Neutrophil-to-lymphocyte ratio and its correlation with tuberculosis infection: a cross-sectional survey based on the NHANES database
Highlight box
Key findings
• Our study reveals a significant negative correlation between neutrophil-to-lymphocyte ratio (NLR) and the risk of tuberculosis infection (TBI), particularly in males and individuals with hypertension.
What is known and what is new?
• Inflammatory responses play a crucial role in the pathogenesis of TBI, but the association between the emerging inflammatory marker NLR and the risk of adult TBI remains unclear.
• By examining data from the National Health and Nutrition Examination Survey, our study is the first to explore the association between NLR and the risk of adult TBI, finding variations in the association across different populations.
What is the implication, and what should change now?
• The results suggest that a moderate level of systemic inflammation, as indicated by NLR, may aid in resistance to pathogens. Monitoring NLR could help identify high-risk individuals early, especially in resource-limited settings where the accessibility of NLR makes it a potential tool for screening TBI risk.
• Further validation in diverse populations is needed to assess the universality and applicability of these findings within different health systems. NLR could serve as a biomarker for assessing TBI risk, providing valuable insights for future clinical applications and public health strategies.
Introduction
Tuberculosis (TB) is an infectious disease triggered by Mycobacterium tuberculosis (Mtb) and is also one of the leading culprits of death from a single infectious agent (1). According to the data from the World Health Organization (WHO), around 10.6 million people worldwide were estimated to have TB in 2022, with 1.3 million TB deaths (2). TB has become one of the primary causes of infectious disease deaths globally, placing a serious threat to human health. Tuberculosis infection (TBI) is a state of sustained immune response to antigens of Mtb without clinical evidence of active TB, serving as a precursor event for Mtb transmission (3,4). It is estimated that about a quarter of the global population is infected with Mtb (5), with the majority of cases being controlled by the immune system and remaining asymptomatic. Although only 5–10% of TBI progress to active TB with a lifelong risk (6), reactivation of latent TBI accounts for over 80% of active TB cases (7). In light of these data, in 2016, the WHO recommended effective diagnosis and more targeted management of TBI patients as a key component of the End TB Strategy (8). Unfortunately, these strategies have not yet been proven to be sufficiently effective, leaving the global elimination of TB a daunting challenge. Since TB is not a simple infectious disease but an infectious disease influenced by various risk factors (9), understanding the risk factors related to TBI will aid in formulating important healthcare measures.
It is well known that the inflammatory response is a major manifestation of the pathophysiology of TBI. During TBI, the immune system can promote and limit the spread of pathogens by manipulating the inflammatory response, causing harm to the host (10,11). As a commonly utilized clinical marker of inflammatory response, the expression of neutrophil-to-lymphocyte ratio (NLR) has been proven to be linked with the TBI status. A project on children demonstrated obvious differences in NLR between active TB and healthy groups (12). Its accuracy as a diagnostic biomarker as well as its function in monitoring anti-TB treatment has been confirmed in a previous observational study (13). Nevertheless, whether NLR is a reliable predictive factor related to changes in TBI risk has not been determined. Currently, only one project focusing on children investigated the relationship between NLR and TBI (14). However, it did not mention the correlation between NLR and changes in TBI death risk (14).
Therefore, we utilized data from the National Health and Nutrition Examination Survey (NHANES) database to dig out the association between NLR and TBI risk, attempting to analyze potential influencing factors of this association and examine the relationship between NLR and survival risk in TBI patients. We present this article in accordance with the TRIPOD reporting checklist (available at https://jtd.amegroups.com/article/view/10.21037/jtd-24-750/rc).
