K. pneumoniae) strains in a major city in northern China.
• The distribution of the ST11 and ST23 strains was strongly correlated with neurosurgery patients and those with liver abscesses and gallbladder infections, respectively.
• The antibiotic resistance genes rmtB and TEM-1 were significantly correlated with amikacin and β-lactam resistance.
What is known, and what is new?
• ST11 has been reported to be the prevalent K. pneumoniae type in China and East Asia. KPC-2-producing ST11 exhibits carbapenem resistance and is the dominant carbapenem-resistant K. pneumoniae (CRKP) strain transmitting in China.
• We reported on the molecular epidemiological characteristics of K. pneumoniae disseminating in northern China, including the phylogenetic evolution, antibiotic resistance profile, virulence, and pathogenicity, and we were the first to report that ST11 strains co-harboring the KPC-2, rmtB, and TEM-1 genes have been disseminated in Tianjin.
What is the implication, and what should change now?
• Continuous monitoring against multidrug-resistant and highly virulent K. pneumoniae (hvKP) infections is necessary for disease control and prevention.
• The standardized use of antibiotics and precise treatment schemes are needed to reduce drug-induced resistance evolution and lighten the burden place on the healthcare system.
Introduction
K. pneumoniae is a Gram-negative opportunistic pathogen that can cause multiple life-threatening infections in major organs, such as liver abscesses (1), pyelonephritis, pneumonia, and sepsis (2,3). It has been reported that community-acquired K liver abscess has already become a serious clinical problem in Asia, Europe, North America, and many places in rest of the world (1). K. pneumoniae is also a major causative agent of ventilator-associated pneumonia and blood stream infections, which the mortality rate of blood stream infections could be up to 20–30% (4). According to the 2021 National Bacterial Resistance Surveillance Report from the China Antimicrobial Resistance Surveillance System (CARSS), K. pneumoniae has become the second most prevalent nosocomial pathogen in China, and accounts for 20.7% of Gram-negative bacterial infections (http://www.carss.cn/Report). Hospital-acquired multidrug-resistant K. pneumoniae has a global prevalence of about 32.8% (5). Both carbapenem-resistant K. pneumoniae (CRKP) and highly virulent K. pneumoniae (hvKP) strains represent a serious threat to public health. A five-year longitudinal epidemiological study of over one thousand CRKP isolates from 40 medical centers showed that the prevalence of hypervirulent CRKP has increased from 28.2% to 45.7% between 2016 and 2020 in China (6). According to previous meta-analyses, the overall mortality rate of CRKP-infected patients has reached 42.14% (7), and its resistance to multiple drugs often reduces the therapeutic efficacy.
Conversely, hvKP often causes community-acquired infections in healthy individuals (1). Recently, research has detected carbapenem-resistant, hypervirulent K. pneumoniae (CR-hvKP) strains (8-10), which pose significant challenges to clinical treatments. Thus, the genomic characteristics of K. pneumoniae strains should be continuously monitored to provide further insights into their drug resistance and virulence, and promote more effective treatments.
To pursue these objectives, this study employed a high-throughput sequencing method to obtain the genomic information of different K. pneumoniae clinical strains isolated from patients with different types of K. pneumoniae infections including liver abscesses, lung infections and etc. The strains were analyzed by multi-locus sequence typing (MLST), and a phylogenetic tree was constructed based on their genome sequences. Virulence and drug resistance genes such as tet(A), bla LAP-2, qnrS1, 291 aac (3)-IId, dfrA1, and sul2 were also identified and classified. We aim to compare the pathogenicity, virulence, and drug resistance gene distribution of the K. pneumoniae strains isolated to depict its prevalence in a major city in northern China for a better disease control and more treatment plans. We present this article in accordance with the STROBE reporting checklist (available at https://jtd.amegroups.com/article/view/10.21037/jtd-24-1148/rc).
Methods
Bacterial strains
A total of 38 K. pneumoniae isolates were collected from the clinical samples of 30 patients during routine laboratory examinations at The Second Hospital of Tianjin Medical University from February 2022 to March 2023. Patients diagnosed with lung infections, liver abscess, wound infection, blood infection and catheter infections from departments listed in Table 1 were enrolled in this study. The isolates were cultured and stored at –80 ℃ awaiting use. The isolates were recovered on Luria-Bertani (LB) agar plates overnight. Single colonies were picked and cultivated in 2 mL of LB broth at 37 ℃ until the early stationary phase to obtain bacterial cell suspension for sequencing. The study was conducted in accordance with the Declaration of Helsinki (as revised in 2013). This study was approved by the Ethics Committee of The Second Hospital of Tianjin Medical University (No. KY2023K220). Verbal consent was obtained from the patients.
