Farnesoid X receptor (FXR) as a potential therapeutic target for lung diseases: a narrative review
Introduction
Farnesoid X receptor (FXR), which is encoded by the NR1H4 gene, is mainly expressed in the liver and gastrointestinal tract, is a member of the nuclear receptor (NR) superfamily, and is a major bile acid (BA) ligand-activated transcription factor (1). As a BA-sensing receptor, it is well known that FXR mainly participates in regulating BA metabolism through two pathways. First, a high concentration of BA activates the FXR/small heterodimer chaperone (SHP) pathway, thereby inhibiting the expression of cholesterol-7a-hydroxylase (CYP7A1) and sterol 12a-hydroxylase (CYP8B1) in the liver and reducing BA synthesis (2,3). Second, FXR activation increases the secretion of fibroblast growth factor-15/19 (mouse FGF15 and human FGF19) in the intestine, and binds to fibroblast growth factor receptor 4 (FGFR4) and its co-receptor β-klotho in hepatocytes, activates the c-Jun N-terminal kinase (JNK) and extracellular regulated protein kinases 1/2 (ERK1/2) pathways, inhibits CYP7A1 and CYP8B1 expression, and reduces BA synthesis (4,5). In brief, FXR regulates the enterohepatic circulation of BA by controlling the synthesis and secretion of BA, which is an efficient physiological strategy to maintain BA homeostasis.
In addition, recent evidence suggests that FXR also participates in the regulation of metabolic-related diseases, such as atherosclerosis, type 2 diabetes, hyperlipidemia, and abnormal weight gain (6,7). Notably, FXR is tissue-specific in regulating lipid metabolism. Specifically, the knockout of FXR in the intestine (8-10) and adipocytes (11) has been shown to reduce obesity and its side effects, while the knockout of FXR in the whole body (12,13) or liver (14) has bene shown to lead to excessive lipid accumulation and promote obesity. There is also increasing evidence that FXR not only plays a role in metabolic diseases, but also in the inflammatory response and immune system. For example, the activation of pancreatic acinar FXR restores the expression of oxidative stress-induced growth inhibitor 1, thereby improving pancreatitis (15). It is widely accepted that FXR exerts anti-inflammatory effects by directly inhibiting inflammatory signaling pathways and indirectly regulating BA, glucose, and lipid metabolism. However, it was recently discovered that FXR binds to the promoter of the oxidase glutathione S-transferase α-4 (Gsta4) gene to reduce the expression of Gsta4 and thus also plays a pro-inflammatory role in high-fat diet (HFD)-induced inflammation (11).
The mechanism by which FXR regulates different lung diseases may not be consistent. Indeed, the mechanism of action may even differ in the same lung disease. Thus, research needs to be conducted to examine the exact role of FXR in lung diseases, and its use as a new target for the treatment of lung diseases. In this article, we comprehensively summarize the pathogenic mechanisms of FXR in lung diseases to provide potential targets for the clinical treatment of lung diseases. We present this article in accordance with the Narrative Review reporting checklist (available at https://jtd.amegroups.com/article/view/10.21037/jtd-24-734/rc).
Methods
Online databases were searched to retrieve relevant articles. Research on the role of FXR in regulating lung diseases was then summarized. The search strategy is detailed in Table 1.
Table 1
Items | Specification |
---|---|
Date for search | Jan 2023 to Sep 2024 |
Databases and other sources searched | PubMed and National Center for Biotechnology Information |
Search terms used | “FXR”, “lung disease”, “ALI”, “bile acid”, “acute lung injury”, “lung ischemia-reperfusion”, “lung fibrosis”, “lung cancer” |
Timeframe | Apr 2000 to Aug 2024 |
Inclusion criteria | Study type: original article, review, clinical trials; language restrictions: English only |
Selection process | All authors independently searched and shared the articles, disagreements were resolved by discussion |
FXR, farnesoid X receptor; ALI, acute lung injury.
Pathophysiological functions of FXR
The basics
The NR superfamily is a transcription factor family that is widely expressed throughout the body and consists of more than 500 members (16). As a member of the NR family. FXR has a typical NR structure: a ligand-independent transcriptional activation function domain (AF1), a conserved DNA binding domain (DBD), a ligand binding domain (LBD), a ligand-dependent activation function domain (AF2), and a hinge region connecting the LBD and DBD (Figure 1A) (17,18). FXR has two known genotypes: FXRα (NR1H4) and FXRβ (NR1H5) (19). Four distinct human and rodent isoforms of FXRα1-α4 are encoded by a single FXRα gene using different promoters and RNA alternative splicing (20,21). Among them, FXRa3 and FXRa4 have an extended N-terminal. FXRα1 and FXRα3 insert a four amino acid (MYTG) in the hinge region adjacent to the DBD (Figure 1B) (22). FXRβ is a functional NR in mice, rats, rabbits, and dogs, but is a pseudogene in humans and primates, and its functional role is still unclear (6,23). In this article, we mainly summarize the role of FXRα in lung diseases; in this article, the term FXR refers to FXRα. FXR is mostly expressed in digestive tissues, such as the liver (24) and intestine (25). However, it is also expressed in the non-digestive tissues of “non-classical” BA target organs, such as the kidney (26), adrenal gland (27), vascular wall (28), adipose tissue (11), pancreas (15), and lung (29).
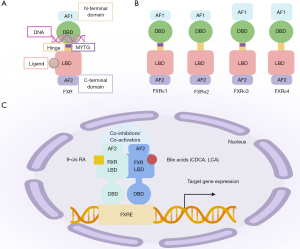
Notably, FXR is a ligand-activated transcription factor. FXR can be activated by hydrophobic molecules, such as steroids, hormones, and fatty acids. FXR binds to ligands and the heterodimeric complex formed by the retinoid X receptor and FXR response element (FXRE), triggering receptor conformational changes and regulating the recruitment of co-inhibitors and co-activators, affecting targeted gene transcription (Figure 1C) (30,31). It is well known that FXR is widely involved in regulating BA, the inflammatory response, and the barrier function, and lipid and glucose homeostasis (32,33).
