Investigation of causal associations between cerebral cortical structure and Barrett’s esophagus: insights from Mendelian randomization and meta-analysis
Highlight box
Key findings
• Our study identifies specific variations in cerebral cortical structure that are associated with the risk of Barrett’s esophagus (BE), using Mendelian randomization (MR) and meta-analysis.
What is known and what is new?
• BE has been linked to several risk factors, but the potential neurobiological contributions to its development, particularly involving the brain-gut axis, remain underexplored.
• Our study is among the first to investigate the causal relationship between cortical structure variations and BE risk, pinpointing cortical regions that may either protect against or increase BE risk.
What is the implication, and what should change now?
• The findings suggest that incorporating neuroimaging biomarkers could enhance BE risk assessment, potentially leading to more personalized screening and intervention strategies. Future research should explore integrating neurobiological insights into clinical practice, which could transform BE prevention and management, offering more tailored diagnostic and therapeutic approaches.
Introduction
Barrett’s esophagus (BE) is a condition of significant clinical importance, characterized by the metaplastic transformation of the distal esophagus’s normal squamous epithelium into a columnar lining (1,2). This alteration is not merely a benign morphological curiosity but a recognized precursor to esophageal adenocarcinoma (EAC), a malignancy with a notoriously poor prognosis. The insidious nature of BE often means it goes undetected until advanced stages, as its symptoms frequently overlap with those of gastroesophageal reflux disease (GERD), including persistent heartburn, regurgitation, and occasionally dysphagia (3). The pathogenesis of BE is multifactorial, with chronic exposure to gastric acid and bile reflux identified as key etiological factors. Genetic predispositions also play a critical role, underlining the condition’s complex nature (4-6). Epidemiologically, BE predominantly affects males and individuals of Caucasian descent, contributing to a significant disease burden. Current research efforts face challenges in early detection, precise risk stratification, and the development of effective strategies to prevent progression to EAC (7,8).
The intersection of neurobiology and gastroenterology has recently emerged as a promising frontier in medical research, broadening our understanding of diseases beyond their traditional organ-specific contexts. One of the most intriguing developments in this field is the exploration of the gut-brain axis—a complex, bidirectional communication network that integrates signals between the central nervous system and the gastrointestinal tract (9). This axis involves neural, hormonal, and immune pathways that regulate digestive processes and influence the onset and progression of various diseases, including BE (10-12). Intriguingly, contemporary research underscores the potential of cortical structures in modulating gastrointestinal functions, suggesting that these brain regions could significantly influence the progression and management of BE through this multifaceted neural network.
Recent studies have suggested that the cerebral cortex, particularly regions involved in processing visceral sensations and regulating emotional responses, may play a critical role in modulating gastrointestinal functions through this gut-brain axis (13-15). Additionally, investigating the associations between cerebral cortical structure and BE pioneers a novel paradigm within this interdisciplinary research frontier (16,17).
Despite the growing body of evidence supporting the involvement of cortical structures in gastrointestinal diseases, the exact nature and extent of these associations remain controversial. While some studies have demonstrated strong links between changes in cortical structure and the severity of gastrointestinal conditions, others have found minimal or no association (18,19). This discrepancy highlights the need for further research to clarify these relationships and determine whether cortical changes are a cause or consequence of gastrointestinal pathology. Several potential confounding factors may contribute to these conflicting findings. Variability in study populations, differences in methodologies, and the presence of comorbid conditions like GERD could all affect the observed associations between cortical structures and BE. Additionally, lifestyle factors, such as diet and stress, which impact both brain and gut health, might confound these relationships (20).
Understanding the role of the brain-gut axis in BE could open new avenues for treatment, particularly for patients whose disease is refractory to conventional therapies. By exploring these relationships, we aim to offer a fresh perspective on the etiology and progression of BE, potentially leading to innovative approaches in diagnosis, treatment, and prevention. Such insights could redefine traditional views on BE, positioning neurobiological factors at the forefront of future research and therapeutic strategies.
Mendelian randomization (MR) offers a robust approach to addressing these challenges by utilizing genetic variants as instrumental variables (IVs) to infer causality between modifiable risk factors and health outcomes. MR takes advantage of the random allocation of alleles at conception, mimicking the randomization process in controlled trials, which helps mitigate confounding factors and biases inherent to observational studies (21,22). Rooted in the principles of genetic epidemiology, MR utilizes genetic variants as IVs to infer causal relationships between modifiable risk factors and health outcomes. This method leverages the random allocation of alleles at conception, akin to the randomization process in controlled trials, thereby mitigating confounding factors and biases inherent to observational studies (23-25). The advantages of MR in medical research are manifold. Primarily, it provides a framework for the identification of therapeutic targets by establishing causality rather than mere association. Furthermore, MR can elucidate the direction and magnitude of disease risk factors, offering insights into the biological mechanisms driving disease progression.
The primary objective of this study is to apply the principles of MR to explore the potential causal relationship between cortical structure—a quantifiable marker of neurobiological integrity—and the risk of developing BE. By delving into this novel area of investigation, the study seeks to uncover previously unexplored neurobiological factors that may contribute to BE’s pathogenesis. Ultimately, this research aims to enrich our understanding of BE, offering new avenues for early intervention, risk assessment, and personalized treatment strategies, thereby addressing the pressing need for improved management and outcomes in patients with BE. We present this article in accordance with the STROBE-MR reporting checklist (available at https://jtd.amegroups.com/article/view/10.21037/jtd-24-698/rc).