Methods
Data source and study population
The NHANES is a survey that collects demographic information on the health and nutritional intakes of U.S. citizens, supervised and administered by the National Center for Health Statistics (NCHS) (15). Each year, the NHANES visits 15 counties nationwide and examines a nationally representative sample of approximately 5,000 people. The uniqueness of this database lies in the combination of interviews and physical examinations. The interviews include data on demographics, diet, socioeconomics, and health, assessing the health and nutritional status of the U.S. residents. Participants provided written informed consent at the time of the survey. All NHANES data have been de-identified and all NHANES studies have been approved by the National Center for Health Statistics Research Ethics Review Board (16). The study was conducted in accordance with the Declaration of Helsinki (as revised in 2013).
This project selected information from 19,721 participants in two consecutive cycles of 1999–2000 and 2011–2012 from the database with comprehensive TBI-related questionnaire data as well as other necessary covariate information. To ensure the integrity of the sample information in this project, 13,246 cases with missing data on lymphocytes and neutrophils, as well as participants with missing results of Tuberculin Skin Test (TST) or QuantiFERON-TB Gold In-Tube Test (QFT-GIT) examinations, were excluded. Additionally, 4,042 cases with missing data on covariates [gender, age, race, body mass index (BMI), poverty-income ratio (PIR), smoking, alcohol consumption, hypertension, diabetes, history of TB, contact with active TB patients, and human immunodeficiency virus (HIV)] were excluded. Finally, 2,433 eligible participants were included, including 391 TBI patients and 2,042 non-TBI patients. The detailed screening process of participants is presented in Figure 1.
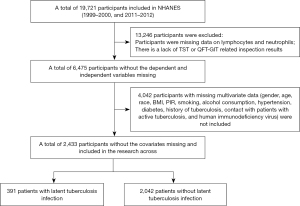
NLR
The morning fasting venous blood samples were collected from all participants for routine clinical chemistry analysis. The Coulter counter method was employed to obtain lymphocyte and neutrophil counts of 1,000 cells/µL from whole blood. The NLR was calculated using the formula: NLR = neutrophil count/lymphocyte count (17).
TBI
TBI was defined as participants who tested positive for TST and/or QFT-GIT (18). If only one TST result was recorded, it was utilized. Otherwise, the average was utilized. A positive TST was defined as an induration reading of greater than or equal to 10 millimeters. Participants with a positive QFT-GIT result were sorted as TBI positive, while those with a negative QFT-GIT result were sorted as TBI negative (19).
Variables
Variables including age at diagnosis, gender (male and female), race (categorized as Mexican descent, other Hispanic descent, non-Hispanic white, non-Hispanic black, and other race), BMI (normal: <25 kg/m2, overweight: 25 to <30 kg/m2, obese: ≥30 kg/m2), alcohol consumption (at least 12 drinks per year), and smoking status (current smoker, former smoker, never smoked) were analyzed. PIR was the ratio of family income to the poverty line, which was applied to define income.
Diabetes was defined as self-reported information on insulin and oral hypoglycemic agent use, or glycated hemoglobin A1c level >6.5% and fasting blood glucose >126 mg/dL (20). Hypertension (21) was defined as meeting one of the following criteria: (I) previously diagnosed with hypertension; (II) self-reported use of antihypertensive medication; (III) in the NHANES examination component, average systolic blood pressure greater than or equal to 130 mmHg or diastolic blood pressure greater than or equal to 80 mmHg (22). History of TB was defined as participants who said “yes” to the following question: were you ever told that you had active TB or TB? A history of contact with an active TB patient was defined as participants who responded “yes” to the following question: have you ever lived in the same household with someone while that person was sick with active TB or TB (18)? HIV status was determined by HIV-1 antibody blood test results [HIV+: individuals infected with the acquired immunodeficiency syndrome (AIDS) virus; HIV–: individuals not infected with the AIDS virus] (23).