BA homeostasis
BAs are considered endogenous FXR ligands with a high affinity for FXR. FXR is essential in regulating BA circulation, and maintaining BA homeostasis in the intestine and liver (Figure 2). It has been suggested that two FXR-dependent pathways mediate BA feedback to regulate BA homeostasis (34). One of these pathways occurs in the liver; elevated BA-activated FXR mediates the transcriptional inhibition of CYP7A1-encoding genes and limits the synthesis of BA (35,36). It is well known that CYP7A1 is a rate-limiting enzyme for BA synthesis. In addition, the FXR/SHP pathway also inhibits the transcriptional regulation of BA synthesis via the CYP8B1 gene (13,37). Notably, when BA is elevated in the liver, FXR induces the expression of bile salt expert pump (BSEP) and multi-drug resistance protein 2 (MRP2), which mediates the efflux of BAs into the bile duct (38). At the same time, activated FXR inhibits the hepatocyte uptake of BA by down-regulating sodium/taurocholate cotransporter polypeptide (NTCP) and organic anion transport peptide (OATP), and promotes BA secretion from the liver to the blood circulation by stimulating organic solute transporter α and β heterodimer (OSTα/β) and multi-drug resistance protein 4 (MRP4) BA transporters (38). FXR mediates BA feedback to inhibit BA synthesis, maintains low BA concentration in the liver, and prevents cholestatic liver injury (38). FXR can also induce ATP-binding cassette transporters G5 and G8 (ABCG5/ABCG8) to expel cholesterol, and induce multidrug resistance transporter 3 (MDR3 and ABCB4) to expel phospholipids into bile, forming mixed micelles with BAs, and BAs are stored in the gallbladder (38). Another pathway that regulates BA synthesis is dependent on the contribution of intestinal FXR. A high concentration of BA activates FXR in intestinal epithelial cells, which enhances the transcription and secretion of mouse FGF15 or human FGF19 that are then further transported into the liver via enterohepatic circulation (39). Subsequently, FGF15/FGF19 activates the intrahepatic FGFR4/β-Klotho complex, a transmembrane tyrosine kinase receptor located on the surface of hepatocytes, leading to the activation of JNK and ERK1/2 pathways, and the subsequent inhibition of the expression of CYP7A1 and CYP8B1, and the decreased synthesis of BA (39,40). This intestinal-liver signaling pathway may be the main mechanism of the FXR-negative feedback regulation of BA synthesis.
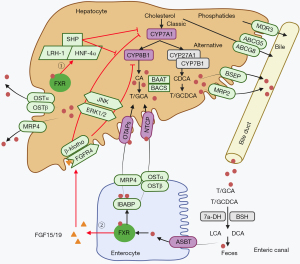
Lipid homeostasis
Recently, numerous studies have shown that FXR plays a significant role in not only in BA metabolism, but also in lipid metabolism. Overweight and obesity often cause adverse metabolic effects such as hypertension, high cholesterol, hyperlipidemia, and insulin resistance. Emerging evidence suggests that the specific targeting of FXR may be an effective way to treat obesity-induced metabolic diseases. Specifically, research has shown that inhibiting FXR activity in the small intestine can counteract obesity and improve metabolic disorders associated with diabetes and non-alcoholic fatty diseases (8-10).
Studies on FXR knockout mice have shown that the specific knockout of FXR in different organs and tissues exerts different effects. Systemic FXR-deficient mice (FXR−/−) had increased triglyceride and cholesterol concentrations, increased atherosclerotic lipid accumulation, and an excessive accumulation of fat in the liver, which suggests a that FXR has a protective function in lipid homeostasis (12,13). Similarly, liver-specific FXR deficiency mice showed aggravated HFD-induced obesity compared with wild type (WT) mice (14). However, a recent study showed that adipocyte-specific FXR deficiency can protect mouse adipose tissue from oxidative stress and insulin resistance, and exert a protective effect on HFD-induced obesity and fasting hyperglycemia, which suggests that FXR has a pathogenic role in metabolic disease (11). In summary, in regulating lipid metabolism, FXR shows tissue specificity. It appears that the specific knockout of FXR in the intestine and adipocytes reduces obesity and the side effects of obesity, while the specific knockout of FXR in the whole body and liver leads to excessive lipid accumulation and promotes obesity.
Inflammation and immunity
The anti-inflammatory effect of FXR is well known, and inhibitory inflammatory mechanisms can be divided into two types: direct and indirect. The direct mechanisms include controlling the activation and proliferation of immune cells (41-43). For example, FXR signaling has been shown to reduce intestinal inflammation by reducing the number of innate lymphoid cells, inflammatory responses mediated by marker transcription factors and functional cytokines (44). Indirect mechanisms include regulating BA metabolism or glucose and lipid metabolism to reduce the inflammatory response. For example, primary biliary cholangitis (PBC) causes immune disorders and cholestasis due to the imbalance of BA signaling pathways (45). However, the activation of FXR attenuates PBC by regulating BA metabolism and alleviating cholestasis (46).
In addition to its anti-inflammatory effects, FXR also exerts unexpected pro-inflammatory effects in adipose tissue. In the adipocyte-specific FXR deficient mouse model, under the condition of fat-induced HFD, adipocyte-specific FXR deficiency leads to the up-regulation of Gsta4 gene expression, an antioxidant enzyme that attenuates lipid peroxide produced during oxidative stress and its α,ꞵ-unsaturated aldehyde to protect cells from damage (47). At the same time, the infiltration of pro-inflammatory macrophages was reduced, and the levels of tumor necrosis factor-alpha (TNF-α) and interleukin-6 (IL-6) messenger RNA were decreased (11). In summary, the role of FXR in regulating the immune response is bidirectional, which may be attributed to differences in pathological conditions and inflammatory disease progression, all of which need to be further elucidated.