Methods
Study design
The investigation into the causal relationship between cortical structure and BE utilizes a MR approach. This method employs genetic variants as IVs to elucidate causal relationships between a modifiable exposure and an outcome (26). The MR study design is based on three key principles: relevance, exclusivity, and independence:
- The genetic variants chosen as IVs must be strongly associated with the exposure of interest. This association guarantees that the genetic variants can serve as dependable proxies for the exposure (27);
- Additionally, the genetic variants used should be independent of any confounding factors that may also influence the risk of BE. This assumption is based on the random assortment of genes from parents to offspring, which mimics the random allocation in a clinical trial (28);
- The relationship between the IVs and the health outcome must operate exclusively through the exposure, according to the principle of exclusivity. This means that any influence the genetic variants have on BE risk must be mediated through exposure and not through other pathways. Figure 1 illustrates the overall research design (25).
Selection of IV
In this study, single nucleotide polymorphisms (SNPs) were employed as IVs to explore the causal connections between variations in cortical structure and BE. Our approach to selecting SNPs was meticulously structured, leveraging a multi-tiered screening protocol grounded in stringent criteria to ensure the robustness and reliability of our findings:
- Initial SNP selection: utilizing the R software (version 4.3.1), the first step involved identifying SNPs associated with characteristics of cortical structure. This process adhered to a predefined significance threshold of P<5×10−6, ensuring that only SNPs with a strong statistical link to cortical structure were considered.
- Ensuring independence: to maintain the independence of our IVs, we screened for and eliminated SNPs exhibiting linkage disequilibrium (LD) with an r2=0.001 within a 10,000 kb radius. This critical step was taken to prevent the confounding effects of correlated genetic variants, thereby enhancing the integrity of our IV analysis.
- Exclusion of confounding-linked SNPs: the PhenoScanner database, a comprehensive resource for exploring SNP associations, was utilized to sift through and exclude SNPs associated with known confounders or direct outcomes related to BE. This precaution helped to mitigate the influence of external factors that could potentially skew the causal interpretation of our results.
- Instrumental strength verification: to further refine our selection, the instrumental strength of each SNP was quantified using the F statistic, with SNPs exhibiting an F statistic <10 being excluded. This criterion ensured that only SNPs with sufficient instrumental strength were included in our analysis, minimizing weak instrument bias (22).
This rigorous SNP screening process is designed to ensure the validity and reliability of the IVs employed in our analysis. Through this comprehensive methodology, we have established a solid foundation for exploring the causal dynamics between cortical structure variations and the development of BE.
Cortical structure genome-wide association study (GWAS) data sources
The GWAS data on cortical structure, focusing on surface area (SA) and thickness (TH), were sourced from the ENIGMA Consortium (https://enigma.ini.usc.edu). The study utilized magnetic resonance imaging (MRI) technology to gather and analyze brain imaging and genetic data from 51,665 participants, primarily of European descent, distributed across 60 global cohorts (29). Critically, the data have been meticulously adjusted to account for global genetic influences, ensuring the genetic correlations (LD score rG) derived are specific to regional variations, isolating these from overarching genetic background effects (30,31). This rigorous adjustment facilitates a more accurate analysis of the genetic architecture affecting cortical metrics within the human population.
Outcome data sources
The outcome variable was the presence of BE, identified using the ICD-10 code K22.7. Data for BE cases and controls were sourced from two prominent GWAS databases: the IEU Open GWAS database (available at https://gwas.mrcieu.ac.uk), which provided data on 13,358 BE cases and 43,071 controls, and the FinnGen database (available at https://r10.finngen.fi), which included data on 1,259 BE cases and 350,064 controls. The extensive datasets from these two databases allowed for robust statistical analysis and ensured a comprehensive assessment of the associations between cortical structures and BE risk.
Statistical analysis
R software version 4.3.1 was employed for all analyses.
The causal relationship between the exposure and outcome was analyzed using “TwoSampleMR” and “MR-PRESSO” packages (32,33), employing methods like inverse variance weighted (IVW), constrained maximum likelihood (cML), weighted median and mode-based estimations (34-38). Cochran’s Q statistic assessed IV heterogeneity, leading to a shift to random-effects IVW if significant variability was detected (39). The MR-Egger method addressed horizontal pleiotropy, indicated by its intercept, while MR-PRESSO identified and corrected for pleiotropic outliers. Visual validations through funnel and scatter plots confirmed the analysis’s robustness, and the Steiger test verified the directionality of SNP associations, ensuring a precise and streamlined causal inference (40). Correlation heatmaps were plotted with ChiPlot (https://www.chiplot.online/).
A meta-analysis was performed to synthesize the effect sizes from the MR analyses, providing a more robust estimate of the causal relationship between cortical structure and BE. This was done using the “meta” package in R (version 6.5.0), which facilitated the combination of results across studies. Both fixed-effect and random-effects models were employed to account for potential variability between datasets. The fixed-effect model assumes that the true effect size is consistent across all studies, making it suitable when minimal heterogeneity is expected. In contrast, the random-effects model accounts for potential differences in effect sizes across populations, recognizing that the true effect might vary between different datasets. To evaluate the degree of heterogeneity, Cochran’s Q statistic was calculated, and the I² statistic was used to quantify the proportion of variation attributable to heterogeneity rather than chance. When significant heterogeneity was identified, the random-effects model was prioritized to ensure the results reflected this variability. In cases where heterogeneity was minimal, results from both the fixed-effect and random-effects models were reported to provide a more comprehensive understanding of the data (41,42).