Table 1
Sample No. | Patient No. | Sample type | Infection types | Department | Sequence types |
---|---|---|---|---|---|
K721 | P21-1 | Sputum | Lung infection | Neurosurgery | 11 |
K142 | P21-2 | Blood | Blood infection | Neurosurgery | 11 |
K394 | P30-1 | Sputum | Lung infection | Neurosurgery | 11 |
K56 | P30-2 | Sputum | Lung infection | Neurosurgery | 11 |
K1444 | P30-3 | Sputum | Lung infection | Neurosurgery | 11 |
K380 | P30-4 | Wound secretion | Wound infection | Neurosurgery | 11 |
K57 | P20 | Sputum | Lung infection | Respiratory | 11 |
K2570 | P3-1 | Catheter | Liver abscess | Hepatobiliary and Pancreatic Surgery | 11 |
K174 | P3-2 | Sputum | Lung infection | Hepatobiliary and Pancreatic Surgery | 11 |
K1653 | P3-3 | Pus | Liver abscess | Hepatobiliary and Pancreatic Surgery | 11 |
K64 | P5 | Blood | Blood infection | Neonatology | 15 |
K1538 | P27 | Blood | Blood infection | Traditional Chinese Medicine | 23 |
K2169 | P2-1 | Vitreous aspirate | Eye infection | Ophthalmology | 23 |
K2909H | P8-1 | Sputum | Lung infection | Neurosurgery | 23 |
K2909B | P8-2 | Sputum | Lung infection | Neurosurgery | 23 |
K26 | P9 | Blood | Blood infection | Urology | 23 |
K1689 | P13 | Pus | Liver abscess | Hepatobiliary and Pancreatic Surgery | 23 |
K1241 | P17 | Pus | Liver abscess | Hepatobiliary and Pancreatic Surgery | 23 |
K2308 | P18 | Pus | Liver abscess | Hepatobiliary and Pancreatic Surgery | 23 |
K2013 | P2-2 | Pus | Liver abscess | Hepatobiliary and Pancreatic Surgery | 23 |
K2001 | P6 | Pus | Liver abscess | Breast and Endocrine Surgery | 29 |
K434 | P12 | Blood | Blood infection | Intensive Care Unit | 45 |
K1990 | P29 | Pus | Liver abscess | Hepatobiliary and Pancreatic Surgery | 65 |
K2261 | P22 | Blood | Blood infection | Cardiovascular surgery | 86 |
K1293 | P23 | Sputum | Lung infection | Neurosurgery | 111 |
K617 | P28 | Sputum | Lung infection | Neurosurgery | 252 |
K1351 | P11 | Pus | Liver abscess | Hepatobiliary and Pancreatic Surgery | 268 |
K198 | P4 | Sputum | Lung infection | Neurosurgery | 380 |
K239 | P16 | Pus | Liver abscess | Hepatobiliary and Pancreatic Surgery | 380 |
K897 | P10 | Blood | Blood infection | Cardiology | 412 |
K1353 | P25 | Sputum | Lung infection | Neurosurgery | 412 |
K2994 | P26 | Sputum | Lung infection | Traditional Chinese Medicine | 485 |
K1435 | P14 | Blood | Blood infection | Rheumatology and Immunology | 592 |
K188 | P1 | Sputum | Lung infection | Health Care | 611 |
K2902 | P24 | Blood | Blood infection | Oncology | 791 |
K2520 | P15 | Blood | Blood infection | Intensive Care Unit | 1,266 |
K464 | P7 | Blood | Blood infection | Hematology | 5,781 |
K1824 | P19 | Blood | Blood infection | Intensive Care Unit | Unknown |
‘-1/-2/-3/-4’ labels after the patient numbers indicate isolate numbers from the same patient.
High-throughput sequencing
Cell suspensions of the isolates were centrifuged, and cell pellets were collected for DNA extraction. DNA extraction kits (Vanzyme, Nanjing, China) were used to extract the genomes of the isolates following the standard protocol provided by the manufacturer. DNA (0.2 µg per sample) was used as the input material for the library construction. A sequencing library was generated using the Rapid Plus DNA Lib Prep Kit for Illumina (Cat. No. RK20208) following the manufacturer’s standard protocol. Individual index sequences were then tagged for each sample. Briefly, the genomic DNA samples were fragmented by sonication to an average size of 350 bp. These inserts were then polished, A-tailed, and ligated with the adapters, after which polymerase chain reaction (PCR) amplification was performed. The PCR products were then purified using the AMPure XP system (Beckman, USA). The quality of the library was examined using the 5400 system (Agilent, USA) and measured by quantitative PCR (1.5 nM). The qualified libraries were pooled and sequenced on the Illumina platform to generate paired-end reads with a length of 150 bp. The sequencing data are available under BioProject number of PRJNA1102315.