FXR in lung diseases
Recently, the discovery of FXR expression in lung tissue has spurred great interest in understanding the biological mechanisms of lung diseases. Chen et al. (48) and Francis et al. (49) found that FXR was expressed in type I and type II alveolar epithelial cells (AEC-I and AEC-II), alveolar macrophages, lung endothelial cells, and fibroblasts. Extensive research has shown that FXR participates in the pathogenesis of lung diseases such as lung injury (50-55), lung ischemia/reperfusion injury (LI/RI) (56), lung fibrosis (29,41,48,50,57-61), and lung cancer (62-67) (Figure 3). These observations suggest that FXR could serve as a novel potential target in the treatment of various lung diseases. In this review, we summarize the latest research on FXR in lung diseases.
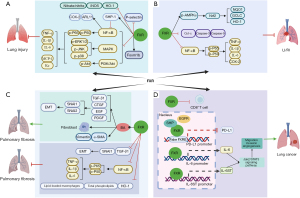
Lung injury
Acute lung injury (ALI) is an acute, diffuse, inflammatory lung injury caused by various intrapulmonary and extrapulmonary pathogenic factors (such as pneumonia, sepsis, shock, respiratory tract bacterial or viral infection), which leads to severe acute respiratory distress syndrome (ARDS). In severe cases, an inflammatory storm may develop that may lead to acute respiratory failure and death (68). ALI is mainly characterized by increased pulmonary vascular permeability, pulmonary edema, neutrophil isolation, and hypoxemia (69). ALI/ARDS is characterized by the activation of a range of inflammatory signaling pathways, and the release of typical inflammatory mediators such as TNF-α, interferon-γ (IFN-γ), IL-6, inducible nitric oxide synthase (iNOS), and monocyte chemotactic protein 1 (MCP-1) leading to pulmonary inflammatory response (55).
Several studies have confirmed that FXR plays an important role in decreasing the severity of ALI (Figure 3A). It has been reported that the protective role of FXR in ALI is mainly dependent on its anti-inflammatory effects. For example, the ligand-dependent activation of pulmonary FXR prevents lipopolysaccharide-induced ALI by inhibiting multiple signaling pathways, including the nuclear factor kappa-B (NF-κB), mitogen-activated protein kinase (MAPK), and phosphatidylinositol 3-kinase/protein kinase B (PI3K/Akt) signaling pathways (51). Despite its direct anti-inflammatory effects, FXR exerts an antioxidant effect in lung inflammation. A recent study showed that the orthocaspase (OCA)-mediated activation of FXR mitigates nitrogen mustard (NM) vesicant-induced pulmonary oxidative stress, which is known to cause ALI, by inhibiting the expression of antioxidant enzyme heme oxygenase-1 (HO-1), iNOS, and nitrate/nitrite in lung tissue (52). Conversely, the absence of FXR may directly or indirectly activate NF-κB in AEC-II through SHP-1, thereby increasing the expression of cyclooxygenase-2 (COX-2) and ADP-ribosylation factor-like protein 11 (ARL11) (53). Therefore, FXR can also reduce ALI by exerting antioxidant effects. OCA-mediated FXR activation has also been shown to promote lung repair after monocrotaline-induced lung injury by producing surfactants and promoting alveolar repair (61). In addition, FXR activation may reduce inflammation-induced lung injury by inhibiting the p-selectin-mediated recruitment of leukocytes and enhancing the proliferation of Foxm1b-mideated pulmonary vascular endothelium cells, which in turn improves lung permeability and inhibits leukocytes from circulating into inflammatory tissues, thus promoting lung repair (55). In summary, the mechanism by which FXR inhibits lung injury mainly involves weakening multiple inflammatory signaling pathways, exerting antioxidant effects, and improving pulmonary vascular endothelial permeability.
LI/RI
LI/RI is a common clinical issue caused by lung transplantation, extracorporeal circulation, and trauma, and usually leads to high morbidity and mortality. It is characterized by increased microvascular permeability and pulmonary vascular resistance, decreased lung compliance, oxygenation inhibition, pulmonary edema, and hypertension, and increased total protein and pro-inflammatory factors in bronchoalveolar lavage fluid (70,71). LI/RI has been shown to prolong the length of hospital stay, seriously affect patient prognosis, lead to pulmonary failure, and be life-threatening (72).
In recent years, researchers have explored the pathogenesis of LI/RI, and found that oxidative stress, apoptosis, and inflammation are the key factors leading to LI/RI (73,74). A more recent study showed that dioscin-mediated FXR activation alleviated LI/RI by regulating nuclear erythroid factor 2-related factor 2 (Nrf2)-mediated oxidative stress, cytochrome C mediated apoptosis, and NF-κB-mediated inflammation (56) (Figure 3B). However, to date, there has been little discussion of whether the regulatory mechanism of FXR is involved in LI/RI disease. Unlike the role of FXR in liver or myocardial ischemia-RI (75-78), which has been widely studied, the role of FXR in LI/RI has received little attention, and more research should be conducted in this field in the future.
Lung fibrosis
Pulmonary fibrosis is the final result of various diffuse lung parenchymal diseases, and is characterized by excessive extracellular matrix (ECM) deposition, which leads to the destruction of lung structure, and varying degrees of inflammation and fibrosis in lung parenchyma (57,79). The most common type of pulmonary fibrosis is idiopathic pulmonary fibrosis (IPF), which is characterized by chronic, progressive fibrosis, and progressive decline in lung function in interstitial pneumonia, which can lead to considerable morbidity and mortality (80,81). The typical lesion of IPF is fibroblast foci, which is mainly composed of α-smooth muscle actin (α-SMA)-positive myofibroblasts that secrete ECM components. The potential pathological mechanism of IPF is not yet fully understood; however, it IPF is thought to originate from repeated minor damage to the alveolar epithelium, followed by abnormal repair (82,83). Myofibroblasts are thought to cause the abnormal and excessive deposition of collagen and the structural remodeling observed in IPF (84). Myofibroblasts are derived from epithelial-mesenchymal transition (EMT) and fibroblast differentiation. The pathogenesis of IPF is mainly based on EMT, inflammatory process, and collagen deposition (85).