Ethical statement
The study was conducted in accordance with the Declaration of Helsinki (as revised in 2013). All data utilized in this study were derived from publicly available GWAS datasets that have received ethical approval from their respective institutional review boards. All participants provided informed consent, and no new data were collected directly from individuals for this analysis. We made sure that all studies had approval from their institutional review boards to ensure participants were fully informed and consented.
Results
A thorough two-sample MR analysis, mainly using the IVW method, was performed to investigate the causal relationship between with SA and TH of brain region or full cortex and BE.
Exploration of the causal effect of cortical structure on BE (FinnGen)
https://cdn.amegroups.cn/static/public/jtd-24-698-1.xlsx shows the full cortex causal effect on the BE. The SA and TH of full cortex had no causal relationship with BE [odds ratio (OR)SA =0.93, PSA=0.60; ORTH =15.49, PSA=0.15]. Figures 2,3 illustrate the significant estimates between the TH and SA of different brain regions and BE. The analysis revealed that increased cortical TH in the banks of the superior temporal sulcus (bankssts) correlates with a decreased risk of developing BE (ORbankssts =0.36, Beta=−1.03, Pbankssts=0.002), suggesting that a thicker cortex in this region may serve as a protective barrier against the disease. Similarly, the cortical TH within the parahippocampal region showed a protective effect against BE (ORparahippocampal =0.61, Beta=−0.50, Pparahippocampal=0.03), indicating that greater cortical integrity here could mitigate the risk.
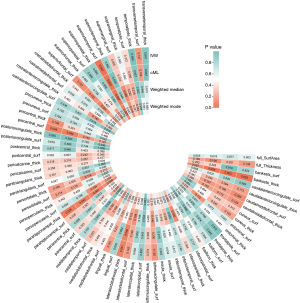
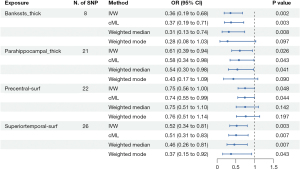
Furthermore, a larger SA in the superior temporal region is negatively correlated with BE risk (ORsuperiortemporal =0.52, Beta=−0.65, Psuperiortemporal=0.003), emphasizing its role in potentially safeguarding against the condition. The cortical SA in the precentral region also exhibited a protective association with BE (ORprecentral =0.75, Beta=−0.23, P=0.04), reinforcing the notion that larger cortical SAs in specific brain regions could contribute to lower BE risk. These findings illuminate the nuanced and significant protective factors that specific cortical structures may offer in the context of BE.
Through additional analyses and sensitivity checks utilizing three alternative methods, we further confirmed the strength of the causal associations we observed (available online: https://cdn.amegroups.cn/static/public/jtd-24-698-1.xlsx, Tables S1). In specific, the MR-Egger intercept and the MR-PRESSO global test successfully mitigated concerns related to horizontal pleiotropy, thus enhancing the credibility of our results, and the Steiger directionality test found no reverse causation. The utilization of scatter plots and funnel plots as visual aids facilitated the interpretation of our findings (Figures 4,5). The leave-one-out analysis provided additional confidence by demonstrating that no SNP introduced a pleiotropic bias into the causal estimates (Figure 6).
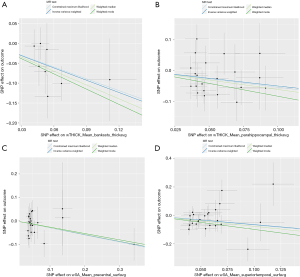
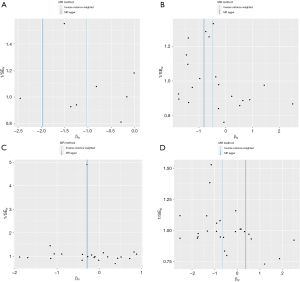
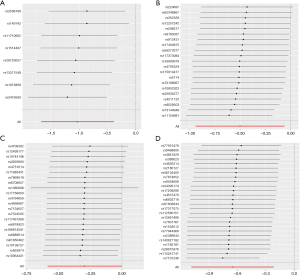
Investigating cortical structure’s causal effect on BE (IEU OpenGWAS)
In parallel analyses using the IEU OpenGWAS database, our investigation further explored the relationship between cortical structure and BE. Globally, a negative correlation between the SA of the full cortex and BE suggests that a larger full cortical SA may act as a protective factor against the disease, while the TH of the full cortex showed no causal relationship with BE (ORSA =0.61, PSA=0.002; ORTH =0.72, PSA=0.75).
