Investigation of pure lung microbiota in patients with lung cancer after eliminating upper airway contamination: a prospective cohort study
Highlight box
Key findings
• Using a novel method, we successfully eliminated upper airway microbiota contamination and detected pure lung microbiota in patients with lung cancer.
What is known and what is new?
• The relationship between gut microbiome and gastrointestinal cancer has been extensively studied. However, the association between lung cancer and lung microbiome remains unexplored owing to technical limitations in harvesting pure lung microbiota. Previous lung microbiota studies mainly used bronchoalveolar lavage fluid (BALF) samples collected via bronchoscopy, which may have been contaminated by upper airway microbiota. In our prospective cohort study, BALF from the resected lung specimens collected under sterile conditions were used for high-throughput next-generation sequencing. Candidate Phylum OD1 bacteria (OD1) was detected in a significant proportion of patients. The predominant bacterial genera included Aquabacterium, Acinetobacter, Ralstonia, and bacteria commonly recognized as causative agents of postoperative pneumonia (POP).
What is the implication, and what should change now?
• Our collection method allows for comprehensive understanding of lung microbiota and can contribute to further research on the relationship between lung microbiota and lung cancer, as well as the potential use of targeted antibiotic prophylaxis to prevent POP by addressing pathogenic lung microbiota; additional research is required to elucidate the role of OD1 in lung cancer.
Introduction
Background
The relationship between the human microbiome and carcinogenesis has recently been elucidated. Several studies have analyzed the role of microbiota in cancer, particularly the relationship between gut microbiota and gastrointestinal cancer (1-4). Historically, studies on microbiota and lung cancer were limited because healthy lungs were considered sterile, owing to challenges in cultivating lung-specific microorganisms (5). Moreover, the bacterial load in a healthy lung is lower (103–105 bacteria/g of tissue) than that in the large intestine (up to 1011–1012 bacteria/g of tissue) (6,7). However, the widespread use of high-throughput sequencing techniques, such as next-generation sequencing (NGS), has revealed the existence of lung microbiota (8).
Rationale and knowledge gap
Several studies have highlighted the role of lung microbiota in inflammatory lung diseases, such as chronic obstructive pulmonary disease (COPD) and idiopathic pulmonary fibrosis (9-12); however, they used samples obtained from the oropharynx, such as saliva and sputum, and bronchoalveolar lavage fluid (BALF) collected during bronchoscopy, which may have been contaminated with upper airway microbiota. Charlson et al. (8) used BALF from a healthy human respiratory tract to compare lung and upper airway microbiota and demonstrated that healthy lung flora is indistinguishable from upper respiratory tract flora due to low bacterial levels. However, they were unable to determine whether their findings could have resulted from aspiration or bronchoscope contamination.
Analytical studies on lung microbiota in lung cancer are gradually emerging, but their precise role remains largely unknown. Certain microbiomes are associated with an increased risk of lung cancer (13). A previous study using BALF samples reported that lung microbiota differs between cancer-affected lobes and unaffected contralateral lung lobes in the same patients, with poorly differentiated cancers showing a trend toward reduced bacterial diversity (14). These findings suggest that the lung microbial environment can influence the intratumor microenvironment, with potential implications for future lung cancer treatments. Furthermore, regarding lung cancer recurrence, Patnaik et al. (15) revealed that the composition of lower airway microbiota may be associated with the recurrence of early-stage non-small-cell lung cancer (NSCLC), possibly due to an influence of the microbiome on tumor biology. They used presurgery BALF and saliva samples and resected lung tissue as specimens. Further, some studies have used surgically resected tumor tissues (16,17). Liu et al. (17) compared lung tissue-associated microbial signatures in smokers with lung cancer and in those with emphysema and reported a distinct difference in the lung microbial composition and milieu. Notably, only one study has used BALF from surgery specimens for analysis (18).
Postoperative pneumonia (POP) is a major complication of lung resections, especially in older patients with complicated clinical conditions, and is associated with high mortality (19,20). However, in recent years, the preoperative hospitalization period for lung resection has been reduced. Thus, we hypothesized that POP is caused by pre-existing bacteria and pathogens aspirated after hospitalization. Therefore, identifying the pure lung microbiota in patients with lung cancer may be beneficial in preventing and treating POP.
Objective
This study aimed to detect pure lung microbiota in patients with lung cancer using BALF samples collected from resected lung specimens under sterile conditions to avoid contamination from upper airway microbiota. Additionally, we aimed to evaluate the lung microbiota to clarify their relationship with lung cancer and aid in POP prevention and treatment. We present this article in accordance with the STROBE reporting checklist (available at https://jtd.amegroups.com/article/view/10.21037/jtd-24-933/rc).
Methods
Ethical statement
This prospective cohort study was performed in accordance with the Declaration of Helsinki (as revised in 2013). The study was approved by the Institutional Review Board of the Japanese Red Cross Suwa Hospital (No. 2-3), and written informed consent was obtained from all participants.