Statistical analysis
We employed the “tableone” package to generate baseline tables. Based on the general population characteristics and whether they had TBI, we grouped the respondents and drew baseline tables. Categorical variables were represented by n (%), while continuous variables were represented by mean [standard deviation (SD)] (n: sample size without weight adjustment; n (%): proportion with weight adjustment; mean: mean value with weight adjustment; SD: standard deviation with weight adjustment). The “survey” package was utilized to construct a weighted logistic regression model of NLR and TBI. The stratified analysis was carried out on the categorical variables in the model without adjusting for confounding factors. A Chi-squared ratio test was applied to the interaction term P values of the stratified logistics regression model with all confounders adjusted. P<0.05 indicated a notable difference. We stratified the NLR, set up a weighted logistic regression model of NLR and TBI with confounding factors adjusted, and carried out the subgroup analysis on confounding factors with interaction term P value <0.1. In the weighted logistic regression model, the association between NLR and TBI was dissected by using restricted cubic spline (RCS), and the relationship between NLR and TBI survival rates was investigated by utilizing Kaplan-Meier (K-M) curves. Based on these analyses, we determined the critical value of NLR for distinguishing TBI patients with different risk levels. In this investigation, the models included the Crude model without adjustment; model I with gender, age, race, BMI, PIR, smoking, and alcohol consumption adjusted; model II with gender, age, race, BMI, PIR, smoking, alcohol consumption, hypertension, diabetes, history of TB, history of contact with active TB patients, and HIV status adjusted. All statistical analyses were carried out by using R (V4.2.2) software.
Results
Baseline characteristics of respondents
This investigation included a total of 2,433 relevant patients from the NHANES database between 1999–2000 and 2011–2012. Table 1 displays their characteristics. Among all participants, individuals with non-TBI and TBI exhibited great differences in gender, race, PIR, smoking status, alcohol consumption, history of contact with active TB patients, history of TB, lymphocyte count, and NLR (all P<0.05). From the table, TBI patients were observed to be more likely to be male (60.2%) with a history of smoking (52.2%) and alcohol consumption (76.0%), lower PIR (2.18±1.58 vs. 2.88±1.69), but without a history of contact with active TB patients (91.3%) or TB history (96.5%). TBI patients had higher lymphocyte counts (2.22±0.63 vs. 2.07±0.66, P=0.01) but lower NLR (1.99±0.83 vs. 2.19±1.04, P=0.001).
Table 1
Characteristics | Total | Non-TBI | TBI | P value |
---|---|---|---|---|
Overall | 2,433 | 2,042 (89.6) | 391 (10.4) | |
Gender | 0.002 | |||
Female | 1,189 (49.6) | 1,025 (50.7) | 164 (39.8) | |
Male | 1,244 (50.4) | 1,017 (49.3) | 227 (60.2) | |