Further, our detailed exploration of specific brain regions identified a variety of associations: increased cortical SA in the bankssts and the medial orbitofrontal cortex were both found to reduce the risk of developing BE (ORbankssts =0.66, Beta=−0.42, Pbankssts=0.01; ORmedialorbitofrontal =0.65, Beta=−0.43, Pmedialorbitofrontal=0.01), signifying their potential protective capabilities. Additionally, greater cortical TH in the supramarginal and pars orbitalis regions also correlated negatively with BE (ORsupramarginal=0.68, Beta=−0.39, Psupramarginal=0.02; ORparsorbitalis =0.58, Beta=−0.54, Pparsorbitalis=0.006), enhancing their protective profile against the condition. Conversely, an increase in cortical SA in the middle temporal and precuneus regions was positively correlated with BE (ORmiddletemporal =1.32, Beta=0.28, Pmiddletemporal=0.01; ORprecuneus =1.33, Beta=0.29, Pprecuneus=0.03), suggesting these areas may increase the susceptibility to BE. These comprehensive results underscore the significant role of cortical structural variations across different brain regions in modulating the risk of BE, illustrating a complex interplay between protective and risk-enhancing cortical attributes. https://cdn.amegroups.cn/static/public/jtd-24-698-2.xlsx and Figures 7,8 exhibit the findings of the above.
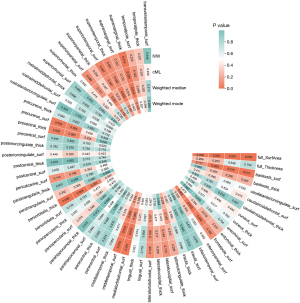
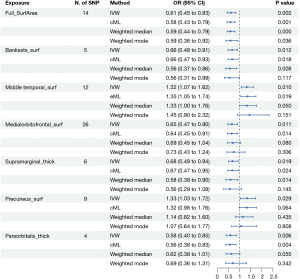
In the sensitivity analyses for the negative correlation between the full cortex SA and BE, significant heterogeneity and horizontal pleiotropy were detected, suggesting variability in causal estimates and potential alternative pathways influencing this association. However, the leave-one-out test confirmed the robustness of this finding, indicating no undue influence from any single study. For other cortical metrics—cortical SA in the bankssts and medial orbitofrontal, and cortical TH in the supramarginal and pars orbitalis regions—no significant heterogeneity or horizontal pleiotropy was found, affirming the consistency of these negative correlations with BE. Positive correlations between BE and cortical SA in the middle temporal and precuneus also passed these tests. All results across different cortical structures successfully passed the Steiger test, validating the directionality and appropriateness of the genetic instruments used, thereby reinforcing the integrity and reliability of our causal inferences. The above results are shown in Tables S2,S3. Using scatterplots and funnel plots as visual aids facilitated interpretation of our results (Figures 9,10). By demonstrating that no SNP introduced a pleiotropic bias into the causal estimates, the leave-one-out analysis provided additional confidence (Figure 11).
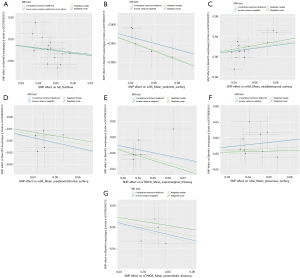
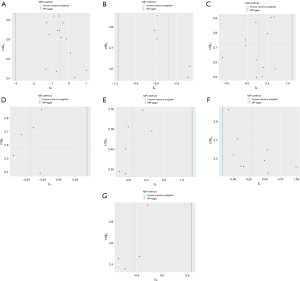

Meta-analysis of combined database results
After conducting separate analyses using data from the IEU OpenGWAS and FinnGen databases, a meta-analysis was performed to combine the findings and assess overall effect sizes and statistical significance across both datasets. This approach aimed to evaluate the consistency and reliability of the positive associations identified in the individual analyses. The meta-analysis confirmed that three associations remained statistically significant across both datasets. Specifically, the results showed that increased SA in the middle temporal gyrus was associated with a higher risk of BE, with a pooled odds ratio (POR) of 1.3034 [95% confidence interval (CI): 1.08–1.57, P=0.006]. Conversely, increased cortical TH in both the supramarginal gyrus (POR =0.71, 95% CI: 0.53–0.95, P=0.02) and the pars orbitalis (POR =0.61, 95% CI: 0.45–0.84, P=0.002) was associated with a reduced risk of BE. Across these analyses, heterogeneity was minimal, with I2 values less than 25%, supporting the use of a fixed-effect model in each case. These findings suggest that while increased SA in certain cortical regions may elevate the risk of BE, increased cortical TH in other regions could provide a protective effect (Figure 12).
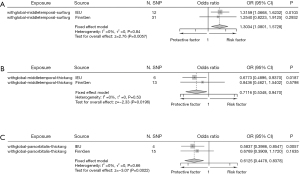
For the remaining results that initially appeared significant, the subsequent meta-analysis did not maintain statistical significance, with P values exceeding the 0.05 threshold (Figure 13). Although the preliminary MR analyses indicated positive associations, the pooled effect sizes from these regions in the meta-analysis showed no significant relationship with BE. Heterogeneity, as indicated by I2 values ranging from 54% to 88%, was a major factor in necessitating the use of random-effects models throughout these analyses.
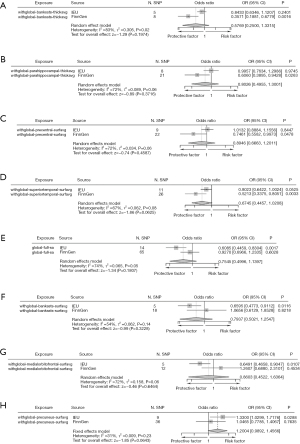
These findings underscore the importance of using meta-analytic approaches to validate the robustness of associations across multiple studies. They further highlight the necessity of exploring the specific mechanisms through which cortical structures in these regions might influence the risk of developing BE. Although the meta-analysis did not confirm the statistical significance of these associations, the presence of substantial heterogeneity, particularly in analyses requiring the use of random-effects models, may have influenced these outcomes. As a result, these findings point to the need for further research to clarify the underlying neurobiological mechanisms and to address the potential variability across studies.