Bioinformatics analysis
The quality of the raw sequencing data was assessed using FASTQC software and trimmed using Fastp (version 0.19.5) with the default parameters to obtain clean data (11). The clean reads were assembled into contigs using Megahit (version 1.2.9) with the default settings (12). Protein functions were predicted using emapper (version 2.1.12) against the EggNOG databases [http://eggnog-mapper.embl.de, i.e., the Diamond, Kyoto Encyclopedia of Genes and Genomes (KEGG), and the Protein families (Pfam) databases]. The sequences were analyzed by MLST based on the gapA, infB, mdh, pgi, phoE, rpoB, and tonB genes. A phylogenetic tree was constructed using Parsnp (version 1.5.2) (--use-fasttree), and the GCF_000240185.1 genome, which was downloaded from the National Center for Biotechnology Information (NCBI), as the reference. The phylogenetic tree was visualized using the interactive Tree of Life (iToL) online tool (https://itol.embl.de/). A pangenomic analysis of the strains was conducted using Prokka (version 1.13.3) (genus: Klebsiella, species: pneumoniae) for genome annotation and Roary (version 3.13.0) (-e --mafft) for pangenomic analysis with the default settings. Specifically, Prokka was used for the gene annotation, and Roary was used for the pangenomic analysis based on the gff files generated by Prokka. Antimicrobial resistance genes were identified using the Resistance Gene Identifier (RGI) (version 5.2.1) (rgi main --local --clean --include_loose) and the Comprehensive Antibiotic Resistance Database(https://card.mcmaster.ca/) based on an identity >80% and a length >80%. Major virulent genes were identified using Diamond (version 0.9.32) and the Virulence Factor database (http://www.mgc.ac.cn/VFs/) based on an identity >80% and a query-cover >80% (diamond blastx --id 80 --query-cover 80). Pathogenicity was assessed using the PathogenFinder online tool, which is available at http://genomicepidemiology.org/.
Statistical analysis
The statistical analysis was conducted using R version 4.1.1. Correlation between sequence type (ST) type and clinical department was analyzed using chi-square test by ‘chisq.test’ function. Comparison of pathogenicity of ST types was analyzed using Wilcoxon test by ‘wilcox.test’ function. The correlation of antibiotic genes and a resistance was analyzed using ‘cor.test’ function.
Antibiotic resistance test
Isolation and identification, as well as the antimicrobial susceptibility testing (available online: https://cdn.amegroups.cn/static/public/jtd-24-1148-1.xlsx), were conducted using VITEK 2 Compact (BioMerier, France) and drug test cards. The minimum inhibitory concentrations (MICs) against various antibacterial drugs were examined using the broth microdilution method. Drug susceptibility was determined based on the standards and guidelines of the 2019 American Clinical and Laboratory Standards Institute (CLSI) (13).
Results
The MLST and phylogenetic analyses indicated that ST11 and ST23 were prevalent
In total, 38 K. pneumoniae strains were isolated from different departments including neurosurgery department, hepatobiliary and pancreatic surgery department, intensive care unit and etc. (Tables 1,2). Most of these strains were collected from sputum and blood samples (Table 1). High-throughput sequencing was performed to obtain the whole-genome sequences of these isolates. After obtaining the genome assemblies, we first analyzed the pangenome among these isolates to examine any differences among them. A total of 12,072 genes were predicted, among which 3,535 were core genes (>99% of the 38 genomes carried the genes), 591 were soft core genes (95–99% of the 38 genomes carried the genes), 2,071 were shell genes (15–95% of the 38 genomes carried the genes), and 5,875 were cloud genes (0–15% of the 38 genomes carried the genes). These results suggested that these isolates had diverse genetic traits.
Table 2
Sequence type | Clinical departments | Total | χ² | P value | |
---|---|---|---|---|---|
Non-neurosurgery | Neurosurgery | ||||
ST11 | 4 | 6 | 10 | 5.0736 | 0.02 |
Non-ST11 | 22 | 6 | 28 | ||
Total | 26 | 12 | 38 |
ST, sequence type.
We then conducted sequence typing and phylogenetic analyses to investigate the evolutionary distances between these isolates based on their whole-genome sequences. The GCF_000240185.1 genome was used as the reference. As Figure 1 shows, these strains were categorized into two clusters, while the reference genome branched out individually. Cluster 1 was formed by 10 isolates of ST11, while cluster 2 was formed by 28 isolates of various STs, of which ST23 was dominant (accounting for nine of the 28 STs). These results were consistent with those of the phylogenetic and MLST analyses, which indicated that the carbapenemase producing ST11 and hypervirulent ST23 K. pneumoniae were the local prevalent strains.
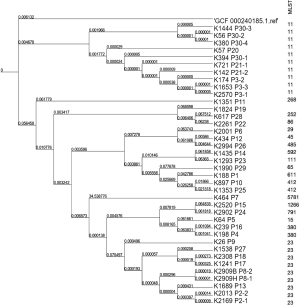
The statistical analysis revealed the prevalent strains dominant in the neurosurgery department, and hepatobiliary and pancreatic surgery department
We then conducted a statistical analysis to investigate whether there was any correlation between the isolates and their origins. The MLST analysis of these 38 K. pneumoniae strains detected 18 known STs (Tables 1,2) and 1 unknown ST. Among all the isolates, there were 10 ST11 strains, accounting for 26.32% of all the STs, and 9 ST23 strains, accounting for 23.68% of all the STs (Table 3).
Table 3
Sequence types | No. of isolates (n=38) | Ratio (%) |
---|---|---|
ST11 | 10 | 26.32 |
ST23 | 9 | 23.68 |
ST380 | 2 | 5.26 |
ST412 | 2 | 5.26 |
ST111 | 1 | 2.63 |
ST1266 | 1 | 2.63 |
ST15 | 1 | 2.63 |
ST252 | 1 | 2.63 |
ST268 | 1 | 2.63 |
ST29 | 1 | 2.63 |
ST45 | 1 | 2.63 |
ST485 | 1 | 2.63 |
ST5781 | 1 | 2.63 |
ST592 | 1 | 2.63 |
ST611 | 1 | 2.63 |
ST65 | 1 | 2.63 |
ST791 | 1 | 2.63 |
ST86 | 1 | 2.63 |
Unknown | 1 | 2.63 |
STs, sequence types.