Participants, sample acquisition, and processing
This study enrolled patients with clinically suspected lung cancer who underwent lobectomy or segmentectomy at the Department of Thoracic Surgery, Japanese Red Cross Suwa Hospital, between April 2020 and March 2022. After resection, the staple at the bronchial stump of the resected lung was removed under sterile conditions. Subsequently, 100–300 mL of normal saline was injected into the bronchi using a 50-mL syringe. Once the resected lung was fully expanded, BALF was collected as much as possible by spontaneous drip, followed by gently squeezing the specimen with caution to avoid destruction of lung tissue (Figure 1). The BALF was then immediately transported to a clinical laboratory. All procedures for BALF collection were performed by a single investigator. A portion of the BALF was placed in a 2-mL Eppendorf tube and centrifuged (13,000 rpm, 30 min, 4 ℃), and the supernatant was discarded. The pellets were stored at −80 ℃ until DNA extraction. Bacterial cultures were cultivated using the remaining BALF samples. The study protocol is presented in Figure 2. The method of BALF sampling and preservation was based on a previous study that used BALF collected during bronchoscopy (21).
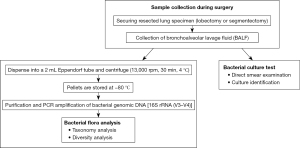
BALF DNA isolation and 16S sequencing
Genomic DNA was extracted from the BALF pellets using NucleoSpin™ Soil (Macherey-Nagel™, Düren, Germany) according to the manufacturer’s protocol. Double-stranded DNA (dsDNA) was quantified using the Quant-iT dsDNA HS Assay Kit (Invitrogen, Darmstadt, Germany). Then, purification was performed to improve the quality of genomic DNA using AMPure XP beads (Beckman Coulter Inc., Brea, CA, USA). The bacterial 16S rRNA V3–V4 region was amplified with forward and reverse primers of 341F primer (5'-TCGTCGGCAGCGTCAGATGTGTATAAGAGACAGCCTACGGGNGGCWGCAG-3') and 806R primer (5'-GTCTCGTGGGCTCGGAGATGTGTATAAGAGACAGGGACTACHVGGGTWTCTAAT-3'), respectively, with 16S (V3-V4) Metagenomic Library Construction Kit for NGS (Takara-bio, Shiga, Japan). The 16S rRNA amplification products were subjected to 28 cycles of polymerase chain reaction (PCR), with an annealing temperature of 50 ℃ and Tks Gflex DNA Polymerase. For PCR, the maximum volume of the sample’s DNA preparation was used. Amplified PCR products were purified using AMPure XP beads and were indexed with Nextera XT Index Kit v2 (Illumina Inc., San Diego, CA, USA) in 8-cycle PCR, with an annealing temperature of 60 ℃ to generate a sequencing library. The quantum fluorescence of the sequence library was quantified using the Quant-iT dsDNA HS Assay kit. Subsequently, cluster formation was performed using MiSeq Reagent Kit v3 and PhiX Control Kit v3. Sequence analysis was performed on the MiSeq platform (Illumina Inc, San Diego, CA, USA) using MiSeq Control Software v4.0.0.1769, Real-Time Analysis v1.18.54.4, and bclfastq2 v2.17.
Data analyses
To determine the bacterial composition of the sample, we performed taxonomic analysis using the QIIME2 pipeline (version 2021.2). After merging perfectly matched reads, eliminating noise and chimeric reads, and assembling the R1R2 reads, we constructed operational taxonomic units (OTUs) via clustering. The OTU construction based on FASTQ (after tag classification) for all samples obtained from the sequence was performed as follows. First, DADA2 was used for denoising, which included PCR primer sequence removal, overlapping of paired-end reads, burlaps, chimera checks, and merging sequences with a 100% match. Second, VSEARCH was employed for clustering with a 99% match rate threshold based on the sequences obtained through DADA2. The longest sequence in each cluster was selected as the representative sequence.
Phylogenetic nomenclature was used with the QIIME2 pipeline based on the OTU representative sequence. First, a native Bayes classifier model was created using the GreenGenes (version gg_13_8) 99% Clustering dataset. The classifier was then used to assign phylogenetic nomenclature to the OTU representative sequences. Abundance ratios were calculated from the results and visualized using a heat map and bar graph. Additionally, we performed a homology search with BLAST using DDBJ 16S ribosomal RNA (acquisition date: February 1, 2022) database to collect information on sequences related to each OTU.
A phylogenetic tree was created with representative OTU sequences using the QIIME2 pipeline. Initially, representative sequences were aligned using the MAFFT, and the storage area was masked. Subsequently, the evolutionary distance was calculated from the alignment results using FastTree, and phylogenetic tree data were created in the Newick format.
α-diversity analysis was performed using the QIIME2 pipeline. Initially, 50,000 reads (maximum lead amount if the number of leads was <50,000) were used, and leads were randomly extracted in 10 steps from one-tenth of the amount to the total amount. Based on the extracted leads, a Rarefaction Curve was created for the number of OTUs, Faith’s phylogenetic diversity, Chao1, and Shannon’s index.
Statistical analysis
Categorical variables were presented as number (percentage), while continuous variables were presented as the mean ± standard deviation (SD) or median (range). The correlation between bacterial abundance ratios and clinicopathological factors was assessed using Pearson’s product-moment correlation coefficient. The diversity analysis between patient characteristics was compared using t-tests. P<0.05 was considered a statistically significant value. All statistical analyses were performed using the IBM SPSS Statistics software (version 20.0.1.0).