It is well known that the abnormal regulation of lipid homeostasis plays an important role in the occurrence of pulmonary fibrosis. HO-1 may contribute to the development of fibrotic M2 macrophages in the lung (86,87). Meshanni et al. found that OCA-mediated FXR activation inhibits the progression of NM-induced lung fibrosis by reducing the expression of HO-1 in the lung and restoring lipid homeostasis (52). In addition, Comeglio et al. found that the long-term use of OCA treatment significantly mitigates bleomycin-induced lung fibrosis by activating FXR in the lung, the mechanism involved in suppressing of the production of inflammatory factors (IL-1ꞵ and IL-6), and reducing the expression of transforming growth factor-β1 (TGF-β1) and zinc finger transcription factor 1 (SNAI1), which are responsible for activating the EMT process (29,41). In contrast, several studies have shown that FXR plays a role in promoting fibrosis. For example, Chen et al. found that FXR is up-regulated in proliferative AEC-II and is also located in the fibroblast foci of IPF lungs. In vitro, FXR can mediate BA-induced alveolar EMT and lung fibroblast activation in various cell lines (e.g., the human AEC-II cell line A549, the human fetal lung fibroblasts cell line WI-38, and the IPF-derived lung fibroblasts cell line LL-97A) (48). Specifically, in one study, A549 cells were induced to generate EMT after FXR activation by GW4064 stimulation, and FXR antagonist Z-guggulsterone or FXR knockdown significantly attenuated BA-induced α-SMA and vimentin in A549 cells (48). At the same time, in vivo, after BA inhalation, the expression of FXR in alveolar epithelium and pulmonary interstitial fibroblasts was promoted, and pulmonary fibrosis was induced in rats. It has been speculated that the up-regulation and activation of FXR may be involved in the process of BA micro-aspiration-induced fibrosis (57). The role of FXR in lung fibrosis remains controversial. However, after examining the mechanism by which FXR regulates lung fibrosis, this contradiction may be related to whether the function of FXR depends on ligand-mediated activation or simply the expression of FXR itself. It would appear that the expression of FXR itself directly exerts anti-inflammatory and anti-fibrotic effects. In high BA environments in vivo and in vitro, FXR can mediate BA-induced EMT and lung fibroblast activation, and promote α-SMA and vimentin production to indirectly promote fibrosis (Figure 3C).
Lung cancer
Lung cancer is the leading cause of cancer-related death in men and women worldwide (88). According to China’s national statistics, more than 600,000 people die from lung cancer every year (89). Lung cancer includes non-small cell lung cancer (NSCLC) and small cell lung cancer (SCLC) (90,91). NSCLC accounts for the majority of all lung cancer cases, and is a major cause of cancer-related deaths worldwide (92). Despite significant advances in targeted therapy over the years, the prognosis of NSCLC patients remains poor, with a 5-year survival rate of less than 20% (93).
It has been reported that FXR participates in the pathogenesis of numerous cancers, such as lung cancer, breast cancer, colorectal cancer, and liver cancer, but the mechanisms by which FXR regulates tumor progression are not always consistent, and are sometimes contradictory in different types of cancer (67,94-96). In recent years, researchers have begun to pay increasing attention to the role of FXR in lung cancer (Figure 3D). The expression of FXR is elevated in NSCLC cells and is associated with poor survival outcomes in patients (66). A recent study showed that highly selective FXR antagonists with chalcone inhibited NSCLC, which suggests that FXR activation has a carcinogenic effect in NSCLC (63). In addition, the overexpression of FXR in lung cancer has been shown to promote tumor growth by directly transactivating CCND1 in NSCLC (66). In addition to promoting local tumor growth, FXR has also been confirmed to promote the metastasis of NSCLC by the transactivation of IL-6ST and IL-6 genes, and the subsequent activation of the Janus kinases-2 (Jak2)/signal transducer and transcription-3 (STAT3) signaling pathway (62). In addition to having a direct effect on lung cancer cell growth and metastasis, FXR has also been shown to lead to the development of an immunosuppressive microenvironment in lung cancer, characterized by the increased infiltration of myeloid-derived suppressor cells and the decreased infiltration of anti-tumor lymphocyte populations [such as cytotoxic (CD8+) T cells]. A significant negative correlation was found between FXR and programmed death ligand 1 (PD-L1) expression in NSCLC specimens (65,67). Consistent with these observations, inhibiting the expression or activity of FXR with Z-guggulsterone was shown to exert anti-tumor effects by up-regulating the expression of PD-L1 in NSCLC (64). Further research revealed that this may be due to the fact that FXR inhibits PD-L1 transcription by binding to the presumptive FXRE element in the PD-L1 promoter via SHP and epidermal growth factor receptor signalling (67). In brief, these observations suggest that FXR plays a carcinogenic role in lung cancer by directly promoting tumor growth and metastasis, or constructing an immunosuppressive microenvironment by up-regulating PD-L1 expression. These findings strongly suggest that FXR could serve as a prognostic biomarker and therapeutic target for NSCLC.
Conclusions
As a BA-activated NR, FXR is involved in the body’s BA, lipid metabolism, and inflammatory response. It is not only expressed in the gastrointestinal tract, but is also expressed in the lung, the main organ of the respiratory system with an immune defense function. FXR activation participates in the immune response in pulmonary vascular endothelial cells, bronchial epithelial cells, alveolar epithelial cells, and alveolar macrophages. FXR also regulates the occurrence and progression of lung diseases by participating in the processes of the inflammatory response, oxidative stress, apoptosis, cancer cell proliferation, and metastasis, and the construction of the immunosuppressive microenvironment.
In summary, FXR is expressed in the lungs, and plays an important role in the development of lung diseases. However, the activation of FXR has different roles in different disease models, and FXR can exert dual effects even in the same lung disease. The dominant role of FXR in lung diseases is not clear; however, following further research on the mechanism of FXR in the pathogenesis of various lung diseases, the receptor could serve as an indicator of the progression of these diseases or could be used to design potential therapeutic targets.