Among all the K. pneumoniae isolates, 12 (31.58%) strains were collected from the neurosurgery department, while 10 (26.32%) strains were collected from the hepatobiliary and pancreatic surgery department (Table 4). Of the isolates from the neurosurgery department, 50% were ST11 (Table 1). The distribution of ST11 was strongly correlated with the neurosurgery department (chi square test, P=0.02, Table 2). Of the strains collected from the hepatobiliary and pancreatic surgery department, 40% were ST23. Four ST23 strains caused liver abscesses in patients, and while one caused eye infections in patients.
Table 4
Department | No. isolates | Ratio (%) |
---|---|---|
Neurosurgery | 12 | 31.58 |
Hepatobiliary and Pancreatic Surgery | 10 | 26.32 |
Intensive Care Unit (ICU) | 3 | 7.89 |
Traditional Chinese Medicine | 2 | 5.26 |
Rheumatology and Immunology | 1 | 2.63 |
Hematology | 1 | 2.63 |
Oncology | 1 | 2.63 |
Ophthalmology | 1 | 2.63 |
Breast and Endocrine Surgery | 1 | 2.63 |
Urology | 1 | 2.63 |
Neonatology | 1 | 2.63 |
Cardiovascular surgery | 1 | 2.63 |
Cardiology | 1 | 2.63 |
Health Care | 1 | 2.63 |
Respiratory | 1 | 2.63 |
Antibiotic resistance profiling showed that ST11 is the local dominant drug-resistant type
To gain a better understanding of the antibiotic resistance of these K. pneumoniae strains for more precise clinical treatments, we conducted antibiotic gene identification based on their genomes. The results indicated that these strains carried extended-spectrum beta-lactamase genes, including bla-SHV-11, TEM-1, CTX-M-65, and multiple SHV mutants, and a beta-lactamase LAP-2 gene (available online: https://cdn.amegroups.cn/static/public/jtd-24-1148-2.xlsx). Additionally, 23 (60.53%) strains, of which 9 were ST11, carried SHV-11. The positive rate of SHV-11 in ST11 was 90%. The TEM-1 gene was identified in 7 (18.42%) ST11 strains, while CTX-M-65 was detected in 3 (7.89%) ST11 strains.
The carbapenemase resistance gene, bla(KPC-2), was identified in 10 (26.32%) ST11 strains, while OXA-1 was detected in one non-ST11 strain. The aminoglycoside resistance genes rmtA, rmtB, aac3-IId, and aac (6’)-Ib-cr6. RmtA, aac3-IId, and aac (6’)-Ib-cr6 were all identified in 1 non-ST11 strain with an overall positive rate of 2.63%. The rmtB gene was detected in 7 (18.42%) ST11 strains.
In addition, 22 (57.89%) strains, of which 10 were ST11, were found to carry Escherichia coli elongation factor Tu (EF-Tu) mutants that confer resistance to pulvomycin.
The sulfonamide resistance genes sul1, sul2, and dfrA1 were identified in 3 (7.89%) non-ST11 strains, 3 (7.89%) ST11 strains, and 2 (5.26%) non-ST11 strains, respectively.
The quinolone resistance genes, oqxA, oqxB, and qnrS1.oqxA, and oqxB were identified in 33 (86.94%) strains, of which 6 were ST11. Additionally, 7 (18.42%) strains, of which 3 were ST11, carried the qnrS1 gene.
The formycin resistance genes identified were fosA3, fosA5, and fosA6. FosA3 and fosA5 were identified in 3 (7.89%) ST11 strains and 5 (13.16%) non-ST11 strains, respectively. FosA6 was carried by 33 (86.84%) strains, of which 10 strains were ST11.
The tetracycline resistance genes identified were tetA and ramR. TetA gene was carried in 5 (13.16%) strains, of which 3 were ST11. RamR gene was observed in 1 non-ST11 strain.
The gene related to antiseptic resistance, qacEdelta1, was detected in 3 non-ST11 strains.
Moreover, we noted that 7 ST11 strains isolated from P21, P30, and P20 co-harbored the KPC-2, TEM-1, and rmtB genes. The above genetic antibiotic resistance profiles of these K. pneumonia strains showed that the prevalent ST11 has extensive drug resistance. To validate our observation, we then conducted drug susceptibility tests of these isolates.
The drug susceptibility test results of the 38 K. pneumoniae isolates are shown in Table 5. The dominant drug-resistant strain was the prevalent ST11 strain, while the other two drug-resistant strains were ST15 and ST1266. Among all the ST11 strains, 10 exhibited multiple drug-resistance characteristics toward ceftriaxone, cefuroxime, cefoxitin, cefepime, cefoperazone/sulbactam, piperacillin/tazobactam, amoxicillin/clavulanate, levofloxacin, imipenem, and ertapenem. The overall resistance rate to ceftazidime was 90%, while those to amikacin were over 60%. These K. pneumoniae strains exhibited higher susceptibility to the compound cotrimoxazole, as only one strain was resistant to this drug.