Results
Table 1 provides an overview of the clinico-demographic characteristics of the 54 participants in our study. Among them, 27 (50.0%) were men, with age ranging from 46 to 86 years (mean age: 72.8 years). Smoking history was reported in 24 participants (44.5%), with 15 (27.8%) being heavy smokers, as defined by a Brinkman index (BI) of ≥600. Regarding comorbid lung conditions, eight participants (14.8%) had COPD and three (5.6%) had asthma. Although one participant had a history of bacterial pneumonia over a decade ago, there were no signs of pneumonia at the time of surgery. Histologically, 44 participants (81.6%) presented with adenocarcinomas, including atypical adenomatous hyperplasia and adenocarcinoma in situ, while seven (13.0%) had squamous cell carcinoma. Hospital stays were relatively short, with median pre- and postoperative durations of 1 [1–7] and 5.5 [3–22] days, respectively.
Table 1
Variables | All cases (N=54) |
---|---|
Age, years | 72.8±9.4 |
Sex, male | 27 (50.0) |
Smoking history | |
Current | 1 (1.9) |
Former | 23 (42.6) |
Never | 30 (55.5) |
Brinkman index | |
<600 | 39 (72.2) |
≥600 | 15 (27.8) |
Comorbid lung disease | |
COPD | 8 (14.8) |
Asthma | 3 (5.6) |
MAC | 1 (1.9) |
History of bacterial pneumonia | 1 (1.9) |
Interstitial pneumonia | 0 |
Surgery | |
Lobectomy | 37 (68.5) |
Segmentectomy | 17 (31.5) |
Histology | |
AAH | 1 (1.9) |
AIS | 12 (22.2) |
MIA | 3 (5.6) |
Invasive adenocarcinoma | 28 (51.9) |
Lepidic | 9 (16.7) |
Acinar | 6 (11.1) |
Papillary | 9 (16.7) |
Micropapillary | 1 (1.9) |
Solid | 1 (1.9) |
Mucinous | 2 (3.7) |
Squamous cell carcinoma | 7 (13.0) |
Squamous cell dysplasia | 1 (1.9) |
Metastatic tumor | 2 (3.7) |
Bacterial culture positive | 13 (24.1) |
Length of hospital stay, days | |
Preoperative | 1 [1–7] |
Postoperative | 5.5 [3–22] |
Data are presented as mean ± standard deviation, n (%), or median [range]. COPD, chronic obstructive pulmonary disease; MAC, mycobacterium avium complex; AAH, atypical adenomatous hyperplasia; AIS, adenocarcinoma in situ; MIA, minimally invasive adenocarcinoma.
The results of bacterial cultures are outlined in Table 2. Bacterial growth was observed in 13 (24.1%) samples, with Staphylococcus spp. being the most frequently detected in four cases, while Bacillus spp., Streptomyces spp., Aspergillus spp., and Penicillium spp. were detected in three cases. Notably, six of the 13 patients showed similar bacterial genera when analyzed via NGS: Staphylococcus spp. in three cases, Propionibacterium spp. in two cases, and Streptomyces spp. in one case.
Table 2
Case | Detected bacteria |
---|---|
1 | Streptomyces hachijoensis |
2 | Penicillium spp. |
3† | Staphylococcus capitis, Propionibacterium spp.†, Aspergillus niger, Penicillium spp., Streptomyces spp. |
4† | Propionibacterium acnes |
5 | Aspergillus niger |
6 | Bacillus spp. |
7 | Bacillus spp. |
8† | Baccillus subtilis, Paenibacillus spp., Streptomyces spp.†, Aspergillus niger |
9† | Staphylococcus capitis |
10 | Penicillium spp. |
11† | Staphylococcus hominis |
12† | Staphylococcus saccharolyticus |
13 | Paenibacillus spp. |
Bacteria were detected in 13 samples (24.1%) via a general bacterial culture. †, six cases had the same type of bacteria detected in high-throughput next-generation sequencing analysis.
Adhering to quality control procedures, such as noise elimination, 8,906 valid tags were obtained using the Illumina MiSeq™ platform. A total of 6,504 OTUs were identified, with a mean sequence length of 326.48 (±64.15) and average reads per sample of 147,645 (±30,822). After excluding host reads, the average number of bacterial reads per sample was 56,645 (±14,788).
A bar graph illustrating the phylogenetic classification and abundance ratio based on the read amount of each OTU was created (Figure 3). This graph indicated that Candidate Phylum OD1 bacteria (OD1) and Proteobacteria spp. were prevalent in a large proportion of the samples. A correlation analysis between the relative abundance ratio (each bacterial read amount/total bacterial read amount) of OD1 and pathological tumor size was performed to evaluate the relationship between OD1 and lung cancer (Figure S1A-S1D). The results demonstrated a significant positive correlation between OD1 ratio and invasive tumor size in patients with primary lung cancer (P=0.044, r=0.283). However, no such correlation was observed with the total tumor size (P=0.70, r=0.055). Notably, there was no significant correlation among patients with adenocarcinoma alone. Figure 4 displays a pie chart (genus level) representing the major bacteria with a relative abundance ratio >1% in at least one sample. These include Aquabacterium, Acinetobacter, Ralstonia, Sphingomonas, Burkholderia, Pseudomonas, Novosphingobium, Methylobacterium, Staphylococcus, Pelomonas, Bifidobacterium, Cupriavidus, and Kocuria. No uniformity or characteristic differences were observed in the relative abundance ratios of each bacterium across samples.
