Efficacy and safety of immune checkpoint inhibitors combined with iodine-125 seed implantation in driver gene-negative non-small cell lung cancer: a retrospective cohort study
Highlight box
Key findings
• Compared to radiotherapy (RT) in combination with chemotherapy (CT) and immunotherapy, patients with driver gene-negative non-small cell lung cancer who received iodine-125 (I125) seed implantation had greater advantages with longer survival and fewer adverse effects.
What is known and what is new?
• Clinical evidence supports the substantial benefits of integrating RT with immunotherapy. Compared to RT, I125 seed implantation offers a precise, less invasive alternative, minimizing damage to surrounding lung tissues and reducing side effects.
• This manuscript evaluated the safety and efficacy of I125 seed implantation combined with immune checkpoint inhibitors and CT in treating driver gene-negative non-small cell lung cancer patients.
What is the implication, and what should change now?
• A higher-quality prospective cohort study needs to be established to reduce bias and further enhance the reliability of the results.
Introduction
Background
Non-small cell lung cancer (NSCLC) presents the most common type of lung cancer, accounting for 80–85% of cases. Immune checkpoint inhibitors (ICIs) are now the first-line standard of care for patients with driver gene-negative advanced and locally advanced lung cancers (1), and combination therapy based on ICIs in combination with chemotherapy (CT) has improved lung cancer patients’ survival rates.
Combining immunotherapy with radiotherapy (RT) has emerged as a significant research area in recent years. Radiotherapy can enhance antigen presentation and programmed death 1 ligand 1 (PD-L1) expression in tumor cells, augmenting the efficacy of ICIs. Conversely, ICIs might ameliorate the tumor’s hypoxic environment and enhance its radiosensitivity, potentially amplifying radiotherapy’s long-term benefits (2,3). Clinical evidence supports the substantial benefits of integrating radiotherapy with immunotherapy (4-7).
However, the risk of radiation pneumonitis, especially in lung cancer patients, poses a significant concern (8). Iodine-125 (I125) seed implantation offers a precise, less invasive alternative, minimizing damage to surrounding lung tissues and reducing side effects (9,10). While Wang et al.’s study (11) demonstrates the effectiveness and safety of combining I125 seed implantation with CT in treating advanced NSCLC patients, research on combining CT, ICIs, and I125 seed implantation remains limited (12-14).
Objective
This study aims to evaluate the safety and efficacy of I125 seed implantation combined with ICIs and CT versus external RT plus CT and ICIs in treating driver gene-negative NSCLC patients, offering insights into potentially safer and more effective treatment options and multidisciplinary approaches to lung cancer therapy. We present this article in accordance with the STROBE reporting checklist (available at https://jtd.amegroups.com/article/view/10.21037/jtd-24-1403/rc).
Methods
Setting
Retrospective analysis of 95 patients with driver gene-negative NSCLC who visited the First Affiliated Hospital of Army Medical University between December 2018 and December 2023 was conducted, including 33 patients treated with I125 seed implantation in combination with CT and ICIs (observation group) and 62 patients treated with extracorporeal RT in combination with CT and ICIs (control group).
The criteria for inclusion were: (I) age greater than 18 years; (II) pathologically confirmed NSCLC with negative driver genes; (III) no prior treatment (other than surgery or radiofrequency ablation) regarding this tumor; (IV) Eastern Cooperative Oncology Group (ECOG) score of 0–1; and (V) at least two cycles of ICI therapy. The criteria for exclusion were: (I) concurrent primary malignancy at other sites; (II) concurrent I125 seed implantation and RT during first-line treatment; (III) presence of a serious disease affecting treatment or survival as assessed by the investigator; (IV) incomplete clinical data; (V) participation in an randomized controlled trial (RCT) clinical study; and (VI) combination of symptomatic brain metastases. The study was conducted in accordance with the Declaration of Helsinki (as revised in 2013). The study was approved by the Ethics Committee of the First Affiliated Hospital of Army Medical University (No. KY2024028). Medical records obtained in previous clinical treatment were collected and analyzed retrospectively, individual consent for this study was waived.