Table 5
Antimicrobial drugs | No. of isolates (n=38) | Resistance % | MLST |
---|---|---|---|
Ceftriaxone | 12 | 31.58 | ST11, ST15 |
Cefuroxime | 12 | 31.58 | ST11, ST15 |
Amoxicillin/clavulanate | 11 | 28.95 | ST11, ST1266 |
Levofloxacin | 10 | 26.32 | ST11 |
Imipenem | 10 | 6.32 | ST11 |
Cefoxitin | 10 | 26.32 | ST11 |
Cefoperazone/sulbactam | 10 | 26.32 | ST11 |
Cefepime | 10 | 26.32 | ST11 |
Piperacillin/tazobactam | 10 | 26.32 | ST11 |
Ertapenem | 10 | 26.32 | ST11 |
Ceftazidime | 9 | 23.68 | ST11 |
Amikacin | 7 | 18.42 | ST11 |
Cotrimoxazole | 1 | 2.63 | ST11 |
MLST, multi-locus sequence typing.
The virulence and pathogenicity analysis showed that the prevalent ST23 strains are more pathogenic and hypervirulent
To further investigate the molecular characteristics of these K. pneumoniae strains, we analyzed their virulence gene profiles. Based on the results (Table 6), all of the isolates carried 4 virulence genes on their genomes (i.e., entB, fimH, iutA, and mrkD). Notably, 9 strains, all of which were ST23 strains, carried the allS gene. Additionally, 20 (52.63%) strains carried iroB and rmpA. The IucA gene was found in 21 (55.26%) strains. Additionally, 19 (50%) strains carried the rmpA2 gene, while 30 (78.95%) strains carried the ybtS gene. Further, 9 ST23 strains carried 10 virulence genes (Table 6). In total, 3 ST11 strains carried the iucA, rmpA, and rmpA2 genes; these strains were collected from the same patient at different time points.
Table 6
Isolates | allS | entB | fimH | iroB | iucA | iutA | mrkD | rmpA | rmpA2 | ybtS |
---|---|---|---|---|---|---|---|---|---|---|
ST11 | 0 | 10 | 10 | 0 | 3 | 10 | 10 | 3 | 3 | 10 |
ST23 | 9 | 9 | 9 | 9 | 9 | 9 | 9 | 9 | 9 | 9 |
ST380 | 0 | 2 | 2 | 2 | 2 | 2 | 2 | 2 | 0 | 2 |
ST412 | 0 | 2 | 2 | 2 | 2 | 2 | 2 | 2 | 2 | 0 |
ST111 | 0 | 1 | 1 | 1 | 0 | 1 | 1 | 1 | 0 | 1 |
ST1266 | 0 | 1 | 1 | 0 | 0 | 1 | 1 | 0 | 0 | 1 |
ST15 | 0 | 1 | 1 | 0 | 0 | 1 | 1 | 0 | 0 | 1 |
ST252 | 0 | 1 | 1 | 0 | 0 | 1 | 1 | 0 | 0 | 0 |
ST268 | 0 | 1 | 1 | 1 | 1 | 1 | 1 | 1 | 1 | 1 |
ST29 | 0 | 1 | 1 | 1 | 1 | 1 | 1 | 1 | 1 | 1 |
ST45 | 0 | 1 | 1 | 0 | 0 | 1 | 1 | 0 | 0 | 1 |
ST485 | 0 | 1 | 1 | 0 | 0 | 1 | 1 | 0 | 0 | 1 |
ST5781 | 0 | 1 | 1 | 0 | 0 | 1 | 1 | 0 | 0 | 0 |
ST592 | 0 | 1 | 1 | 1 | 1 | 1 | 1 | 1 | 0 | 0 |
ST611 | 0 | 1 | 1 | 1 | 0 | 1 | 1 | 0 | 1 | 0 |
ST65 | 0 | 1 | 1 | 1 | 1 | 1 | 1 | 0 | 1 | 1 |
ST791 | 0 | 1 | 1 | 0 | 0 | 1 | 1 | 0 | 0 | 0 |
ST86 | 0 | 1 | 1 | 1 | 1 | 1 | 1 | 0 | 1 | 1 |
Unknown | 0 | 1 | 1 | 0 | 0 | 1 | 1 | 0 | 0 | 0 |
Total No. of isolates | 9 | 38 | 38 | 20 | 21 | 38 | 38 | 20 | 19 | 30 |
The pathogenicity analysis predicted pathogenic families carried by each genome, such as siderophore receptor, fimbrial biogenesis outer membrane usher protein, ABC transport system and etc., that could potentially cause a bacteria pathogenic. The results showed that the ST23 strains carried an average of 582.22 pathogenic gene families, while the ST11 strains carried an average of 341.70 pathogenic gene families, and the other ST strains carried an average of 245.19 pathogenic gene families. There was a significant difference between the ST23 and non-ST23 strains (Wilcox test, P<0.001, Appendix 1), which shows that the pathogenicity of the ST23 strains was higher than that of the other STs.