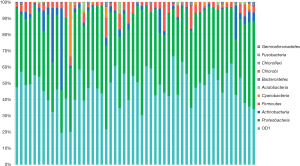
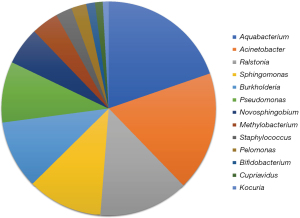
The causative bacteria of POP are generally Haemophilus spp., Streptococcus pneumoniae, Staphylococcus spp., Pseudomonas spp., and Enterobacter spp. (22). The causative bacteria of nursing and healthcare-associated pneumonia (NHCAP) are S. pneumoniae, Escherichia coli, H. influenzae, Klebsiella spp., Pseudomonas spp., Staphylococcus spp., and Proteus spp. (23). The results of NGS analysis for bacteria that may be common pathogens for POP and NHCAP are listed in Table 3. The most prevalent bacteria (genus level) were Acinetobacter spp. in 51 cases (94.4%), Pseudomonas spp. in 35 cases, Corynebacterium spp. in 18 cases, Bacillus spp. in 10 cases, and Staphylococcus spp. in seven cases.
Table 3
Bacteria (genera) | All cases (N=54) | Smoking history (N=24) | No-smoking history (N=30) |
---|---|---|---|
Acinetobacter | 51 (94.4) | 22 (91.7) | 29 (96.7) |
Pseudomonas | 35 (64.8) | 15 (62.5) | 20 (66.7) |
Corynebacterium | 18 (33.3) | 7 (29.2) | 11 (36.7) |
Bacillus | 10 (18.5) | 3 (12.5) | 7 (23.3) |
Staphylococcus | 7 (13.0) | 3 (12.5) | 4 (13.3) |
Propionibacterium | 5 (9.3) | 5 (20.8) | 0 |
Streptococcus | 4 (7.4) | 3 (12.5) | 1 (3.3) |
Mycobacterium | 4 (7.4) | 2 (8.3) | 2 (6.7) |
Streptomyces | 3 (5.6) | 1 (4.2) | 2 (6.7) |
Prevotella | 2 (3.7) | 2 (8.3) | 0 |
Bacteroides | 2 (3.7) | 2 (8.3) | 0 |
Fusobacterium | 1 (1.9) | 1 (4.2) | 0 |
Citrobacter | 1 (1.9) | 0 | 1 (3.3) |
All data are presented as N (%). Bacteria that are common pathogens for POP and NHCAP in the high-throughput next-generation sequencing analysis. NGS, next generation sequencing; POP, postoperative pneumonia; NHCAP, nursing and healthcare-associated pneumonia.
Diversity analysis, performed based on phylogenetic analysis, revealed that the mean Shannon’s index was 6.43 (±0.27). Comparison of α-diversity for smoking history, BI, and COPD is shown in Figure S2A-S2C. The result of comparison of Shannon’s diversity index between current or former smokers and never smokers was P=0.25, 95% CI: −0.64 to 0.24, BI <600 and ≥600 was P=0.53, 95% CI: −0.23 to 0.12, and COPD (+) and COPD (−) was P=0.15, 95% CI: −3.67 to 0.06, respectively.
Discussion
Almost all previous studies on lung BALF microbiota used samples obtained via bronchoscopy, which had a risk of microbial contamination from the upper airway. Therefore, whether previous reports detected pure lung microbiota was unclear. Some previous studies showed that bacterial samples collected using bronchoscopic BALF were not affected by contamination from upper airway tract (24), and this procedure is the most used sampling method to evaluate lower airway tract. Meanwhile, in chronic lung diseases such as COPD, some reports suggest that bacterial flora tend to differ between the upper and lower airway tracts depending on disease duration (25). Therefore, it is crucial to separate the upper and lower airway microbiomes to accurately evaluate the influence of lung microbiome in such diseases. Establishing a sampling method for detecting pure microbiota is essential because the techniques and sources for acquiring samples play an important role in ensuring accurate evaluation and advancing research on the lung microbiome (26).
To the best of our knowledge, only one study to date has assessed the pure lung microbiome using BALF collected from surgical specimens under sterile conditions (18), with no reports of obtaining samples from the entire excised lung. In this regard, our experimental procedure is novel. In this study, we aimed to detect pure lung microbiota in patients with lung cancer. We found that bacterial flora certainly exists in the lung, and bacterial species were also identified. Additionally, we aimed to evaluate the lung microbiota to clarify its relationship with lung cancer and POP.