I125 seed implantation: complete blood routine, coagulation function, and electrocardiogram (ECG) to exclude contraindications. To locate the tumor and identify the surgical site, as well as the direction and depth of needle entry, a preoperative computed tomography scan was carried out. The radioactive particle treatment planning system (TPS) system is used to determine the radiation dose and number of seeds. I125 seeds are implanted by TPS after a puncture needle is injected into the tumor tissue under computed tomography guidance. The gap between the puncture needles is typically 1 to 1.5 cm. Once all of the tips have reached the tumor’s distal edge, the I125 seeds are implanted by folding the tips back along the same distance or by TPS. Throughout the procedure, closely monitor the patient’s heart rate, blood pressure, and oxygen saturation, observe the patient’s consciousness, breathing, and symptoms (including pain, cough, or hemoptysis), and treat if necessary (15). After implantation, the entire lung is rescanned to verify the distribution and quantity of I125 seeds in each plane. Additionally, look for any problems, such as bleeding or pneumothorax, and treat if necessary.
Study design
Evaluation and follow-up
The results of the last imaging test performed before the initiation of treatment served as the baseline. The evaluation included a review of the efficacy and adverse events, and the follow-up data included information from our medical records and publicly available out-of-hospital data. The efficacy was assessed using the RECIST 1.1 criteria, and the results were divided into four grades: complete response (CR), partial response (PR), stable disease (SD), and progression disease (PD).
Observational indicators
Progression-free survival (PFS) was the primary observation endpoint, while PFS rate and adverse event rate were the secondary endpoints. PFS was defined as the time from the start of treatment to the first progression of the tumor or death from any cause (censored data were terminated by the last meaningful imaging), and overall survival (OS) was defined as the time from the start of tumor diagnosis to the patient’s death (censored data were terminated by the last follow-up). The safety analysis was based on medical records and examination test results, and it was graded using Common Terminology Criteria for Adverse Events (CTCAE) 5.0.
Statistical methods
Data analysis was conducted using SPSS 26.0 and R 4.2.2. Baseline characteristics between groups were compared using the Chi-squared test (or Fisher’s exact test) and the t-test (or Mann-Whitney U test). Cox regression analysis was applied for survival analysis. The Kaplan-Meier method constructed subgroup PFS curves to compare the PFS rates between groups, with the log-rank test evaluating differences. The restricted mean survival time (RMST) was calculated for both groups. The incidence of adverse events was compared using the Chi-squared test (or Fisher’s exact test), considering P<0.05 as statistically significant.
Results
Patient population
As of December 31, 2023, the median follow-up for the observation group was 23.6 [95% confidence interval (CI): 18.792–28.408] months, with 13 cases (39.4%) experiencing an endpoint event (progression after first-line treatment). In contrast, the control group had a median follow-up of 28.5 (95% CI: 24.334–32.666) months, with 52 cases (83.9%) encountering an endpoint event. Fifteen patients were lost to follow-up, including eleven from the control group and four from the observation group. Despite these differences, baseline characteristics between the two groups were comparable and statistically insignificant (P>0.05), as shown in Table 1, which outlines the distribution of baseline characteristics.
Table 1
Baseline characteristics | Observation group (n=33) | Control group (n=62) | P value |
---|---|---|---|
Age (years) | 60.18±8.39 | 60.77±8.47 | 0.69 |
Gender | 0.19 | ||
Male | 29 (87.9) | 59 (95.2) | |
Female | 4 (12.1) | 3 (4.8) | |
Postoperative recurrence† | 0.35 | ||
Yes | 3 (9.1) | 3 (4.8) | |
No | 30 (90.9) | 59 (95.2) | |
T stage | 0.34 | ||
T1–2 | 8 (24.2) | 19 (30.6) | |
T3–4 | 25 (75.8) | 43 (69.4) | |
N stage | 0.38 | ||
N0 | 8 (24.2) | 12 (19.4) | |
N1 | 25 (75.8) | 50 (80.6) | |
M stage | 0.54 | ||
M0 | 15 (45.5) | 29 (46.8) | |
M1 | 18 (54.5) | 33 (53.2) | |
AJCC stage | 0.57 | ||
Stage I & II | 2 (6.1) | 3 (4.8) | |
Stage III & IV | 31 (93.9) | 59 (95.2) | |
Pathological type | 0.23 | ||
Squamous carcinoma | 25 (75.8) | 41 (66.1) | |
Non-squamous carcinoma | 8 (24.2) | 21 (33.9) | |
Smoking index (pack-years) | 0.59 | ||
≤400 | 9 (27.3) | 17 (27.4) | |
>400 | 24 (72.7) | 45 (72.6) | |
Pulmonary disease | 0.55 | ||
Yes | 12 (36.4) | 22 (35.5) | |
No | 21 (63.6) | 40 (64.5) | |
Anti-angiogenesis therapy | 0.13 | ||
Yes | 10 (30.3) | 11 (17.7) | |
No | 23 (69.7) | 51 (82.3) |
Data are presented as mean ± standard deviation or number (%). †, refers to the primary lesion’s surgical excision and recurrence following systemic therapy. AJCC, American Joint Committee on Cancer.