Acknowledgments
Funding: The present study was supported by funding from
Footnote
Reporting Checklist: The authors have completed the Narrative Review reporting checklist (available at https://jtd.amegroups.com/article/view/10.21037/jtd-24-734/rc).
Peer Review File: Available at https://jtd.amegroups.com/article/view/10.21037/jtd-24-734/prf
Conflicts of Interest: All authors have completed the ICMJE uniform disclosure form (available at https://jtd.amegroups.com/article/view/10.21037/jtd-24-734/coif). The authors have no conflicts of interest to declare.
Ethical Statement: The authors are accountable for all aspects of the work in ensuring that questions related to the accuracy or integrity of any part of the work are appropriately investigated and resolved.
Open Access Statement: This is an Open Access article distributed in accordance with the Creative Commons Attribution-NonCommercial-NoDerivs 4.0 International License (CC BY-NC-ND 4.0), which permits the non-commercial replication and distribution of the article with the strict proviso that no changes or edits are made and the original work is properly cited (including links to both the formal publication through the relevant DOI and the license). See: https://creativecommons.org/licenses/by-nc-nd/4.0/.
References
- Arab JP, Karpen SJ, Dawson PA, et al. Bile acids and nonalcoholic fatty liver disease: Molecular insights and therapeutic perspectives. Hepatology 2017;65:350-62. [Crossref] [PubMed]
- Chiang JY, Kimmel R, Weinberger C, et al. Farnesoid X receptor responds to bile acids and represses cholesterol 7alpha-hydroxylase gene (CYP7A1) transcription. J Biol Chem 2000;275:10918-24. [Crossref] [PubMed]
- Ma K, Saha PK, Chan L, et al. Farnesoid X receptor is essential for normal glucose homeostasis. J Clin Invest 2006;116:1102-9. [Crossref] [PubMed]
- Al-Aqil FA, Monte MJ, Peleteiro-Vigil A, et al. Interaction of glucocorticoids with FXR/FGF19/FGF21-mediated ileum-liver crosstalk. Biochim Biophys Acta Mol Basis Dis 2018;1864:2927-37. [Crossref] [PubMed]
- Shapiro H, Kolodziejczyk AA, Halstuch D, et al. Bile acids in glucose metabolism in health and disease. J Exp Med 2018;215:383-96. [Crossref] [PubMed]
- Teodoro JS, Rolo AP, Palmeira CM. Hepatic FXR: key regulator of whole-body energy metabolism. Trends Endocrinol Metab 2011;22:458-66. [Crossref] [PubMed]
- Wang H, He Q, Wang G, et al. FXR modulators for enterohepatic and metabolic diseases. Expert Opin Ther Pat 2018;28:765-82. [Crossref] [PubMed]
- Jiang C, Xie C, Li F, et al. Intestinal farnesoid X receptor signaling promotes nonalcoholic fatty liver disease. J Clin Invest 2015;125:386-402. [Crossref] [PubMed]
- Li F, Jiang C, Krausz KW, et al. Microbiome remodelling leads to inhibition of intestinal farnesoid X receptor signalling and decreased obesity. Nat Commun 2013;4:2384. [Crossref] [PubMed]
- Sonne DP. Mechanisms in endocrinology: FXR signalling: a novel target in metabolic diseases. Eur J Endocrinol 2021;184:R193-205. [Crossref] [PubMed]
- Dehondt H, Marino A, Butruille L, et al. Adipocyte-specific FXR-deficiency protects adipose tissue from oxidative stress and insulin resistance and improves glucose homeostasis. Mol Metab 2023;69:101686. [Crossref] [PubMed]
- Lambert G, Amar MJ, Guo G, et al. The farnesoid X-receptor is an essential regulator of cholesterol homeostasis. J Biol Chem 2003;278:2563-70. [Crossref] [PubMed]
- Sinal CJ, Tohkin M, Miyata M, et al. Targeted disruption of the nuclear receptor FXR/BAR impairs bile acid and lipid homeostasis. Cell 2000;102:731-44. [Crossref] [PubMed]
- Prawitt J, Abdelkarim M, Stroeve JH, et al. Farnesoid X receptor deficiency improves glucose homeostasis in mouse models of obesity. Diabetes 2011;60:1861-71. [Crossref] [PubMed]
- Zheng Y, Sun W, Wang Z, et al. Activation of Pancreatic Acinar FXR Protects against Pancreatitis via Osgin1-Mediated Restoration of Efficient Autophagy. Research (Wash D C) 2022;2022:9784081. [Crossref] [PubMed]
- Porter BA, Ortiz MA, Bratslavsky G, et al. Structure and Function of the Nuclear Receptor Superfamily and Current Targeted Therapies of Prostate Cancer. Cancers (Basel) 2019;11:1852. [Crossref] [PubMed]
- Olsson MA, García-Sosa AT, Ryde U. Binding affinities of the farnesoid X receptor in the D3R Grand Challenge 2 estimated by free-energy perturbation and docking. J Comput Aided Mol Des 2018;32:211-24. [Crossref] [PubMed]
- Weikum ER, Liu X, Ortlund EA. The nuclear receptor superfamily: A structural perspective. Protein Sci 2018;27:1876-92. [Crossref] [PubMed]
- Lee FY, Lee H, Hubbert ML, et al. FXR, a multipurpose nuclear receptor. Trends Biochem Sci 2006;31:572-80. [Crossref] [PubMed]
- Huber RM, Murphy K, Miao B, et al. Generation of multiple farnesoid-X-receptor isoforms through the use of alternative promoters. Gene 2002;290:35-43. [Crossref] [PubMed]