First, we verified the reliability of our proposed method. Analysis of the lung microbiota using the Illumina MiSeq platform yielded a total of 6,504 OTU and 56,645 (±14,788) bacterial reads/sample. These results were better compared with those of previous reports using bronchoscopy-obtained BALF; for example, 190 unique OTUs and 11,964 (±18,337) bacterial reads/sample (27), indicating the reliability of our experimental method. Moreover, we found only one report on lung microbiota using BALF from surgical specimens (18); however, unlike our method, which involved injection of 100–300 mL normal saline until complete expansion of the resected lung, BALF was obtained after instillation and aspiration of 20 mL of normal saline. Although the reads and OTU counts were not mentioned in the aforementioned study, we believe that our sampling method enabled more consistent detection of the lung microbiota throughout the entire resected lung.
Regarding bacterial species, the results of the phylum-level analysis indicated that OD1 and Proteobacteria spp. were present in large proportions in every sample. OD1 or “Parcubacteria” have been identified in various anoxic environments (28,29), and their genomes generally lack genes for the biosynthesis of amino acids, nucleotides, vitamins, and lipids (30); further, their ecosystems are largely unknown. Although we were unable to find reports on lung microbiota-related OD1, several studies have suggested a relationship between OD1 and cancer. Thomas et al. (31) evaluated differences in microbiota found in tissue samples from 18 rectal cancer patients and 18 non-cancer control participants and concluded that the rectal cancer samples had an increased abundance of OD1. Hawkins et al. (32) evaluated the microbiota of 95 patients with early-stage endometrial cancer according to race and revealed that microbial diversity was increased, and OD1 abundance was higher in Black women than in women of other racial groups. Although these studies did not clarify the significance of OD1 in each cancer type, they suggest a possible association between OD1 and cancer. In our study, we detected OD1, which is hitherto unreported in lung cancer. Furthermore, the results of correlation between OD1 relative abundance ratio with pathological tumor size indicated a significant positive correlation between invasive tumor size with OD1 ratio in patients with primary lung cancer. While no significant correlation was noted in patients with adenocarcinoma alone, a correlation was observed between invasive size and OD1 ratio. Although the correlation was weak, these results suggest that OD1 may influence high tumor malignancy, although we were unable to compare with non-tumor samples. However, the observed correlation appears weak, possibly due to the limited number of cases in our analysis, indicating the need for future research on the significance of OD1.
Culture results revealed that bacteria were detected in only 13 samples (24%). Additionally, although the remaining 41 samples were clinically diagnosed as sterile, bacterial flora analysis using NGS revealed the presence of microbiota in all samples, indicating that the microbiota are present in clinically healthy individuals. Regarding comparison of bacterial cultures and NGS, at least one type of bacteria was detected in six of 13 samples. In seven samples, the results were inconsistent between bacterial detection methods (bacteria were detected only in the culture test); the detected genera in these samples were Streptomyces, Aspergillus, Bacillus, and Paenibacillus. However, since these bacteria are known to reside in the environment, we assumed that these were not detected by NGS owing to possible contamination during the culture phase.
Our bacterial flora analysis revealed a high proportion of genera such as Pseudomonas, Acinetobacter, and Ralstonia. Several studies have identified other lung microbiota genera, including Prevotella, Streptococcus, Veinonella, Fusobacteriumu, and Haemophilus (9,33-35). However, oral microbiota genera, such as Desulfobulbus, Abiotrophia, and Selenomanas (11), were not detected in large proportions in our study, suggesting the efficiency of our method in analyzing pure lung microbiota. To compare the lung microbiota with those from other body sites, we used data from the National Institutes of Health Human Microbiome Project (NIH-HMP) (Figure 5A-5C) (36). Our lung microbiota differed from those of any other body site, especially the gut (stool); however, the most similar composition was observed in the mouth. This result is consistent with a previous report (17) and supports the hypothesis that the lung microbiota results from aspiration of the upper airway microbiota. Patnaik et al. (15) reported significant levels of α-amylase enzyme activity in BALF samples of some patients and found a closer correlation between the salivary and BALF microbial composition in patients with higher amylase activity level in BALF. Given that α-amylase is generally not secreted in the respiratory tract, their results also support the hypothesis that the airway microbiome may originate from seeding by the oral microbiome. Some studies found that Acinetobacter, detected in our study as well, was present in significantly lower abundance in patients with lung cancer than in healthy individuals (17,37). However, we were unable to confirm this because we did not enroll healthy control participants; our study only included patients who underwent surgery to avoid additional invasive procedures. Moreover, even though previous studies have demonstrated that various bacteria are present in higher concentrations in patients with lung cancer rather than in controls (16), a consensus has yet to be reached due to large differences between reports. Generally, COPD is a common comorbidity in lung cancer and an independent risk factor for lung cancer (38,39). Furthermore, COPD is known to cause alterations in the lung microbiota, similar to other inflammatory lung diseases (40,41). Cookson et al. (40) observed an overgrowth of Proteobacteria, such as H. influenzae and Neisseria spp., and a loss of normal flora in COPD patients. We compared the constituent bacteria between patients with and without COPD and found that Proteobacteria accounted for a large proportion in patients with COPD. This finding was consistent with the results of previous reports (40), although the number of COPD patients in our research remains small (n=8), and disease severity was not controlled. We believe that further studies with a large cohort are needed to substantiate our results.