Discussion
According to previous studies, ST11 is the prevalent strain of K. pneumoniae in China and East Asia (14,15). In this study, we showed that the evolutionary characteristics of 38 clinical K. pneumoniae strains in a major city in northern China were consistent with those reported in previous studies (14,15). The ST11 strain exhibits carbapenem resistance and is the main CRKP strain. In recent years, KPC-2-producing K. pneumoniae has been the dominant genotype of CRKP transmitted in China, and accounts for the largest proportion of K. pneumoniae infections in various regions of the country, for which ST11 is the dominant ST (16-18). Consistent with previous research (16-18), we showed that all the ST11 strains included carried the KPC-2 gene. Moreover, one ST1266 strain carried the OXA-1 gene with a MIC of 8 mg/L for piperacillin/tazobactam. Livermore et al. reported that the presence of the OXA-1 gene in Escherichia coli led to an increase in the MIC of piperacillin/tazobactam to 8 mg/L (19). Sugumar et al. showed that 20.3% of piperacillin/tazobactam-resistant K. pneumoniae strains carry OXA-1 (20). The present study showed that the presence of the OXA-1 gene can lead to an increase in piperacillin/tazobactam resistance in K. pneumoniae.
The co-harboring of rmtB and TEM-1 genes on a conjugative plasmid has recently been reported in clinical CRKP isolates (21). TEM-1 confers resistance to β-lactam. The presence of rmtB is significantly associated with amikacin resistance (P<2.2e−16, R2=1). RmtB is a 16S ribosomal RNA methyltransferase favoring resistance to aminoglycoside antibiotics (22,23). Moreover, we noted that the rmtB, TEM1, and KPC-2 genes co-existed in strains isolated from three patients; that is, P21 (K721 and K142), P30 (K394, K56, and K1444, K380), and P20 (K57). Of these three patients, two patients were from the neurosurgery department and one patient was from the respiratory department (available online: https://cdn.amegroups.cn/static/public/jtd-24-1148-2.xlsx). A previous study reported on the transmission of K. pneumoniae strains co-harboring rmtB, TEM1, and KPC-2 in Southern China (24). Klebsiella quasipneumoniae carrying these genes was isolated from urban sewage in Argentina (25). To the best of our knowledge, this is the first study to report on the dissemination of such multi-drug-resistant K. pneumoniae strains in Tianjin, a major city in northern China. Such findings suggest that the on-time monitoring and control of such multidrug-resistant pathogens is needed to prevent their transmission worldwide.
FosA gene was detected in most of the 38 K. pneumoniae strains, and it is known to be associated with fosfomycin resistance (26). QacEΔ1 mediates resistance to triclosan disinfectants in Salmonella and E. coli (27), which suggests that the wide use of disinfectants may place selective pressure.
In this study, four ST11 strains were collected from different samples of the same patient (P30) at different time points. Of these strains, 3 were CR-hvKP and exhibited multidrug-resistant characteristics. It is highly likely that the virulence-encoding conjunctive plasmids, such as the large virulence plasmid pLVPK-like plasmid and multidrug-resistant pKPC CR-HvKP267 plasmid, could play a key role. Antibiotic resistance genes carried by this plasmid include the tet(A) mutant, bla LAP-2, qnrS1, aac (3)-IId, dfrA1, and sul2 (28). After the second admission of the patient (P30), significant changes were observed in the results of the drug susceptibility tests and the genomic characteristics between the K. pneumoniae isolates collected, including decreased resistance and a loss of virulence genes. Based on previous studies, most highly resistant and virulent CRKP K. pneumoniae strains achieve their multidrug resistance and virulence phenotypes by acquiring plasmids or mobile elements (29,30). Drug resistance could be acquired through the formation of the thick capsule of hvKP (31,32). As carrying multiple plasmids places evolutionary pressures on K. pneumoniae, virulence and resistance may be lost to gain an evolutionary advantage in a relative stress-free environment. However, further research is needed to confirm this speculation.
Patients in the neurosurgery department tended to be more prone to CR-KP infections, as they underwent invasive operations, resulting in weaker immune systems. Moreover, there is a higher risk of cross infection among such immunocompromised patients. Only effective prevention and control measures in hospitals can help reduce such incidence.
The ST11 strains were highly resistant, while the other STs identified in this study showed varied resistance, and all of them carried multiple antibiotic resistance genes. Events of horizontal gene transfer frequently occur in the microbial community during massive antibiotic treatment under stressful conditions. However, we did not observe an increase in drug resistance over time among the isolates collected from the same patients at different time points, indicating that the standardized use of antibiotics has reduced the evolutionary pressure.
HvKP is sensitive to commonly used antibacterial drugs; however, it has strong pathogenicity, for which a primary liver abscess is often the initial symptom, and can lead to other symptoms such as intraocular infection (33). Multiple cases of liver abscesses were caused by ST23 with close phylogenetic relationships. Thus, some community-acquired infection events with hvKP might have occurred locally. However, no increase in drug resistance in these isolates was observed.
It is necessary to be vigilant against multidrug-resistant and hvKP infections, which have shown an upward trend, and may be underestimated in China and other places in the world. However, given that the sample size and the area involved in this study were relatively small, and the study was conducted in a short time span. The results have only a local representativeness. Further larger scale studies need to be conducted to continue monitoring K. pneumonia infections in larger region. Moreover, it is necessary to continuously monitor and standardize the use of antibiotics in medical organizations, and adopt precise treatment schemes to reduce drug-induced resistance evolution.