- Zhang Y, Kast-Woelbern HR, Edwards PA. Natural structural variants of the nuclear receptor farnesoid X receptor affect transcriptional activation. J Biol Chem 2003;278:104-10. [Crossref] [PubMed]
- Tian SY, Chen SM, Pan CX, et al. FXR: structures, biology, and drug development for NASH and fibrosis diseases. Acta Pharmacol Sin 2022;43:1120-32. [Crossref] [PubMed]
- Wang YD, Chen WD, Moore DD, et al. FXR: a metabolic regulator and cell protector. Cell Res 2008;18:1087-95. [Crossref] [PubMed]
- Preidis GA, Kim KH, Moore DD. Nutrient-sensing nuclear receptors PPARα and FXR control liver energy balance. J Clin Invest 2017;127:1193-201. [Crossref] [PubMed]
- Friedman ES, Li Y, Shen TD, et al. FXR-Dependent Modulation of the Human Small Intestinal Microbiome by the Bile Acid Derivative Obeticholic Acid. Gastroenterology 2018;155:1741-1752.e5. [Crossref] [PubMed]
- Herman-Edelstein M, Weinstein T, Levi M. Bile acid receptors and the kidney. Curr Opin Nephrol Hypertens 2018;27:56-62. [Crossref] [PubMed]
- Lee H, Zhang Y, Lee FY, et al. FXR regulates organic solute transporters alpha and beta in the adrenal gland, kidney, and intestine. J Lipid Res 2006;47:201-14. [Crossref] [PubMed]
- Zhang R, Peng L, Ran H, et al. Farnesoid X Receptor Activation Modulates Calcium Homeostasis in Rat Aortic Vascular Smooth Muscle Cells. Chin J Physiol 2018;61:210-20. [Crossref] [PubMed]
- Comeglio P, Morelli A, Adorini L, et al. Beneficial effects of bile acid receptor agonists in pulmonary disease models. Expert Opin Investig Drugs 2017;26:1215-28. [Crossref] [PubMed]
- Hiebl V, Ladurner A, Latkolik S, et al. Natural products as modulators of the nuclear receptors and metabolic sensors LXR, FXR and RXR. Biotechnol Adv 2018;36:1657-98. [Crossref] [PubMed]
- Zheng W, Lu Y, Tian S, et al. Structural insights into the heterodimeric complex of the nuclear receptors FXR and RXR. J Biol Chem 2018;293:12535-41. [Crossref] [PubMed]
- Chávez-Talavera O, Haas J, Grzych G, et al. Bile acid alterations in nonalcoholic fatty liver disease, obesity, insulin resistance and type 2 diabetes: what do the human studies tell? Curr Opin Lipidol 2019;30:244-54. [Crossref] [PubMed]
- Han CY. Update on FXR Biology: Promising Therapeutic Target? Int J Mol Sci 2018;19:2069. [Crossref] [PubMed]
- Keitel V, Dröge C, Häussinger D. Targeting FXR in Cholestasis. Handb Exp Pharmacol 2019;256:299-324. [Crossref] [PubMed]
- Choi YJ, Yang HS, Zhang Y, et al. Intratracheal exposure to polyhexamethylene guanidine phosphate disrupts coordinate regulation of FXR-SHP-mediated cholesterol and bile acid homeostasis in mouse liver. Ecotoxicol Environ Saf 2022;247:114213. [Crossref] [PubMed]
- Fiorucci S, Distrutti E, Biagioli M, Special FX. Harnessing the Farnesoid-X-Receptor to Control Bile Acid Synthesis. Dig Dis Sci 2021;66:3668-71. [Crossref] [PubMed]
- Ji S, Liu Q, Zhang S, et al. FGF15 Activates Hippo Signaling to Suppress Bile Acid Metabolism and Liver Tumorigenesis. Dev Cell 2019;48:460-474.e9. [Crossref] [PubMed]
- Chiang JYL, Ferrell JM. Bile acid receptors FXR and TGR5 signaling in fatty liver diseases and therapy. Am J Physiol Gastrointest Liver Physiol 2020;318:G554-73. [Crossref] [PubMed]
- Inagaki T, Choi M, Moschetta A, et al. Fibroblast growth factor 15 functions as an enterohepatic signal to regulate bile acid homeostasis. Cell Metab 2005;2:217-25. [Crossref] [PubMed]
- Kong B, Wang L, Chiang JY, et al. Mechanism of tissue-specific farnesoid X receptor in suppressing the expression of genes in bile-acid synthesis in mice. Hepatology 2012;56:1034-43. [Crossref] [PubMed]
- Comeglio P, Filippi S, Sarchielli E, et al. Anti-fibrotic effects of chronic treatment with the selective FXR agonist obeticholic acid in the bleomycin-induced rat model of pulmonary fibrosis. J Steroid Biochem Mol Biol 2017;168:26-37. [Crossref] [PubMed]
- Verbeke L, Mannaerts I, Schierwagen R, et al. FXR agonist obeticholic acid reduces hepatic inflammation and fibrosis in a rat model of toxic cirrhosis. Sci Rep 2016;6:33453. [Crossref] [PubMed]
- Zhang Y, Xu Y, Qi Y, et al. Protective effects of dioscin against doxorubicin-induced nephrotoxicity via adjusting FXR-mediated oxidative stress and inflammation. Toxicology 2017;378:53-64. [Crossref] [PubMed]
- Fu T, Li Y, Oh TG, et al. FXR mediates ILC-intrinsic responses to intestinal inflammation. Proc Natl Acad Sci U S A 2022;119:e2213041119. [Crossref] [PubMed]
- Gulamhusein AF, Hirschfield GM. Primary biliary cholangitis: pathogenesis and therapeutic opportunities. Nat Rev Gastroenterol Hepatol 2020;17:93-110. [Crossref] [PubMed]