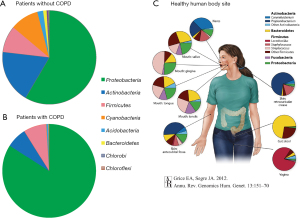
In Japan, the major pathogens causing NHCAP were reportedly S. pneumonia, E. coli, Staphylococcus aureus, and P. aeruginosa (23). Hospital-acquired pneumonia is reportedly caused by the aspiration of these bacteria. However, in cases of lung resection, particularly in patients with lung cancer, preoperative hospital stays are becoming shorter, as observed in our results (median, 1 day). Therefore, we assume that the pathogens causing POP are aspirated post-hospitalization and bacteria are already present pre-admission. Schussler et al.’s study on POP (22) involved 168 patients who underwent lung resection. They collected cultures of intraoperative bronchial aspirates for comparison with POP samples and found that POP developed more frequently in patients with colonization (preoperative sample-positive) than in patients without colonization (44.4% vs. 21.0%, P=0.04) and that POP occurred earlier in patients with colonization. Furthermore, Georges et al. (42) suggested that a positive bronchial aspirate, which reflected preoperative respiratory tract colonization, was an independent risk factor for the occurrence of POP. Those reports (22,42) support our hypothesis that lung microbiota may cause POP in postoperative immunocompromised conditions. Our results revealed the presence of Acinetobacter spp. and Pseudomonas spp. in many cases, which are widely known pathogens of opportunistic infectious diseases and NHCAP. These bacteria are generally assumed to be aspirated post-hospitalization and cause infections in immunocompromised conditions; however, there is a possibility that they already exist as part of the lung microbiota before hospitalization. Therefore, antibiotic prophylaxis targeting these bacteria may be useful for the prevention and initial treatment of POP. Nevertheless, we were unable to assess the causative bacteria because no patient developed POP. Therefore, further research is required on the influence of the lung microbiota, which have not been previously described as causative bacteria.
In the α diversity analysis, Shannon’s diversity index exhibited no significant difference between current or former smokers and never smokers, BI <600 and ≥600, and COPD (+) and COPD (−). Notably, α diversity has been reported to decrease in inflammatory lung disease due to increased levels of certain bacteria (43-45). However, no significant differences were observed in the present study, which had a relatively small sample size.
Despite elucidating a novel microbe detection method, this study had several limitations. First, it was a single-investigator study conducted at a single institute, and, although the sample size was relatively larger compared to previous studies involving surgical tissues (17,46), it remains relatively small. Therefore, a comparison of the microbiota by background is insufficient. Nevertheless, this may be advantageous because it could eliminate differences in experimental techniques between samples. Second, we did not investigate the oral and upper airway microbiota. Although we were able to detect pure lung microbiota, we could not accurately assess whether these results were caused by aspiration/contamination or the original microbiota in the lung. Hence, we compared our results with those of previous studies. Third, the study lacked non-tumor control participants, which prevented a thorough evaluation of the relationship between lung cancer and microbiota. Finally, the data for diversity analysis, particularly for β-diversity analysis, were insufficient. For diversity analysis, we focused only on α-diversity analysis using the Shannon index, but no significant results were obtained in α-diversity analysis between patients with COPD and those without COPD, possibly due to the small sample size. Furthermore, we could not conduct a comparative study of other diversity analyses because of the lack of comparator samples, such as oral and upper airway samples and non-tumor samples, as mentioned above. These aspects should be explored in future studies.
Conclusions
This prospective study showed that our lung microbiota sampling method successfully removed upper airway microbiota contamination and identified the presence of pure lung microbiota. These findings provide valuable baseline data for pure lung microbiota. Future research should elucidate the role of OD1 in lung cancer, which has not been previously reported in lung microbiota studies. While the pathogens responsible for POP are typically assumed to be aspirated after hospitalization, our results indicate that they might already exist as part of the lung microbiota before hospitalization. However, further investigation is needed to substantiate our results and hypothesis.
Acknowledgments
We acknowledge the cooperation of the clinical laboratory of the Japanese Red Cross Suwa Hospital during our research. We would like to thank Takara-bio (www.takara-bio.co.jp) for NGS data analysis and Editage (www.editage.jp) for English language editing.
Funding: This work was supported by
Footnote
Reporting Checklist: The authors have completed the STROBE reporting checklist. Available at https://jtd.amegroups.com/article/view/10.21037/jtd-24-933/rc
Data Sharing Statement: Available at https://jtd.amegroups.com/article/view/10.21037/jtd-24-933/dss
Peer Review File: Available at https://jtd.amegroups.com/article/view/10.21037/jtd-24-933/prf
Conflicts of Interest: All authors have completed the ICMJE uniform disclosure form (available at https://jtd.amegroups.com/article/view/10.21037/jtd-24-933/coif). The authors have no conflicts of interest to declare.
Ethical Statement: The authors are accountable for all aspects of the work in ensuring that questions related to the accuracy or integrity of any part of the work are appropriately investigated and resolved. This prospective cohort study was conducted in accordance with the Declaration of Helsinki (as revised in 2013). The study was approved by the Institutional Review Board of the Japanese Red Cross Suwa Hospital (No. 2-3), and informed consent was obtained from all individual participants.