Comparison of PFS between two groups
The control group’s median PFS was 11.8 months (95% CI: 9.743–13.857), whereas the observation group did not reach a median PFS, indicating a significant difference (log-rank test, P=0.001) as illustrated in Figure 1. The observation group demonstrated superior outcomes, with 1- and 2-year PFS rates of 77.3% vs. 46.7% and 46.0% vs. 10.4%, respectively. Due to the interaction and non-proportionality of the Kaplan-Meier curves between groups, a segmented RMST analysis was conducted.
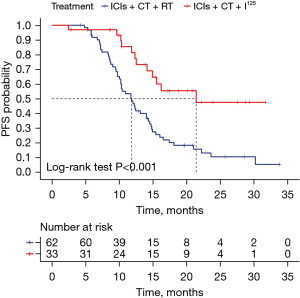
At the point of intersection (5.3 months), the RMST was 5.2 (95% CI: 5.043–5.382) months for the observation group and 5.3 (95% CI: 5.276–5.308) months for the control group, showing no significant difference (P=0.36). At 31.7 months (the shortest maximum observation period among the groups), the RMSTs were 22.2 (95% CI: 18.257–26.101) months for the observation group and 13.8 (95% CI: 11.912–15.718) months for the control group, indicating significantly better PFS in the observation group (P<0.001), as depicted in Figures 2,3.
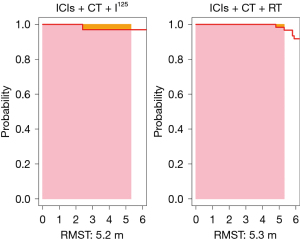
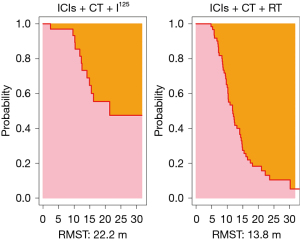
Adverse events
Pneumothorax and hemorrhage were the major complications associated with I125 seed implantation in the observation group. Of these, 14 patients experienced pneumothorax and had a closed chest drain placed; 13 (39.4%) experienced grade 1–2 adverse events (complete lung reexpansion and removal of drain before discharging from the hospital); and one (3.0%) experienced grade 3 adverse events (discharged with a drain). All of the patients experienced hemorrhage; 32 (97.0%) experienced grade 1–2 adverse events (bleeding up to 50 mL in four cases and less than 20 mL in the remaining cases), and 1 (3.0%) experienced grade 3 adverse events (hemopneumothorax with 100 mL of bleeding). Radiation pneumonitis, radiation esophagitis, and other radiation-related adverse events were observed in the control group. Of these, 17 cases (27.4%) were grade 1–2 adverse events and 16 cases (25.8%) were grade 3 adverse events (receiving hormonal or other treatments). The control group saw a greater prevalence of grade 3 adverse events (Chi-squared test P=0.02).
Furthermore, six patients in the observation group (18.2%) suffered immunotherapy-related diseases (such as immune-associated pneumonia), whereas five patients in the control group (8.1%) had immunotherapy-related diseases, all of which were in grade 1–2. In the two groups, there were 16 (48.5%) and 29 (46.8%) occurrences of myelosuppression, all of which were grade 1–2. Nine (14.5%) extra adverse events, all grade 1–2, happened in the control group while none in the observation group (Table 2).