Age (years) | 39.69 (11.70) | 39.62 (11.79) | 40.31 (10.96) | 0.59 |
Race | <0.001 | |||
Mexican American | 300 (9.1) | 202 (7.7) | 98 (21.3) | |
Other Hispanic | 232 (7.5) | 177 (6.1) | 55 (18.9) | |
Non-Hispanic White | 907 (64.6) | 867 (69.3) | 40 (24.3) | |
Non-Hispanic Black | 612 (11.7) | 527 (11.1) | 85 (16.5) | |
Other race | 382 (7.1) | 269 (5.7) | 113 (18.9) | |
PIR | 2.80 (1.69) | 2.88 (1.69) | 2.18 (1.58) | <0.001 |
BMI (kg/m2) | 28.96 (6.68) | 29.04 (6.67) | 28.27 (6.71) | 0.13 |
Smoking | 0.03 | |||
Never smoking | 1,426 (57.2) | 1,212 (58.3) | 214 (47.8) | |
Former smoking | 422 (20.6) | 350 (20.5) | 72 (21.3) | |
Now smoking | 585 (22.2) | 480 (21.2) | 105 (30.9) | |
Alcohol drinking | 0.003 | |||
No | 538 (15.8) | 417 (14.9) | 121 (24.0) | |
Yes | 1,895 (84.2) | 1,625 (85.1) | 270 (76.0) | |
Hypertension | 0.56 | |||
No | 1,406 (58.4) | 1,184 (58.7) | 222 (56.2) | |
Yes | 1,027 (41.6) | 858 (41.3) | 169 (43.8) | |
Diabetes | 0.05 | |||
No | 2,187 (92.0) | 1,851 (92.4) | 336 (88.7) | |
Yes | 246 (8.0) | 191 (7.6) | 55 (11.3) | |
HIV | 0.29 | |||
HIV− | 2,421 (99.6) | 2,031 (99.6) | 390 (99.9) | |
HIV+ | 12 (0.4) | 11 (0.4) | 1 (0.1) | |
Ever lived with someone with active TB | <0.001 | |||
No | 2,357 (97.5) | 1,996 (98.2) | 361 (91.3) | |
Yes | 76 (2.5) | 46 (1.8) | 30 (8.7) | |
History of TB | <0.001 | |||
No | 2,418 (99.6) | 2,041 (100.0) | 377 (96.5) | |
Yes | 15 (0.4) | 1 (0.0) | 14 (3.5) | |
All-cause mortality | >0.99 | |||
No | 2,349 (97.2) | 1,974 (97.2) | 375 (97.1) | |
Yes | 84 (2.8) | 68 (2.8) | 16 (2.9) | |
Cardiovascular mortality | 0.44 | |||
No | 2,414 (99.6) | 2,025 (99.5) | 389 (99.8) | |
Yes | 19 (0.4) | 17 (0.5) | 2 (0.2) | |
Lymphocyte count (1,000 cell/μL) | 2.08 (0.66) | 2.07 (0.66) | 2.22 (0.63) | 0.01 |
Neutrophil number (1,000 cell/μL) | 4.23 (1.66) | 4.23 (1.66) | 4.21 (1.61) | 0.82 |
NLR | 2.17 (1.02) | 2.19 (1.04) | 1.99 (0.83) | 0.001 |
Categorical variables were represented by n (%), while continuous variables were represented by mean (standard deviation). NHANES, National Health and Nutrition Examination Survey; TBI, tuberculosis infection; PIR, poverty-income ratio; BMI, body mass index; HIV, human immunodeficiency virus; TB, tuberculosis; NLR, neutrophil-lymphocyte ratio.
Stratified analysis
We employed weighted logistic regression to set up a relationship model between NLR and TBI. Stratified analysis (Table 2) unveiled that in populations of males, never smokers but with a history of alcohol consumption, with hypertension, HIV-negative, with no history of contact with active TB patients, and with no history of TB, the risk of TBI was negatively correlated with NLR [odds ratio (OR) <1, P<0.05]. Subsequently, after adjusting for all confounding factors for interaction testing, the results demonstrated that diabetes remarkably influenced the relationship between the two (P for interaction =0.049). Patients with diabetes had a 42% lower risk of TBI [OR: 0.58, 95% confidence interval (CI): 0.38–0.90, P=0.009].