Open Access Statement: This is an Open Access article distributed in accordance with the Creative Commons Attribution-NonCommercial-NoDerivs 4.0 International License (CC BY-NC-ND 4.0), which permits the non-commercial replication and distribution of the article with the strict proviso that no changes or edits are made and the original work is properly cited (including links to both the formal publication through the relevant DOI and the license). See: https://creativecommons.org/licenses/by-nc-nd/4.0/.
References
- García-Castillo V, Sanhueza E, McNerney E, et al. Microbiota dysbiosis: a new piece in the understanding of the carcinogenesis puzzle. J Med Microbiol 2016;65:1347-62. [Crossref] [PubMed]
- Chen J, Domingue JC, Sears CL. Microbiota dysbiosis in select human cancers: Evidence of association and causality. Semin Immunol 2017;32:25-34. [Crossref] [PubMed]
- Helmink BA, Khan MAW, Hermann A, et al. The microbiome, cancer, and cancer therapy. Nat Med 2019;25:377-88. [Crossref] [PubMed]
- Rubinstein MR, Wang X, Liu W, et al. Fusobacterium nucleatum promotes colorectal carcinogenesis by modulating E-cadherin/β-catenin signaling via its FadA adhesin. Cell Host Microbe 2013;14:195-206. [Crossref] [PubMed]
- Zhao L. Genomics: The tale of our other genome. Nature 2010;465:879-80. [Crossref] [PubMed]
- Mathieu E, Escribano-Vazquez U, Descamps D, et al. Paradigms of Lung Microbiota Functions in Health and Disease, Particularly, in Asthma. Front Physiol 2018;9:1168. [Crossref] [PubMed]
- Guarner F, Malagelada JR. Gut flora in health and disease. Lancet 2003;361:512-9. [Crossref] [PubMed]
- Charlson ES, Bittinger K, Haas AR, et al. Topographical continuity of bacterial populations in the healthy human respiratory tract. Am J Respir Crit Care Med 2011;184:957-63. [Crossref] [PubMed]
- Wypych TP, Wickramasinghe LC, Marsland BJ. The influence of the microbiome on respiratory health. Nat Immunol 2019;20:1279-90. [Crossref] [PubMed]
- Yagi K, Huffnagle GB, Lukacs NW, et al. The Lung Microbiome during Health and Disease. Int J Mol Sci 2021;22:10872. [Crossref] [PubMed]
- Pragman AA, Lyu T, Baller JA, et al. The lung tissue microbiota of mild and moderate chronic obstructive pulmonary disease. Microbiome 2018;6:7. [Crossref] [PubMed]
- Opron K, Begley LA, Erb-Downward JR, et al. Lung microbiota associations with clinical features of COPD in the SPIROMICS cohort. NPJ Biofilms Microbiomes 2021;7:14. [Crossref] [PubMed]
- Kovaleva OV, Romashin D, Zborovskaya IB, et al. Human Lung Microbiome on the Way to Cancer. J Immunol Res 2019;2019:1394191. [Crossref] [PubMed]
- Reddy RM, Lagisetty K, Lin J, et al. Comprehensive sampling of the lung microbiome in early-stage non-small cell lung cancer. JTCVS Open 2024;17:260-8. [Crossref] [PubMed]
- Patnaik SK, Cortes EG, Kannisto ED, et al. Lower airway bacterial microbiome may influence recurrence after resection of early-stage non-small cell lung cancer. J Thorac Cardiovasc Surg 2021;161:419-429.e16. [Crossref] [PubMed]
- Goto T. Microbiota and lung cancer. Semin Cancer Biol 2022;86:1-10. [Crossref] [PubMed]
- Liu Y, O'Brien JL, Ajami NJ, et al. Lung tissue microbial profile in lung cancer is distinct from emphysema. Am J Cancer Res 2018;8:1775-87.