Table 2
Adverse event | Grade | Observation group (n=33) | Control group (n=62) |
---|---|---|---|
Pneumothorax | 1–2 | 13 (39.4) | – |
3 | 1 (3.0) | – | |
Hemorrhage | 1–2 | 32 (97.0) | – |
3 | 1 (3.0) | – | |
Radiation pneumonitis/esophagitis, etc. | 1–2 | – | 17 (27.4) |
3 | – | 16 (25.8) | |
Immune-associated pneumonia (other diseases) | 1–2 | 6 (18.2) | 5 (8.1) |
3 | – | 0 | |
Myelosuppression | 1–2 | 16 (48.5) | 29 (46.8) |
3 | 0 | 0 | |
Others | 1–2 | 0 | 9 (14.5) |
3 | 0 | 0 |
Data are presented as n (%).
Analysis of subgroups
Subgroup analyses were conducted on seven parameters: tumor size (T-stage), lymph node metastasis (N-stage), distant metastasis (M-stage), pathological type, smoking index, underlying pulmonary disease, and the use of anti-angiogenesis therapy. Analysis of subgroups with distant metastasis, squamous carcinoma, smoking index over 400 pack-years, and those not receiving anti-angiogenesis therapy, compared to their respective controls, identified I125 seed implantation as an independent prognostic factor for improved PFS. This suggests that I125 seed implantation may have a better therapeutic outcome for the aforementioned groups (Figure 4).
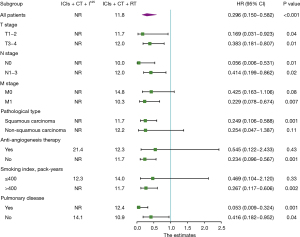
In the observation group, 23 patients were treated solely with I125 seed implantation. Of these, 16 received seed implantation during the maintenance phase (after 4–6 cycles of CT plus immunotherapy), and seven during the initial phase (before starting CT plus immunotherapy), with respective median PFS of not reached and 15.5 (95% CI: 11.373–19.627) months. Although survival curves suggested a trend towards improved outcomes for maintenance phase implantation, statistical significance was not achieved (log-rank test P=0.17; Cox model P=0.18, HR =0.387), as shown in Figure 5. Notably, patients with maintenance phase implantation exhibited higher 1- and 2-year PFS rates compared to those with initial phase implantation.
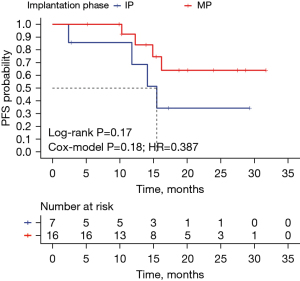
Discussion
For patients with driver gene-negative NSCLC, traditional platinum-based CT and RT show limited effectiveness. The advent of ICIs targeting programmed death 1 (PD-1) and PD-L1 marks a significant advancement, offering substantial survival benefits. Research, including the PACIFIC and NCT02621398 studies, demonstrates that ICIs, followed by concurrent radio-CT, notably enhance survival in stage III unresectable NSCLC (4,5). Prof. Chang et al.’s study (7) further suggests that extending such treatment to early-stage (I and II) unresectable lung cancer with RT plus immunotherapy (I-SABR) considerably improves 4-year event-free survival rates compared to RT alone.
To the best of our knowledge, this research represents the first cohort study exploring the effectiveness and safety of ICIs combined with CT and I125 seed implantation regimens. In our study, the median PFS in the observation group and control group were not reached and 11.8 months, respectively. As the assumption of proportional hazards was not met, we employed the RMST and the area under the Kaplan-Meier curve as alternative analytical approaches. The survival curves of both groups intersected at 5.3 months, a phenomenon potentially attributable to the limited sample size. This small cohort may have introduced variability into the OS analysis, with the outcomes significantly influenced by individual data points. At this time, the RMST for the observation group was 5.2 (95% CI: 5.043–5.382) months, compared to 5.3 months for the control group (95% CI: 5.276–5.308), a difference that was not statistically significant (P=0.36). Notably, at the 31.7-month minimum follow-up, the RMSTs diverged significantly between groups. The observation group demonstrated a RMST of 22.2 (95% CI: 18.257–26.101) months, markedly outperforming the control group’s 13.8 (95% CI: 11.912–15.718) months. This superior performance was also reflected in the PFS rates, with the observation group achieving 1- and 2-year PFS rates of 77.3% versus 46.7%, and 46.0% versus 10.4%, respectively. By the follow-up cut-off point, a significant discrepancy in the rate of endpoint events (disease progression) was observed: 52 cases (83.9%) within the control group had progressed, in stark contrast to 13 cases (39.4%) in the observation group. This suggests that the advantages seen in the observation group could become even more pronounced over time.