Conclusions
In conclusion, we conducted a retrospective study of the epidemiological and genetic characteristics of multidrug-resistant and hypervirulent K. pneumoniae strains in a major city in northern China. Our findings provide insights into the genetic characteristics and could be a theoretical basis for the prevention and control of K. pneumoniae infections.
Acknowledgments
Funding: This work was supported by
Footnote
Reporting Checklist: The authors have completed the STROBE reporting checklist. Available at https://jtd.amegroups.com/article/view/10.21037/jtd-24-1148/rc
Data Sharing Statement: Available at https://jtd.amegroups.com/article/view/10.21037/jtd-24-1148/dss
Peer Review File: Available at https://jtd.amegroups.com/article/view/10.21037/jtd-24-1148/prf
Conflicts of Interest: All authors have completed the ICMJE uniform disclosure form (available at https://jtd.amegroups.com/article/view/10.21037/jtd-24-1148/coif). Z.C. is from Shenzhen Mindray Bio-Medical Electronics Co., Ltd. The other authors have no conflicts of interest to declare.
Ethical Statement: The authors are accountable for all aspects of the work in ensuring that questions related to the accuracy or integrity of any part of the work are appropriately investigated and resolved. The study was conducted in accordance with the Declaration of Helsinki (as revised in 2013). This study was approved by the Ethics Committee of The Second Hospital of Tianjin Medical University (No. KY2023K220). Verbal consent was obtained from the patients.
Open Access Statement: This is an Open Access article distributed in accordance with the Creative Commons Attribution-NonCommercial-NoDerivs 4.0 International License (CC BY-NC-ND 4.0), which permits the non-commercial replication and distribution of the article with the strict proviso that no changes or edits are made and the original work is properly cited (including links to both the formal publication through the relevant DOI and the license). See: https://creativecommons.org/licenses/by-nc-nd/4.0/.
References
- Jun JB. Klebsiella pneumoniae Liver Abscess. Infect Chemother 2018;50:210-8. [Crossref] [PubMed]
- Xu M, Fu Y, Kong H, et al. Bloodstream infections caused by Klebsiella pneumoniae: prevalence of bla(KPC), virulence factors and their impacts on clinical outcome. BMC Infect Dis 2018;18:358. [Crossref] [PubMed]
- Wang G, Zhao G, Chao X, et al. The Characteristic of Virulence, Biofilm and Antibiotic Resistance of Klebsiella pneumoniae. Int J Environ Res Public Health 2020;17:6278. [Crossref] [PubMed]
- Martin RM, Bachman MA. Colonization, Infection, and the Accessory Genome of Klebsiella pneumoniae. Front Cell Infect Microbiol 2018;8:4. [Crossref] [PubMed]
- Mohd Asri NA, Ahmad S, Mohamud R, et al. Global Prevalence of Nosocomial Multidrug-Resistant Klebsiella pneumoniae: A Systematic Review and Meta-Analysis. Antibiotics (Basel) 2021;10:1508. [Crossref] [PubMed]
- Hu F, Pan Y, Li H, et al. Carbapenem-resistant Klebsiella pneumoniae capsular types, antibiotic resistance and virulence factors in China: a longitudinal, multi-centre study. Nat Microbiol 2024;9:814-29. [Crossref] [PubMed]
- Xu L, Sun X, Ma X. Systematic review and meta-analysis of mortality of patients infected with carbapenem-resistant Klebsiella pneumoniae. Ann Clin Microbiol Antimicrob 2017;16:18. [Crossref] [PubMed]
- Yang X, Sun Q, Li J, et al. Molecular epidemiology of carbapenem-resistant hypervirulent Klebsiella pneumoniae in China. Emerg Microbes Infect 2022;11:841-9. [Crossref] [PubMed]
- Chen L, Kreiswirth BN. Convergence of carbapenem-resistance and hypervirulence in Klebsiella pneumoniae. Lancet Infect Dis 2018;18:2-3. [Crossref] [PubMed]
- Cai Z, Jia T, Pu M, et al. Clinical and Molecular Analysis of ST11-K47 Carbapenem-Resistant Hypervirulent Klebsiella pneumoniae: A Strain Causing Liver Abscess. Pathogens 2022;11:657. [Crossref] [PubMed]
- Chen S, Zhou Y, Chen Y, et al. fastp: an ultra-fast all-in-one FASTQ preprocessor. Bioinformatics 2018;34:i884-90. [Crossref] [PubMed]
- Li D, Liu CM, Luo R, et al. MEGAHIT: an ultra-fast single-node solution for large and complex metagenomics assembly via succinct de Bruijn graph. Bioinformatics 2015;31:1674-6. [Crossref] [PubMed]
- CLSI. Autoverification of Medical Laboratory Results for Specific Disciplines, 1st ed. CLSI guideline AUTO15. Clinical and Laboratory Standards Institute; 2019.