Discussion
In our MR analysis, we explored the associations between various cortical structures and BE, employing data from two distinct GWAS databases. Initial findings from the MR analysis revealed several statistically significant associations indicating both protective and risk-enhancing effects of different cortical metrics on BE.
However, upon integrating these results through meta-analysis, some of these associations did not maintain statistical significance. Specifically, only the associations involving cortical TH in the supramarginal and pars orbitalis, and the cortical SA in the middle temporal region remained significant. Increased cortical TH in the supramarginal and pars orbitalis regions was associated with a decreased risk of developing BE, indicating a protective role. Conversely, an increased SA in the middle temporal region was associated with a higher risk, suggesting a potential risk factor for BE. This discrepancy likely reflects the robustness of these findings across diverse populations and methodologies, underlining the complexity of the genetic architecture influencing BE.
The supramarginal and pars orbitalis regions are integral components of the cerebral cortex, each playing vital roles in cognitive and emotional processing, which are critical for effective human interaction and complex social behaviors (43,44). The supramarginal gyrus, nestled within the parietal lobe, is fundamental for language perception, processing, empathy, and the intricate mechanisms of pain perception (45,46). Simultaneously, the pars orbitalis, a significant segment of the inferior frontal gyrus in the frontal lobe, engages primarily in nuanced language processing, social cognition, sophisticated decision-making processes, and the regulation of emotions (47-49). Both areas are susceptible to changes influenced by a myriad of factors including developmental trajectories, educational and environmental stressors, and the progression of neurodegenerative diseases, each of which can profoundly impact their structural integrity and functional capacities.
There appears to be a negative correlation between increased cortical TH in these regions and the reduced risk of BE, suggesting that enhanced neural processing capabilities in these areas may offer protection against gastrointestinal disturbances often exacerbated by chronic stress and emotional dysregulation—factors known to aggravate GERD, a precursor to BE (50,51). Furthermore, the pivotal roles these cortical regions play in pain perception and emotional regulation might facilitate more effective responses to esophageal discomfort, potentially prompting earlier medical intervention and mitigating the severity of BE (47,52). Despite existing research that connects alterations in brain morphology within areas responsible for managing stress and pain to various chronic conditions, direct investigations linking cortical TH in the supramarginal and pars orbitalis regions specifically to BE remain scarce. This gap highlights a critical area for future inquiry, where exploring how targeted cognitive therapies and stress reduction interventions designed to enhance cortical health could emerge as innovative preventive or therapeutic strategies for managing BE.
Conversely, the consistent positive correlation between cortical SA in the middle temporal region and BE underscores the potential risk-enhancing role of alterations in this area. The middle temporal region of the brain, integral to auditory processing and language comprehension, is crucial for recognizing and processing visual motion information (53,54). Changes in this region’s cortical SA, influenced by genetic predispositions, developmental factors, environmental impacts and aging, are implicated in the regulation of stress responses and visceral pain, critical factors in the pathophysiology of gastrointestinal conditions (55,56). The increased SA may enhance sensitivity to visceral pain and discomfort associated with esophageal inflammation or acid exposure, exacerbating the symptoms of gastroesophageal reflux, which can escalate the progression of BE (52,57).
Moreover, the role of the middle temporal region in stress processing may link its increased cortical SA to heightened stress responsiveness, potentially aggravating inflammation and altering esophageal motility (57). This interaction could promote the reflux of acidic contents into the esophagus, contributing to mucosal damage and the advancement of BE. These speculative mechanisms align with current neurogastroenterology research emphasizing the brain-gut axis as a vital pathway in understanding and managing digestive diseases (11,20).
For statistically significant results in the initial MR analysis that were not sustained in the meta-analysis, it’s crucial to consider the potential roles these findings might still play in the pathogenesis of BE. These initial findings could reflect real, albeit subtle, influences on BE that did not reach statistical significance when tested across larger, more diverse datasets in the meta-analysis. This variation underscores the complex interplay of genetic and environmental factors that influence BE, and suggests that these cortical structures might affect certain aspects of the disease’s progression or manifest in individual susceptibility differences.
The insights from our study into the associations between specific cortical structures and BE underscore the potential for enhancing clinical management, particularly through improved screening and innovative intervention strategies. Integrating neuroimaging markers, such as alterations in cortical TH in regions like the supramarginal and pars orbitalis, into screening protocols could greatly refine the identification of individuals at different risk levels for BE. This approach would not only personalize surveillance schedules but could also identify those who might benefit from earlier and more frequent endoscopic evaluations. Furthermore, understanding the implications of increased cortical SA in regions such as the middle temporal might inform the development of targeted therapeutic approaches aimed at modulating stress responses and visceral hypersensitivity, factors known to exacerbate gastrointestinal symptoms. By incorporating these neurobiological insights into the management of BE, we can move towards a more holistic approach that not only aligns with the brain-gut axis model but also enhances the multidisciplinary collaboration between neurologists, psychologists, and gastroenterologists.