Radiation pneumonitis predominantly occurs within 2 to 6 months following radiation therapy (8). Key clinical risk factors that heighten the risk for this condition include advanced age, male gender, the location of the tumor, compromised lung function, and the presence of other health conditions (16-19). Moreover, employing ICIs in combination further escalates this risk (20-22). Various studies indicate that 15–40% of patients undergoing chest radiation therapy develop radiation pneumonitis, with an additional 10–20% experiencing severe forms of the illness. Alarmingly, the mortality rate among these patients can reach up to 50% (23). In our study, we observed a notably high incidence rate of radiation pneumonitis/esophagitis in the control group, reaching 53.2% (33 cases), attributed largely to the augmented risk introduced by the use of ICIs. Of these cases, 16 were classified as grade 3 adverse events, necessitating intervention with hormone therapy or alternative treatments. Additionally, it is well-documented that patients with existing pulmonary conditions, especially those with interstitial lung disease (ILD) or chronic obstructive pulmonary disease (COPD), are at an increased risk of developing radiation pneumonitis (24-28). In our control group, there were 22 cases of underlying pulmonary diseases (19 of COPD/emphysema, one of ILD, one of secondary tuberculosis, and one of pneumoconiosis), leading to 13 instances of radiation pneumonitis as a result of the therapy. Research by Shi et al. (29) suggests that patients with NSCLC and normal lung function generally manage the side effects of radiation therapy well. However, those patients with COPD experience a significant reduction in lung function after therapy. Despite these challenges, radiation therapy remains a critical prognostic factor for NSCLC patients with severe pulmonary impairment (30), underscoring the need for tailored treatment strategies for this patient population.
Based on our research, external beam radiation therapy presents a lower safety profile compared to I125 seed implantation. Advances in the TPS for radioactive seed therapy have led to more precise delineation of the target area and three-dimensional (3D) metrology planning. These improvements ensure the tumor receives an adequate dose while minimizing exposure to nearby critical organs and tissues. Pneumothorax and hemorrhage emerged as the predominant complications after I125 seed implantation. In our observation group of 33 patients, 14 (42.4%) experienced pneumothorax and received closed chest drainage treatment. Of these, 13 patients had their drainage tubes removed before discharge, while one patient was discharged with the tube still in place, which was removed after one week. Post-treatment, all patients exhibited full lung re-expansion without any permanent consequences. Bleeding occurred in every patient during the implantation process, with one instance of 100 mL, four instances of 50 mL, and the rest limited to 20 mL. These hemorrhages were effectively managed through symptomatic treatments and medications that facilitated absorption, leading to complete resolution.
For patients receiving combined regimens of RT and ICIs, RT and immunotherapy are typically performed sequentially in order to avoid the superimposed risk of radiation pneumonitis and immune-associated pneumonitis. Local seed implantation not only gets around this restriction but also amplifies the synergistic effect of RT and immunotherapy. After implantation in the body, the I125 particles will produce circular or cylindrical localized radiation, and this sustained killing effect on tumor cells can be maintained for several months (31). In the meantime, the radioactive seeds keep improving the tumor’s immune milieu, which makes it easier for ICIs to have a therapeutic impact. Additionally, our findings demonstrated that while the incidence of immune-related pneumonitis was slightly higher (18.2%) in the group receiving I125 seed implantation, all of the adverse events were grade 1–2 and did not differ statistically (P=0.26) from the group receiving conventional RT (8.1%). There was no discernible increase in the risk of immune-related pneumonia from I125 seed implantation while radiation pneumonitis was avoided.