- Nevens F, Andreone P, Mazzella G, et al. A Placebo-Controlled Trial of Obeticholic Acid in Primary Biliary Cholangitis. N Engl J Med 2016;375:631-43. [Crossref] [PubMed]
- Awasthi YC, Ramana KV, Chaudhary P, et al. Regulatory roles of glutathione-S-transferases and 4-hydroxynonenal in stress-mediated signaling and toxicity. Free Radic Biol Med 2017;111:235-43. [Crossref] [PubMed]
- Chen B, Cai HR, Xue S, et al. Bile acids induce activation of alveolar epithelial cells and lung fibroblasts through farnesoid X receptor-dependent and independent pathways. Respirology 2016;21:1075-80. [Crossref] [PubMed]
- Francis M, Guo G, Kong B, et al. Regulation of Lung Macrophage Activation and Oxidative Stress Following Ozone Exposure by Farnesoid X Receptor. Toxicol Sci 2020;177:441-53. [Crossref] [PubMed]
- Comeglio P, Filippi S, Sarchielli E, et al. Therapeutic effects of the selective farnesoid X receptor agonist obeticholic acid in a monocrotaline-induced pulmonary hypertension rat model. J Endocrinol Invest 2019;42:951-65. [Crossref] [PubMed]
- Fei J, Fu L, Hu B, et al. Obeticholic acid alleviate lipopolysaccharide-induced acute lung injury via its anti-inflammatory effects in mice. Int Immunopharmacol 2019;66:177-84. [Crossref] [PubMed]
- Meshanni JA, Lee JM, Vayas KN, et al. Suppression of Lung Oxidative Stress, Inflammation, and Fibrosis following Nitrogen Mustard Exposure by the Selective Farnesoid X Receptor Agonist Obeticholic Acid. J Pharmacol Exp Ther 2024;388:586-95. [Crossref] [PubMed]
- Murray A, Banota T, Guo GL, et al. Farnesoid X receptor regulates lung macrophage activation and injury following nitrogen mustard exposure. Toxicol Appl Pharmacol 2022;454:116208. [Crossref] [PubMed]
- Yang D, Liang H, Zhu X, et al. Farnesoid X Receptor Protects Murine Lung against IL-6-promoted Ferroptosis Induced by Polyriboinosinic-Polyribocytidylic Acid. Am J Respir Cell Mol Biol 2024;70:364-78. [Crossref] [PubMed]
- Zhang L, Li T, Yu D, et al. FXR protects lung from lipopolysaccharide-induced acute injury. Mol Endocrinol 2012;26:27-36. [Crossref] [PubMed]
- Dong L, Yin L, Li R, et al. Dioscin alleviates lung ischemia/reperfusion injury by regulating FXR-mediated oxidative stress, apoptosis, and inflammation. Eur J Pharmacol 2021;908:174321. [Crossref] [PubMed]
- Chen B, You WJ, Liu XQ, et al. Chronic microaspiration of bile acids induces lung fibrosis through multiple mechanisms in rats. Clin Sci (Lond) 2017;131:951-63. [Crossref] [PubMed]
- Dou JY, Cui ZY, Xuan MY, et al. Diallyl disulfide, the bioactive component of Allium species, ameliorates pulmonary fibrosis by mediating the crosstalk of farnesoid X receptor and yes-associated protein 1 signaling pathway. Phytother Res 2024;38:4009-21. [Crossref] [PubMed]
- Liu Y, Wang C, Chen H, et al. The profibrotic effects of chronic microaspiration of bile acids on lungs of rats at different stages. Int Immunopharmacol 2020;84:106545. [Crossref] [PubMed]
- Venosa A, Smith LC, Murray A, et al. Regulation of Macrophage Foam Cell Formation During Nitrogen Mustard (NM)-Induced Pulmonary Fibrosis by Lung Lipids. Toxicol Sci 2019;172:344-58. [Crossref] [PubMed]
- Vignozzi L, Morelli A, Cellai I, et al. Cardiopulmonary protective effects of the selective FXR agonist obeticholic acid in the rat model of monocrotaline-induced pulmonary hypertension. J Steroid Biochem Mol Biol 2017;165:277-92. [Crossref] [PubMed]
- Jin X, Shang B, Wang J, et al. Farnesoid X receptor promotes non-small cell lung cancer metastasis by activating Jak2/STAT3 signaling via transactivation of IL-6ST and IL-6 genes. Cell Death Dis 2024;15:148. [Crossref] [PubMed]
- Niu S, Zhang G, Wang N, et al. Structure Modification of FXR Antagonistic Chalcones and Their Inhibitory Effects on NSCLC Cell Proliferation and Metastasis. ChemMedChem 2022;17:e202100778. [Crossref] [PubMed]
- Tian H, Gui Y, Wei Y, et al. Z-guggulsterone induces PD-L1 upregulation partly mediated by FXR, Akt and Erk1/2 signaling pathways in non-small cell lung cancer. Int Immunopharmacol 2021;93:107395. [Crossref] [PubMed]
- Wang L, Xu X, Shang B, et al. High farnesoid X receptor expression predicts favorable clinical outcomes in PD L1(low/negative) non small cell lung cancer patients receiving anti PD 1 based chemo immunotherapy. Int J Oncol 2022;60:40. [Crossref] [PubMed]
- You W, Chen B, Liu X, et al. Farnesoid X receptor, a novel proto-oncogene in non-small cell lung cancer, promotes tumor growth via directly transactivating CCND1. Sci Rep 2017;7:591. [Crossref] [PubMed]
- You W, Li L, Sun D, et al. Farnesoid X Receptor Constructs an Immunosuppressive Microenvironment and Sensitizes FXR(high)PD-L1(low) NSCLC to Anti-PD-1 Immunotherapy. Cancer Immunol Res 2019;7:990-1000. [Crossref] [PubMed]
- Johnson ER, Matthay MA. Acute lung injury: epidemiology, pathogenesis, and treatment. J Aerosol Med Pulm Drug Deliv 2010;23:243-52. [Crossref] [PubMed]