The clinical implications of these findings are twofold. First, our findings suggest that neurobiological factors, specifically changes in cortical structure, may be involved in the risk of developing BE. This insight could pave the way for new clinical research and risk stratification, where cortical imaging may serve as a complementary diagnostic tool to identify individuals at higher risk for BE, particularly when combined with traditional risk factors such as GERD and genetic predisposition. Second, understanding the specific cortical regions involved in BE risk could aid in developing therapeutic strategies targeting the brain-gut axis, potentially altering BE progression or reducing its risk.
We effectively leverage MR and meta-analysis to elucidate the links between cortical structures and BE, utilizing robust methodologies that minimize the confounding typical in observational studies and enhance the statistical robustness and generalizability of our findings. Despite these strengths, the study is constrained by potential biases. One significant limitation is the inability to perform a sex-specific meta-analysis due to some dataset’s lack of sex-specific data. Sex differences are a critical aspect of BE research, and future studies should prioritize datasets that include comprehensive sex-specific information. Furthermore, the homogeneity of the data, primarily from individuals of European descent, may limit the applicability of these findings to more diverse populations.
The results of our study strongly support the gastrointestinal-brain axis hypothesis, suggesting that future research should explore this relationship further, particularly through longitudinal studies that monitor changes in cortical structures over time in relation to BE progression. Such studies could deepen our understanding of the temporal dynamics influencing this axis. Experimental research is also needed to validate the biological mechanisms that we have proposed, potentially leading to novel physical therapy interventions aiming at modulating these neurobiological pathways to manage or prevent BE (9). Furthermore, to enhance the validity and applicability of these findings, future studies should aim to include more diverse populations. This will not only help in verifying the current results across different genetic and environmental backgrounds but also assist in uncovering how these variables interact to influence disease outcomes, thereby broadening the impact of the research and its clinical translation.
Conclusions
Our study conclusively links variations in cortical structure to the risk of BE, enhancing our understanding of the gastrointestinal-brain axis. By utilizing MR and meta-analysis, we have pinpointed specific cortical areas that either mitigate or heighten BE risk. These findings highlight the potential for incorporating neuroimaging biomarkers into BE management, enabling more personalized approaches to diagnosis and treatment. The integration of neuroscientific insights into gastroenterological practice could lead to more effective screening strategies and therapeutic interventions, ultimately improving patient outcomes and transforming the care of BE.
Acknowledgments
We thank all the participants and researchers who contributed to the GWAS and provided the summary data.
Funding: None.
Footnote
Reporting Checklist: The authors have completed the STROBE-MR reporting checklist. Available at https://jtd.amegroups.com/article/view/10.21037/jtd-24-698/rc
Peer Review File: Available at https://jtd.amegroups.com/article/view/10.21037/jtd-24-698/prf
Conflicts of Interest: All authors have completed the ICMJE uniform disclosure form (available at https://jtd.amegroups.com/article/view/10.21037/jtd-24-698/coif). The authors have no conflicts of interest to declare.
Ethical Statement: The authors are accountable for all aspects of the work in ensuring that questions related to the accuracy or integrity of any part of the work are appropriately investigated and resolved. The study was conducted in accordance with the Declaration of Helsinki (as revised in 2013). We used summary-level data from publicly available GWAS studies which have received ethical approval from their respective institutional review boards and informed consent from all participants. No administrative permissions were required to access the data.
Open Access Statement: This is an Open Access article distributed in accordance with the Creative Commons Attribution-NonCommercial-NoDerivs 4.0 International License (CC BY-NC-ND 4.0), which permits the non-commercial replication and distribution of the article with the strict proviso that no changes or edits are made and the original work is properly cited (including links to both the formal publication through the relevant DOI and the license). See: https://creativecommons.org/licenses/by-nc-nd/4.0/.
References
- Jain S, Dhingra S. Pathology of esophageal cancer and Barrett's esophagus. Ann Cardiothorac Surg 2017;6:99-109. [Crossref] [PubMed]
- Schoofs N, Bisschops R, Prenen H. Progression of Barrett's esophagus toward esophageal adenocarcinoma: an overview. Ann Gastroenterol 2017;30:1-6. [Crossref] [PubMed]
- Cook MB, Drahos J, Wood S, et al. Pathogenesis and progression of oesophageal adenocarcinoma varies by prior diagnosis of Barrett's oesophagus. Br J Cancer 2016;115:1383-90. [Crossref] [PubMed]
- Wiseman EF, Ang YS. Risk factors for neoplastic progression in Barrett's esophagus. World J Gastroenterol 2011;17:3672-83. [Crossref] [PubMed]
- Asanuma K, Iijima K, Shimosegawa T. Gender difference in gastro-esophageal reflux diseases. World J Gastroenterol 2016;22:1800-10. [Crossref] [PubMed]
- Frederiks CN, van Munster SN, Weusten BLAM. Goals of endoscopic eradication therapy in Barrett’s esophagus: a narrative review. Ann Esophagus 2022;5:5.
- Stawinski PM, Dziadkowiec KN, Kuo LA, et al. Barrett's Esophagus: An Updated Review. Diagnostics (Basel) 2023;13:321. [Crossref] [PubMed]
- Kolb JM, Wani S. Barrett's esophagus: current standards in advanced imaging. Transl Gastroenterol Hepatol 2021;6:14. [Crossref] [PubMed]
- Srinivasan S, Desai M. Long term care after successful endoscopic therapy in Barrett’s esophagus patients: a review of literature. Ann Esophagus 2022;5:8.