- Wyres KL, Lam MMC, Holt KE. Population genomics of Klebsiella pneumoniae. Nat Rev Microbiol 2020;18:344-59. [Crossref] [PubMed]
- Liao W, Liu Y, Zhang W. Virulence evolution, molecular mechanisms of resistance and prevalence of ST11 carbapenem-resistant Klebsiella pneumoniae in China: A review over the last 10 years. J Glob Antimicrob Resist 2020;23:174-80. [Crossref] [PubMed]
- Hu Y, Liu C, Shen Z, et al. Prevalence, risk factors and molecular epidemiology of carbapenem-resistant Klebsiella pneumoniae in patients from Zhejiang, China, 2008-2018. Emerg Microbes Infect 2020;9:1771-9. [Crossref] [PubMed]
- Zhu X, Yue C, Geng H, et al. Coexistence of tet(A) and bla(KPC-2) in the ST11 hypervirulent tigecycline- and carbapenem-resistant Klebsiella pneumoniae isolated from a blood sample. Eur J Clin Microbiol Infect Dis 2023;42:23-31. [Crossref] [PubMed]
- Weng Y, Wang T, Huang B, et al. Multicenter Study of Colistin Heteroresistance in Carbapenem-Resistant Klebsiella pneumoniae Strains in China. Microbiol Spectr 2023;11:e0221822. [Crossref] [PubMed]
- Livermore DM, Day M, Cleary P, et al. OXA-1 β-lactamase and non-susceptibility to penicillin/β-lactamase inhibitor combinations among ESBL-producing Escherichia coli. J Antimicrob Chemother 2019;74:326-33. [Crossref] [PubMed]
- Sugumar M, Kumar KM, Manoharan A, et al. Detection of OXA-1 β-lactamase gene of Klebsiella pneumoniae from blood stream infections (BSI) by conventional PCR and in-silico analysis to understand the mechanism of OXA mediated resistance. PLoS One 2014;9:e91800. [Crossref] [PubMed]
- Zhu J, Ju Y, Zhou X, et al. Epidemiological characteristics of SHV, cmlv, and FosA6-producing carbapenem-resistant Klebsiella pneumoniae based on whole genome sequences in Jiangsu, China. Front Microbiol 2023;14:1219733. [Crossref] [PubMed]
- Doi Y, Wachino JI, Arakawa Y. Aminoglycoside Resistance: The Emergence of Acquired 16S Ribosomal RNA Methyltransferases. Infect Dis Clin North Am 2016;30:523-37. [Crossref] [PubMed]
- Gao J, Wei X, Yin L, et al. Emergence and Transfer of Plasmid-Harbored rmtB in a Clinical Multidrug-Resistant Pseudomonas aeruginosa Strain. Microorganisms 2022;10:1818. [Crossref] [PubMed]
- Chen J, Zeng Y, Zhang R, et al. In vivo Emergence of Colistin and Tigecycline Resistance in Carbapenem-Resistant Hypervirulent Klebsiella pneumoniae During Antibiotics Treatment. Front Microbiol 2021;12:702956. [Crossref] [PubMed]
- Ghiglione B, Haim MS, Penzotti P, et al. Characterization of Emerging Pathogens Carrying bla(KPC-2) Gene in IncP-6 Plasmids Isolated From Urban Sewage in Argentina. Front Cell Infect Microbiol 2021;11:722536. [Crossref] [PubMed]
- Da R, Zhou Y, Cheng Y, et al. UhpTE350Q mutation along with the presence of fosA6/5 genes in the genome probably contributes to inherent fosfomycin resistance of Klebsiella pneumoniae. Nan Fang Yi Ke Da Xue Xue Bao 2023;43:1110-5. [Crossref] [PubMed]
- Chen S, Fu J, Zhao K, et al. Class 1 integron carrying qacEΔ1 gene confers resistance to disinfectant and antibiotics in Salmonella. Int J Food Microbiol 2023;404:110319. [Crossref] [PubMed]
- Yao H, Qin S, Chen S, et al. Emergence of carbapenem-resistant hypervirulent Klebsiella pneumoniae. Lancet Infect Dis 2018;18:25. [Crossref] [PubMed]
- Gu D, Dong N, Zheng Z, et al. A fatal outbreak of ST11 carbapenem-resistant hypervirulent Klebsiella pneumoniae in a Chinese hospital: a molecular epidemiological study. Lancet Infect Dis 2018;18:37-46. [Crossref] [PubMed]
- Zhou K, Xiao T, David S, et al. Novel Subclone of Carbapenem-Resistant Klebsiella pneumoniae Sequence Type 11 with Enhanced Virulence and Transmissibility, China. Emerg Infect Dis 2020;26:289-97. [Crossref] [PubMed]
- Zhang Y, Jin L, Ouyang P, et al. Evolution of hypervirulence in carbapenem-resistant Klebsiella pneumoniae in China: a multicentre, molecular epidemiological analysis. J Antimicrob Chemother 2020;75:327-36. [Crossref] [PubMed]
- Wyres KL, Wick RR, Judd LM, et al. Distinct evolutionary dynamics of horizontal gene transfer in drug resistant and virulent clones of Klebsiella pneumoniae. PLoS Genet 2019;15:e1008114. [Crossref] [PubMed]
- Sanikhani R, Moeinirad M, Shahcheraghi F, et al. Molecular epidemiology of hypervirulent Klebsiella pneumoniae: a systematic review and meta-analysis. Iran J Microbiol 2021;13:257-65. [Crossref] [PubMed]