Besides, our study also indicated that timing I125 seed implantation at mid-treatment (maintenance phase after CT combined with immunotherapy) appeared to have a better outcome compared to the initial phase of treatment, with PFS not reached and 15.5 months, respectively. In terms of the trend in the survival curves and the comparison of the PFS rates at 1 and 2 years, the maintenance phase implantation examples outperformed the treatment-initial phase, even though the difference was not statistically significant (log-rank test P=0.17; cox-model P=0.18, HR =0.387). Chemotherapeutic drugs can exert anti-tumor effects early in the course of treatment and gradually display a trend of drug resistance over time. In contrast to ICIs, which typically detect anti-tumor efficacy 2–4 months after treatment due to delayed response (31), and I125 seeds, which have a half-life of 59.43 days and are effective in vivo for up to 4 months. Implantation of seeds in the middle of treatment provides a longer period of radio-immuno-coordination compared to the beginning of treatment. Additionally, since this region of the patient responds better to immunotherapy, the implant’s maintenance phase is more effective, because patients will select second-line therapies to skip the maintenance period if they do not react to conventional treatment. Thus, it is crucial to forecast and screen the population undergoing immunotherapy using PD-L1, tumor mutation burden (TMB), and other indications. Implanting seeds during the maintenance phase is advised for patients who respond effectively to immunotherapy. Initial use of combination therapies (such as I125 seeds implantation) can help patients with poor immune responses not only limit the spread of the tumor but also potentially enhance the tumor’s immunological environment, improving treatment outcomes. To have the optimum treatment outcome, this patient group might require more than two seeds implantations (the initial phase of therapy + treatment maintenance stage).
The following are the limitations of our study: due to the retrospective nature of the study and its single-center design, bias in sample selection may have occurred; results may have fluctuated more due to smaller sample sizes and the impact of individual samples; I125 seed implantation required patients to have a better lung condition or general condition than RT, and even though there was no significant difference in the incidence of pulmonary diseases between the observation and control groups, there may still be some potential difference; TMB and PD-L1 expression are linked to the effectiveness of immunotherapy; however, most study participants were not tested for those expressions due to financial or other reasons, and the therapeutic effects of various ICIs and chemotherapeutic agents may differ. Based on preliminary research findings, we are currently establishing a higher-quality prospective cohort study to reduce bias and further enhance the reliability of the results.
Conclusions
I125 seed implantation combined with CT and ICIs improves short-term survival and causes fewer side effects in patients with driver gene-negative NSCLC who are not eligible for surgery, compared to RT combined with CT and immunotherapy. It is especially advised for those with significant radiation pneumonia risk factors to receive I125 seed implantation.
Acknowledgments
Funding: This study was supported by
Footnote
Reporting Checklist: The authors have completed the STROBE reporting checklist. Available at https://jtd.amegroups.com/article/view/10.21037/jtd-24-1403/rc
Data Sharing Statement: Available at https://jtd.amegroups.com/article/view/10.21037/jtd-24-1403/dss
Peer Review File: Available at https://jtd.amegroups.com/article/view/10.21037/jtd-24-1403/prf
Conflicts of Interest: All authors have completed the ICMJE uniform disclosure form (available at https://jtd.amegroups.com/article/view/10.21037/jtd-24-1403/coif). The authors have no conflicts of interest to declare.
Ethical Statement: The authors are accountable for all aspects of the work in ensuring that questions related to the accuracy or integrity of any part of the work are appropriately investigated and resolved. The study was conducted in accordance with the Declaration of Helsinki (as revised in 2013). The study was approved by the Ethics Committee of the First Affiliated Hospital of Army Medical University (No. KY2024028). Medical records obtained in previous clinical treatment were collected and analyzed retrospectively, individual consent for this study was waived.
Open Access Statement: This is an Open Access article distributed in accordance with the Creative Commons Attribution-NonCommercial-NoDerivs 4.0 International License (CC BY-NC-ND 4.0), which permits the non-commercial replication and distribution of the article with the strict proviso that no changes or edits are made and the original work is properly cited (including links to both the formal publication through the relevant DOI and the license). See: https://creativecommons.org/licenses/by-nc-nd/4.0/.