- Gershon MD, Margolis KG. The gut, its microbiome, and the brain: connections and communications. J Clin Invest 2021;131:e143768. [Crossref] [PubMed]
- Martin CR, Osadchiy V, Kalani A, et al. The Brain-Gut-Microbiome Axis. Cell Mol Gastroenterol Hepatol 2018;6:133-48. [Crossref] [PubMed]
- Banfi D, Moro E, Bosi A, et al. Impact of Microbial Metabolites on Microbiota-Gut-Brain Axis in Inflammatory Bowel Disease. Int J Mol Sci 2021;22:1623. [Crossref] [PubMed]
- Bonaz B, Sinniger V, Pellissier S. The Vagus Nerve in the Neuro-Immune Axis: Implications in the Pathology of the Gastrointestinal Tract. Front Immunol 2017;8:1452. [Crossref] [PubMed]
- Mayer EA, Tillisch K. The brain-gut axis in abdominal pain syndromes. Annu Rev Med 2011;62:381-96. [Crossref] [PubMed]
- Keefer L, Ballou SK, Drossman DA, et al. A Rome Working Team Report on Brain-Gut Behavior Therapies for Disorders of Gut-Brain Interaction. Gastroenterology 2022;162:300-15. [Crossref] [PubMed]
- Kharrazian D. Traumatic Brain Injury and the Effect on the Brain-Gut Axis. Altern Ther Health Med 2015;21:28-32.
- Araújo LA, Silva LR, Mendes FAA. Controle neuronal e manifestações digestórias na paralisia cerebral. Jornal de Pediatria 2012;88:455-64. [Crossref] [PubMed]
- Nesheiwat G, Carr R, Molena D, et al. Treatment of Barrett’s esophagus: a narrative review. Ann Esophagus 2022;5:44.
- Ratcliffe E, Liew Y, Kuan J, et al. Dedicated services for Barrett’s esophagus—a survey and service assessment of provision in United Kingdom hospitals. Ann Esophagus 2023;6:39.
- Majka J, Wierdak M, Brzozowska I, et al. Melatonin in Prevention of the Sequence from Reflux Esophagitis to Barrett's Esophagus and Esophageal Adenocarcinoma: Experimental and Clinical Perspectives. Int J Mol Sci 2018;19:2033. [Crossref] [PubMed]
- Timpson NJ, Wade KH, Smith GD. Mendelian randomization: application to cardiovascular disease. Curr Hypertens Rep 2012;14:29-37. [Crossref] [PubMed]
- Davies NM, Holmes MV, Davey Smith G. Reading Mendelian randomisation studies: a guide, glossary, and checklist for clinicians. BMJ 2018;362:k601. [Crossref] [PubMed]
- Tian D, Zhang L, Zhuang Z, et al. A two-sample Mendelian randomization analysis of modifiable risk factors and intracranial aneurysms. Sci Rep 2022;12:7659. [Crossref] [PubMed]
- Smith GD, Ebrahim S. 'Mendelian randomization': can genetic epidemiology contribute to understanding environmental determinants of disease?. Int J Epidemiol 2003;32:1-22. [Crossref] [PubMed]
- Lawlor DA, Harbord RM, Sterne JA, et al. Mendelian randomization: using genes as instruments for making causal inferences in epidemiology. Stat Med 2008;27:1133-63. [Crossref] [PubMed]
- Burgess S, Daniel RM, Butterworth AS, et al. Network Mendelian randomization: using genetic variants as instrumental variables to investigate mediation in causal pathways. Int J Epidemiol 2015;44:484-95. [Crossref] [PubMed]
- Meng YA, Yu Y, Cupples LA, et al. Performance of random forest when SNPs are in linkage disequilibrium. BMC Bioinformatics 2009;10:78. [Crossref] [PubMed]
- de Bakker PI, Burtt NP, Graham RR, et al. Transferability of tag SNPs in genetic association studies in multiple populations. Nat Genet 2006;38:1298-303. [Crossref] [PubMed]
- Grasby KL, Jahanshad N, Painter JN, et al. The genetic architecture of the human cerebral cortex. Science 2020;367:eaay6690. [Crossref] [PubMed]
- Bulik-Sullivan BK, Loh PR, Finucane HK, et al. LD Score regression distinguishes confounding from polygenicity in genome-wide association studies. Nat Genet 2015;47:291-5. [Crossref] [PubMed]
- Bulik-Sullivan B, Finucane HK, Anttila V, et al. An atlas of genetic correlations across human diseases and traits. Nat Genet 2015;47:1236-41. [Crossref] [PubMed]
- Yavorska OO, Burgess S. MendelianRandomization: an R package for performing Mendelian randomization analyses using summarized data. Int J Epidemiol 2017;46:1734-9. [Crossref] [PubMed]
- Ong JS, MacGregor S. Implementing MR-PRESSO and GCTA-GSMR for pleiotropy assessment in Mendelian randomization studies from a practitioner's perspective. Genet Epidemiol 2019;43:609-16. [Crossref] [PubMed]
- Yin Q, Zhu L. Does co-localization analysis reinforce the results of Mendelian randomization? Brain 2024;147:e7-8. [Crossref] [PubMed]