- Zheng L, Sun R, Zhu Y, et al. Lung microbiome alterations in NSCLC patients. Sci Rep 2021;11:11736. [Crossref] [PubMed]
- Kaprin A, Pikin O, Ryabov A, et al. Surgical intervention for lung cancer in patients aged 75 and above: potential associations with increased mortality rates-a single-center observational study. J Cardiothorac Surg 2024;19:471. [Crossref] [PubMed]
- Arozullah AM, Henderson WG, Khuri SF, et al. Postoperative mortality and pulmonary complication rankings: how well do they correlate at the hospital level? Med Care 2003;41:979-91. [Crossref] [PubMed]
- Dickson RP, Erb-Downward JR, Prescott HC, et al. Analysis of culture-dependent versus culture-independent techniques for identification of bacteria in clinically obtained bronchoalveolar lavage fluid. J Clin Microbiol 2014;52:3605-13. [Crossref] [PubMed]
- Schussler O, Alifano M, Dermine H, et al. Postoperative pneumonia after major lung resection. Am J Respir Crit Care Med 2006;173:1161-9. [Crossref] [PubMed]
- Imamura Y, Miyazaki T, Watanabe A, et al. Prospective multicenter survey for Nursing and Healthcare-associated Pneumonia in Japan. J Infect Chemother 2022;28:1125-30. [Crossref] [PubMed]
- Bassis CM, Erb-Downward JR, Dickson RP, et al. Analysis of the upper respiratory tract microbiotas as the source of the lung and gastric microbiotas in healthy individuals. mBio 2015;6:e00037. [Crossref] [PubMed]
- Watson RL, de Koff EM, Bogaert D. Characterising the respiratory microbiome. Eur Respir J 2019;53:1801711. [Crossref] [PubMed]
- Yu S, Zhang H, Wan L, et al. The association between the respiratory tract microbiome and clinical outcomes in patients with COPD. Microbiol Res 2023;266:127244. [Crossref] [PubMed]
- Dickson RP, Erb-Downward JR, Freeman CM, et al. Spatial Variation in the Healthy Human Lung Microbiome and the Adapted Island Model of Lung Biogeography. Ann Am Thorac Soc 2015;12:821-30. [Crossref] [PubMed]
- Harris JK, Kelley ST, Pace NR. New perspective on uncultured bacterial phylogenetic division OP11. Appl Environ Microbiol 2004;70:845-9. [Crossref] [PubMed]
- Nelson WC, Stegen JC. The reduced genomes of Parcubacteria (OD1) contain signatures of a symbiotic lifestyle. Front Microbiol 2015;6:713. [Crossref] [PubMed]
- Kantor RS, Wrighton KC, Handley KM, et al. Small genomes and sparse metabolisms of sediment-associated bacteria from four candidate phyla. mBio 2013;4:e00708-13. [Crossref] [PubMed]
- Thomas AM, Jesus EC, Lopes A, et al. Tissue-Associated Bacterial Alterations in Rectal Carcinoma Patients Revealed by 16S rRNA Community Profiling. Front Cell Infect Microbiol 2016;6:179. [Crossref] [PubMed]
- Hawkins GM, Burkett WC, McCoy AN, et al. Differences in the microbial profiles of early stage endometrial cancers between Black and White women. Gynecol Oncol 2022;165:248-56. [Crossref] [PubMed]
- Dickson RP, Erb-Downward JR, Martinez FJ, et al. The Microbiome and the Respiratory Tract. Annu Rev Physiol 2016;78:481-504. [Crossref] [PubMed]
- Huffnagle GB, Dickson RP, Lukacs NW. The respiratory tract microbiome and lung inflammation: a two-way street. Mucosal Immunol 2017;10:299-306. [Crossref] [PubMed]
- Lee SH, Lee Y, Park JS, et al. Characterization of Microbiota in Bronchiectasis Patients with Different Disease Severities. J Clin Med 2018;7:429. [Crossref] [PubMed]
- Grice EA, Segre JA. The human microbiome: our second genome. Annu Rev Genomics Hum Genet 2012;13:151-70. [Crossref] [PubMed]
- Yang J, Mu X, Wang Y, et al. Dysbiosis of the Salivary Microbiome Is Associated With Non-smoking Female Lung Cancer and Correlated With Immunocytochemistry Markers. Front Oncol 2018;8:520. [Crossref] [PubMed]
- Wang W, Xie M, Dou S, et al. The link between chronic obstructive pulmonary disease phenotypes and histological subtypes of lung cancer: a case-control study. Int J Chron Obstruct Pulmon Dis 2018;13:1167-75. [Crossref] [PubMed]
- Forder A, Zhuang R, Souza VGP, et al. Mechanisms Contributing to the Comorbidity of COPD and Lung Cancer. Int J Mol Sci 2023;24:2859. [Crossref] [PubMed]
- Cookson WOCM, Cox MJ, Moffatt MF. New opportunities for managing acute and chronic lung infections. Nat Rev Microbiol 2018;16:111-20. [Crossref] [PubMed]
- O'Shaughnessy M, Sheils O, Baird AM. The Lung Microbiome in COPD and Lung Cancer: Exploring the Potential of Metal-Based Drugs. Int J Mol Sci 2023;24:12296. [Crossref] [PubMed]
- Georges O, Abou Arab O, Ben Rahal M, et al. Diagnostic value of systematic bronchial aspirate on postoperative pneumonia after pulmonary resection surgery for lung cancer: a monocentre retrospective study. Interdiscip Cardiovasc Thorac Surg 2024;38:ivad212. [Crossref] [PubMed]
- Li N, Dai Z, Wang Z, et al. Gut microbiota dysbiosis contributes to the development of chronic obstructive pulmonary disease. Respir Res 2021;22:274. [Crossref] [PubMed]
- Cuthbertson L, Walker AW, Oliver AE, et al. Lung function and microbiota diversity in cystic fibrosis. Microbiome 2020;8:45. [Crossref] [PubMed]
- Pragman AA, Hodgson SW, Wu T, et al. Sputum microbiome α-diversity is a key feature of the COPD frequent exacerbator phenotype. ERJ Open Res 2024;10:00595-2023. [Crossref] [PubMed]
- Apopa PL, Alley L, Penney RB, et al. PARP1 Is Up-Regulated in Non-small Cell Lung Cancer Tissues in the Presence of the Cyanobacterial Toxin Microcystin. Front Microbiol 2018;9:1757. [Crossref] [PubMed]