References
- Oncology Society of Chinese Medical Association, Chinese Medical Association Publishing House. Chinese Medical Association guideline for clinical diagnosis and treatment of lung cancer (2024 edition). Chinese Journal of Oncology 2024;46:805-43. [PubMed]
- Vanpouille-Box C, Alard A, Aryankalayil MJ, et al. DNA exonuclease Trex1 regulates radiotherapy-induced tumour immunogenicity. Nat Commun 2017;8:15618. [Crossref] [PubMed]
- Ashrafizadeh M, Farhood B, Eleojo Musa A, et al. Abscopal effect in radioimmunotherapy. Int Immunopharmacol 2020;85:106663. [Crossref] [PubMed]
- Antonia SJ, Villegas A, Daniel D, et al. Durvalumab after Chemoradiotherapy in Stage III Non-Small-Cell Lung Cancer. N Engl J Med 2017;377:1919-29. [Crossref] [PubMed]
- Jabbour SK, Berman AT, Decker RH, et al. Phase 1 Trial of Pembrolizumab Administered Concurrently With Chemoradiotherapy for Locally Advanced Non-Small Cell Lung Cancer: A Nonrandomized Controlled Trial. JAMA Oncol 2020;6:848-55. [Crossref] [PubMed]
- Xu TZ, Liang J. Research progress on radiotherapy combined with immunotherapy for non-small cell lung cancer. Chinese Journal of Radiation Oncology 2022;31:579-84.
- Chang JY, Lin SH, Dong W, et al. Stereotactic ablative radiotherapy with or without immunotherapy for early-stage or isolated lung parenchymal recurrent node-negative non-small-cell lung cancer: an open-label, randomised, phase 2 trial. Lancet 2023;402:871-81. Erratum in: Lancet 2023;402:850. [Crossref] [PubMed]
- Hanania AN, Mainwaring W, Ghebre YT, et al. Radiation-Induced Lung Injury: Assessment and Management. Chest 2019;156:150-62. [Crossref] [PubMed]
- Li W, Zheng Y, Li Y, et al. Effectiveness of (125)I seed implantation in the treatment of non-small cell lung cancer during R2 resection. Oncol Lett 2017;14:6690-700. [PubMed]
- Huo X, Huo B, Wang H, et al. Implantation of computed tomography-guided Iodine-125 seeds in combination with chemotherapy for the treatment of stage III non-small cell lung cancer. J Contemp Brachytherapy 2017;9:527-34. [Crossref] [PubMed]
- Wang B, Wang J, Zhang XK, et al. Short-term efficacy evaluation of radio I-125 implantation combined with systemic chemotherapy in the treatment of stage III central lung squamous cell carcinoma. Shandong Medical Journal 2020;54-6.
- Li W, Guan J, Yang L, et al. Iodine-125 brachytherapy improved overall survival of patients with inoperable stage III/IV non-small cell lung cancer versus the conventional radiotherapy. Med Oncol 2015;32:395. [Crossref] [PubMed]
- Yue TH, Xing W. (125)I Seed Brachytherapy Combined with Single-Agent Chemotherapy in the Treatment of Non-Small-Cell Lung Cancer in the Elderly: A Valuable Solution. Onco Targets Ther 2020;13:10581-91. [Crossref] [PubMed]
- Li H, Duan Z, Zhao C, et al. Combination of Brachytherapy with Iodine-125 Seeds and Systemic Chemotherapy versus Systemic Chemotherapy Alone for Synchronous Extracranial Oligometastatic Non-Small Cell Lung Cancer. Cancer Manag Res 2020;12:8209-20. [PubMed]
- Zhang F, Wang J, Guo J, et al. Chinese expert consensus workshop report: Guideline for permanent iodine-125 seeds implantation of primary and metastatic lung tumors (2020 edition). J Cancer Res Ther 2020;16:1549-54. [Crossref] [PubMed]
- Chen Z, Yi G, Li X, et al. Predicting radiation pneumonitis in lung cancer using machine learning and multimodal features: a systematic review and meta-analysis of diagnostic accuracy. BMC Cancer 2024;24:1355. [Crossref] [PubMed]