- Weyker PD, Webb CA, Kiamanesh D, et al. Lung ischemia reperfusion injury: a bench-to-bedside review. Semin Cardiothorac Vasc Anesth 2013;17:28-43. [Crossref] [PubMed]
- Lan CC, Peng CK, Tang SE, et al. Inhibition of Na-K-Cl cotransporter isoform 1 reduces lung injury induced by ischemia-reperfusion. J Thorac Cardiovasc Surg 2017;153:206-15. [Crossref] [PubMed]
- Zhang M, Jin F. 1α,25-Dihydroxyvitamin D3 Ameliorates Seawater Aspiration-Induced Lung Injury By Inhibiting The Translocation Of NF-κB and RhoA. Inflammation 2017;40:832-9. [Crossref] [PubMed]
- Diamond JM, Arcasoy S, McDonnough JA, et al. Adipose Gene Expression Profile Changes With Lung Allograft Reperfusion. Am J Transplant 2017;17:239-45. [Crossref] [PubMed]
- Capuzzimati M, Hough O, Liu M. Cell death and ischemia-reperfusion injury in lung transplantation. J Heart Lung Transplant 2022;41:1003-13. [Crossref] [PubMed]
- Wang H, Zhang Y, Bai R, et al. Baicalin Attenuates Alcoholic Liver Injury through Modulation of Hepatic Oxidative Stress, Inflammation and Sonic Hedgehog Pathway in Rats. Cell Physiol Biochem 2016;39:1129-40. [Crossref] [PubMed]
- Gao Y, Zhao Y, Yuan A, et al. Effects of farnesoid-X-receptor SUMOylation mutation on myocardial ischemia/reperfusion injury in mice. Exp Cell Res 2018;371:301-10. [Crossref] [PubMed]
- Liu H, Wang J, Ding Y, et al. Antibiotic pretreatment attenuates liver ischemia-reperfusion injury by Farnesoid X receptor activation. Cell Death Dis 2022;13:484. [Crossref] [PubMed]
- Pu J, Yuan A, Shan P, et al. Cardiomyocyte-expressed farnesoid-X-receptor is a novel apoptosis mediator and contributes to myocardial ischaemia/reperfusion injury. Eur Heart J 2013;34:1834-45. [Crossref] [PubMed]
- Zhao Q, Wang X, Liu K, et al. Activation of farnesoid X receptor enhances the efficacy of normothermic machine perfusion in ameliorating liver ischemia-reperfusion injury. Am J Transplant 2024;24:1610-22. [Crossref] [PubMed]
- Parimon T, Hohmann MS, Yao C. Cellular Senescence: Pathogenic Mechanisms in Lung Fibrosis. Int J Mol Sci 2021;22:6214. [Crossref] [PubMed]
- Lederer DJ, Martinez FJ. Idiopathic Pulmonary Fibrosis. N Engl J Med 2018;379:797-8. [Crossref] [PubMed]
- Sgalla G, Biffi A, Richeldi L. Idiopathic pulmonary fibrosis: Diagnosis, epidemiology and natural history. Respirology 2016;21:427-37. [Crossref] [PubMed]
- Crestani B, Marchand-Adam S, Fabre A, et al. Mechanisms in pulmonary fibrosis. Rev Prat 2007;57:2222-6.
- King TE Jr, Pardo A, Selman M. Idiopathic pulmonary fibrosis. Lancet 2011;378:1949-61. [Crossref] [PubMed]
- Phan SH. The myofibroblast in pulmonary fibrosis. Chest 2002;122:286S-9S. [Crossref] [PubMed]
- Zhu L, Fu X, Chen X, et al. M2 macrophages induce EMT through the TGF-β/Smad2 signaling pathway. Cell Biol Int 2017;41:960-8. [Crossref] [PubMed]
- Campbell NK, Fitzgerald HK, Dunne A. Regulation of inflammation by the antioxidant haem oxygenase 1. Nat Rev Immunol 2021;21:411-25. [Crossref] [PubMed]
- Rossi M, Korpak K, Doerfler A, et al. Deciphering the Role of Heme Oxygenase-1 (HO-1) Expressing Macrophages in Renal Ischemia-Reperfusion Injury. Biomedicines 2021;9:306. [Crossref] [PubMed]
- Wu F, Wang L, Zhou C. Lung cancer in China: current and prospect. Curr Opin Oncol 2021;33:40-6. [Crossref] [PubMed]
- Zheng RS, Sun KX, Zhang SW, et al. Report of cancer epidemiology in China, 2015. Zhonghua Zhong Liu Za Zhi 2019;41:19-28. [Crossref] [PubMed]
- Bordoloi D, Banik K, Padmavathi G, et al. TIPE2 Induced the Proliferation, Survival, and Migration of Lung Cancer Cells Through Modulation of Akt/mTOR/NF-κB Signaling Cascade. Biomolecules 2019;9:836. [Crossref] [PubMed]
- Bordoloi D, Banik K, Vikkurthi R, et al. Inflection of Akt/mTOR/STAT-3 cascade in TNF-α induced protein 8 mediated human lung carcinogenesis. Life Sci 2020;262:118475. [Crossref] [PubMed]
- Siegel RL, Miller KD, Jemal A. Cancer statistics, 2019. CA Cancer J Clin 2019;69:7-34. [Crossref] [PubMed]
- Wang T, Nelson RA, Bogardus A, et al. Five-year lung cancer survival: which advanced stage nonsmall cell lung cancer patients attain long-term survival? Cancer 2010;116:1518-25. [Crossref] [PubMed]
- Fu T, Coulter S, Yoshihara E, et al. FXR Regulates Intestinal Cancer Stem Cell Proliferation. Cell 2019;176:1098-1112.e18. [Crossref] [PubMed]
- Girisa S, Henamayee S, Parama D, et al. Targeting Farnesoid X receptor (FXR) for developing novel therapeutics against cancer. Mol Biomed 2021;2:21. [Crossref] [PubMed]
- Kainuma M, Takada I, Makishima M, et al. Farnesoid X Receptor Activation Enhances Transforming Growth Factor β-Induced Epithelial-Mesenchymal Transition in Hepatocellular Carcinoma Cells. Int J Mol Sci 2018;19:1898. [Crossref] [PubMed]