Table 2
Participants | OR | 95% CI | P value | P for interaction |
---|---|---|---|---|
Gender | 0.20 | |||
Female | 0.87 | 0.70–1.07 | 0.20 | |
Male | 0.73 | 0.57–0.95 | 0.01 | |
Race | 0.80 | |||
Mexican American | 0.83 | 0.66–1.04 | 0.08 | |
Other Hispanic | 0.83 | 0.54–1.27 | 0.30 | |
Non-Hispanic White | 0.75 | 0.49–1.17 | 0.20 | |
Non-Hispanic Black | 0.90 | 0.66–1.25 | 0.50 | |
Other race | 0.99 | 0.73–1.35 | >0.99 | |
Smoking | 0.33 | |||
Never smoking | 0.65 | 0.50–0.83 | <0.001 | |
Former smoking | 0.89 | 0.60–1.31 | 0.50 | |
Now smoking | 0.91 | 0.70–1.18 | 0.50 | |
Alcohol drinking | 0.51 | |||
No | 0.74 | 0.50–1.11 | 0.12 | |
Yes | 0.79 | 0.67–0.94 | 0.004 | |
Hypertension | 0.09 | |||
No | 0.87 | 0.72–1.05 | 0.12 | |
Yes | 0.68 | 0.54–0.87 | <0.001 | |
Diabetes | 0.049 | |||
No | 0.81 | 0.68–0.96 | 0.01 | |
Yes | 0.58 | 0.38–0.90 | 0.009 | |
HIV | 0.13 | |||
HIV− | 0.79 | 0.67–0.92 | 0.002 | |
HIV+ | – | – | – | |
Ever lived with someone with active TB | 0.40 | |||
No | 0.77 | 0.66–0.91 | 0.002 | |
Yes | 1.17 | 0.58–2.39 | 0.60 | |
History of TB | 0.33 | |||
No | 0.79 | 0.68–0.93 | 0.003 | |
Yes | 2.05 | 0.18–23.0 | 0.20 |
P for interaction indicated adjusting for gender, age, race, BMI, PIR, smoking, alcohol consumption, hypertension, diabetes, history of TB, history of contact with active TB patients, and HIV status. NLR, neutrophil-lymphocyte ratio; TBI, tuberculosis infection; OR, odds ratio; CI, confidence interval; HIV, human immunodeficiency virus; TB, tuberculosis; BMI, body mass index; PIR, poverty-income ratio.
Subgroup analysis
As shown in Table 3, the constructed Crude model (OR: 0.79, 95% CI: 0.67–0.92, P=0.002), model I (OR: 0.83, 95% CI: 0.72–0.97, P=0.02), and model II (OR: 0.84, 95% CI: 0.72–0.98, P=0.03) all indicated a notable negative linkage between NLR and TBI occurrence. To figure out the optimal critical value of NLR associated with adverse outcomes, we applied the Youden index and further stratified NLR using the receiver operating characteristic (ROC) curves. The optimal cutoff value for NLR was determined to be 1.766 (Figure S1). NLR was then divided into two groups (NLR ≤1.766 and NLR >1.766). The results manifested that compared to NLR ≤1.766, NLR >1.766 remarkably reduced TBI risk (OR <1, P=0.03).
Table 3
Participants | Crude | Model I | Model II | |||||
---|---|---|---|---|---|---|---|---|
OR (95% CI) | P value | OR (95% CI) | P value | OR (95% CI) | P value | |||
All participants | 0.79 (0.67–0.92) | 0.002 | 0.83 (0.72–0.97) | 0.02 | 0.84 (0.72–0.98) | 0.03 | ||
NLR | ||||||||
≤1.766 | Ref. | Ref. | Ref. | |||||
>1.766 | 0.69 (0.49–0.96) | 0.002 | 0.69 (0.51–0.94) | 0.02 | 0.69 (0.49–0.96) | 0.03 |
Crude (unadjusted for confounding factors); model I (adjusted for gender, age, race, BMI, PIR, smoking, alcohol consumption); model II (adjusted for gender, age, race, BMI, PIR, smoking, alcohol consumption, hypertension, diabetes, history of TB, history of contact with active TB patients, and HIV status). NLR, neutrophil-lymphocyte ratio; TBI, tuberculosis infection; OR, odds ratio; CI, confidence interval; BMI, body mass index; PIR, poverty-income ratio; TB, tuberculosis; HIV, human immunodeficiency virus.
Subgroup analysis based on gender, hypertension, and diabetes (Table 4) uncovered that in male and hypertensive populations, there was a notable negative linkage between NLR and TBI risk. After adjusting for confounding factors in all models, the linkage between the two remained significant (OR <1, P<0.05).