- Gunnarsson K, Mövik L, Pettersson N, et al. Assessment of radiation pneumonitis and predictive factors in patients with locally advanced non-small cell lung cancer treated with chemoradiotherapy. Acta Oncol 2024;63:791-7. [Crossref] [PubMed]
- Yan Y, Zhu Y, Yang S, et al. Clinical predictors of severe radiation pneumonitis in patients undergoing thoracic radiotherapy for lung cancer. Transl Lung Cancer Res 2024;13:1069-83. [Crossref] [PubMed]
- Tang C, Mistry H, Bayman N, et al. Outcomes of curative-intent radiotherapy in non-small cell lung cancer (NSCLC) patients with chronic obstructive pulmonary disease (COPD) and interstitial lung disease (ILD). Radiother Oncol 2021;160:78-81. [Crossref] [PubMed]
- Shaverdian N, Lisberg AE, Bornazyan K, et al. Previous radiotherapy and the clinical activity and toxicity of pembrolizumab in the treatment of non-small-cell lung cancer: a secondary analysis of the KEYNOTE-001 phase 1 trial. Lancet Oncol 2017;18:895-903. Erratum in: Lancet Oncol 2017;18:e371. [Crossref] [PubMed]
- Li M, Gan L, Song A, et al. Rethinking pulmonary toxicity in advanced non-small cell lung cancer in the era of combining anti-PD-1/PD-L1 therapy with thoracic radiotherapy. Biochim Biophys Acta Rev Cancer 2019;1871:323-30. [Crossref] [PubMed]
- Geng Y, Zhang Q, Feng S, et al. Safety and Efficacy of PD-1/PD-L1 inhibitors combined with radiotherapy in patients with non-small-cell lung cancer: a systematic review and meta-analysis. Cancer Med 2021;10:1222-39. [Crossref] [PubMed]
- Stahl JM, Corso CD, Verma V, et al. Trends in stereotactic body radiation therapy for stage I small cell lung cancer. Lung Cancer 2017;103:11-6. [Crossref] [PubMed]
- Yoneyama M, Matsuo Y, Kishi N, et al. Quantitative analysis of interstitial lung abnormalities on computed tomography to predict symptomatic radiation pneumonitis after lung stereotactic body radiotherapy. Radiother Oncol 2024;198:110408. [Crossref] [PubMed]
- Glick D, Lyen S, Kandel S, et al. Impact of Pretreatment Interstitial Lung Disease on Radiation Pneumonitis and Survival in Patients Treated With Lung Stereotactic Body Radiation Therapy (SBRT). Clin Lung Cancer 2018;19:e219-26. [Crossref] [PubMed]
- Saha A, Dickinson P, Shrimali RK, et al. Is Thoracic Radiotherapy an Absolute Contraindication for Treatment of Lung Cancer Patients With Interstitial Lung Disease? A Systematic Review. Clin Oncol (R Coll Radiol) 2022;34:e493-504. [Crossref] [PubMed]
- Hwang J, Kim H, Kim SM, et al. Preliminary Results of Developing Imaging Complexity Biomarkers for the Incidence of Severe Radiation Pneumonitis Following Radiotherapy in Non-Small Cell Lung Cancer Patients with Underlying Idiopathic Pulmonary Fibrosis. Life (Basel) 2024;14:897. [Crossref] [PubMed]
- Chen H, Senan S, Nossent EJ, et al. Treatment-Related Toxicity in Patients With Early-Stage Non-Small Cell Lung Cancer and Coexisting Interstitial Lung Disease: A Systematic Review. Int J Radiat Oncol Biol Phys 2017;98:622-31. [Crossref] [PubMed]
- Shi LL, Yang JH, Yao HF. Multiple regression analysis of risk factors related to radiation pneumonitis. World J Clin Cases 2023;11:1040-8. [Crossref] [PubMed]
- Deng Q, Zhang Y, Li Y, et al. Survival of Patients With Inoperable Non-Small Cell Lung Cancer With Baseline Severe Pulmonary Dysfunction: Impacts of Thoracic Radiotherapy and Predictive Analysis for Acute Radiation Pneumonitis. Oncology (Williston Park) 2023;37:26-33. [PubMed]
- Zhang Q, Tao XL, Wu N. Research progress of 18F-FDG PET/CT in evaluating the efficacy and prognosis of immunotherapy for non-small cell lung cancer. China Cancer 2024;33:247-53.