- Burgess S, Scott RA, Timpson NJ, et al. Using published data in Mendelian randomization: a blueprint for efficient identification of causal risk factors. Eur J Epidemiol 2015;30:543-52. [Crossref] [PubMed]
- Burgess S, Butterworth A, Thompson SG. Mendelian randomization analysis with multiple genetic variants using summarized data. Genet Epidemiol 2013;37:658-65. [Crossref] [PubMed]
- Bowden J, Davey Smith G, Haycock PC, et al. Consistent Estimation in Mendelian Randomization with Some Invalid Instruments Using a Weighted Median Estimator. Genet Epidemiol 2016;40:304-14. [Crossref] [PubMed]
- Hartwig FP, Davey Smith G, Bowden J. Robust inference in summary data Mendelian randomization via the zero modal pleiotropy assumption. Int J Epidemiol 2017;46:1985-98. [Crossref] [PubMed]
- Kulinskaya E, Dollinger MB, Bjørkestøl K. On the moments of Cochran's Q statistic under the null hypothesis, with application to the meta-analysis of risk difference. Res Synth Methods 2011;2:254-70. Erratum in: Res Synth Methods 2020;11:920. [Crossref] [PubMed]
- Lutz SM, Voorhies K, Wu AC, et al. The influence of unmeasured confounding on the MR Steiger approach. Genet Epidemiol 2022;46:139-41. [Crossref] [PubMed]
- Wang W, Huang M, Ge W, et al. Identifying serum metabolite biomarkers for autoimmune diseases: a two-sample mendelian randomization and meta-analysis. Front Immunol 2024;15:1300457. [Crossref] [PubMed]
- Zou M, Liang Q, Zhang W, et al. Diet-derived circulating antioxidants and risk of inflammatory bowel disease: a Mendelian randomization study and meta-analysis. Front Immunol 2024;15:1334395. [Crossref] [PubMed]
- Watson CG, DeMaster D, Ewing-Cobbs L. Graph theory analysis of DTI tractography in children with traumatic injury. Neuroimage Clin 2019;21:101673. [Crossref] [PubMed]
- Tomic A, Agosta F, Sarasso E, et al. Are there two different forms of functional dystonia? A multimodal brain structural MRI study. Mol Psychiatry 2020;25:3350-9. [Crossref] [PubMed]
- Barbaro MF, Kramer DR, Nune G, et al. Directional tuning during reach planning in the supramarginal gyrus using local field potentials. J Clin Neurosci 2019;64:214-9. [Crossref] [PubMed]
- Isernia S, Blasi V, Baglio G, et al. The key role of depression and supramarginal gyrus in frailty: a cross-sectional study. Front Aging Neurosci 2023;15:1292417. [Crossref] [PubMed]
- Pressman PS, Noniyeva Y, Bott N, et al. Comparing Volume Loss in Neuroanatomical Regions of Emotion versus Regions of Cognition in Healthy Aging. PLoS One 2016;11:e0158187. [Crossref] [PubMed]
- Schroeter ML, Vogt B, Frisch S, et al. Dissociating behavioral disorders in early dementia-An FDG-PET study. Psychiatry Res 2011;194:235-44. [Crossref] [PubMed]
- Wei Y, Bresser T, Wassing R, et al. Brain structural connectivity network alterations in insomnia disorder reveal a central role of the right angular gyrus. Neuroimage Clin 2019;24:102019. [Crossref] [PubMed]
- Kushner BS, Awad MM, Mikami DJ, et al. Endoscopic treatments for GERD. Ann N Y Acad Sci 2020;1482:121-9. [Crossref] [PubMed]
- Chhabra P, Ingole N. Gastroesophageal Reflux Disease (GERD): Highlighting Diagnosis, Treatment, and Lifestyle Changes. Cureus 2022;14:e28563. [Crossref] [PubMed]
- Ozzoude M, Varriano B, Beaton D, et al. White matter hyperintensities and smaller cortical thickness are associated with neuropsychiatric symptoms in neurodegenerative and cerebrovascular diseases. Alzheimers Res Ther 2023;15:114. [Crossref] [PubMed]
- Sykes JM. Applied anatomy of the temporal region and forehead for injectable fillers. J Drugs Dermatol 2009;8:s24-7.
- van Staalduinen EK, Zeineh MM. Medial Temporal Lobe Anatomy. Neuroimaging Clin N Am 2022;32:475-89. [Crossref] [PubMed]
- Liu C, Zhu S, Zhang J, et al. Inflammatory bowel diseases, interleukin-6 and interleukin-6 receptor subunit alpha in causal association with cerebral cortical structure: a Mendelian randomization analysis. Front Immunol 2023;14:1154746. [Crossref] [PubMed]
- Thomann AK, Thomann PA, Wolf RC, et al. Altered Markers of Brain Development in Crohn's Disease with Extraintestinal Manifestations - A Pilot Study. PLoS One 2016;11:e0163202. [Crossref] [PubMed]
- Ali S, Bailey A, Ash S, et al. A Pilot Study on Automatic Three-Dimensional Quantification of Barrett's Esophagus for Risk Stratification and Therapy Monitoring. Gastroenterology 2021;161:865-878.e8. [Crossref] [PubMed]