Table 4
Participants | Crude | Model I | Model II | |||||
---|---|---|---|---|---|---|---|---|
OR (95% CI) | P value | OR (95% CI) | P value | OR (95% CI) | P value | |||
Gender | ||||||||
Female | 0.87 (0.70–1.07) | 0.18 | 0.91 (0.71–1.16) | 0.40 | 0.93 (0.72–1.21) | 0.52 | ||
Male | 0.73 (0.57–0.95) | 0.01 | 0.78 (0.61–0.99) | 0.03 | 0.77 (0.60–0.99) | 0.03 | ||
Hypertension | ||||||||
No | 0.87 (0.72–1.05) | 0.12 | 0.89 (0.73–1.08) | 0.22 | 0.9 (0.74–1.11) | 0.30 | ||
Yes | 0.68 (0.54–0.87) | <0.001 | 0.78 (0.64–0.96) | 0.009 | 0.77 (0.63–0.95) | 0.005 | ||
Diabetes | ||||||||
No | 0.81 (0.68–0.96) | 0.01 | 0.86 (0.74–1.01) | 0.04 | 0.87 (0.74–1.02) | 0.059 | ||
Yes | 0.58 (0.38–0.90) | 0.009 | 0.67 (0.38–1.16) | 0.06 | 0.66 (0.43–1.01) | 0.057 |
Crude (unadjusted for confounding factors); model I (adjusted for gender, age, race, BMI, PIR, smoking, alcohol consumption); model II (adjusted for gender, age, race, BMI, PIR, smoking, alcohol consumption, hypertension, diabetes, history of TB, history of contact with active TB patients, and HIV status). NLR, neutrophil-lymphocyte ratio; TBI, tuberculosis infection; OR, odds ratio; CI, confidence interval; BMI, body mass index; PIR, poverty-income ratio; TB, tuberculosis; HIV, human immunodeficiency virus.
Nonlinear association between NLR and TBI risk
The results of the RCS curve in Figure 2 manifested that there was a remarkable overall trend between NLR and TBI risk (P=0.04). In the model adjusting for all confounding factors, it was detected that the two may be linearly related (P-non-linear =0.96). The critical value of NLR was 1.899. When NLR <1.899, OR >1, it was a risk factor. When NLR >1.899, OR <1, it was a protective factor.
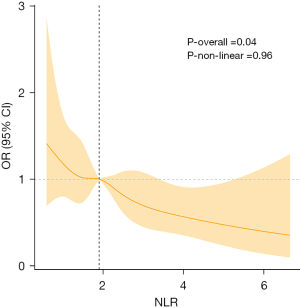
Association between NLR and TBI all-cause mortality risk
In the population of people with TBI, the optimal cutoff value of NLR level was determined by the Youden index of the ROC curves, with the optimal cutoff value being 2.328 (Figure S2). Then, NLR was stratified by quartiles. After adjusting for all confounding factors, K-M survival curves were plotted (Figure 3). As shown in the figure, compared with the group with NLR >2.328, individuals with NLR ≤2.328 possessed better survival rates (log-rank P=0.007).
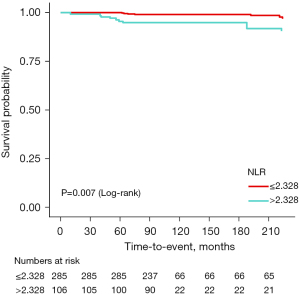
Discussion
In this investigation, we carried out a retrospective analysis of data from the NHANES database to evaluate the relationship between NLR and TBI risk. High NLR values were linked with a reduced TBI risk compared to the non-TBI population, especially in males and individuals with hypertension. RCS analysis indicated a substantial reduction in TBI risk when NLR was >1.899 (OR <1). However, the K-M survival curve demonstrated that high NLR (>2.328) was associated with adverse prognosis in TBI patients.
NLR is an easily accessible inflammatory marker, with an elevated NLR indicating a relative decrease in lymphocytes and an increase in neutrophil count, which is linked to delayed apoptosis and negative responses to treatment in inflammatory conditions, exerting important influence in the immune response to TBI (24,25). Neutrophils are the first cells to infiltrate the lungs after TBI, and they are also the most abundant cell type in bronchoalveolar lavage fluid and sputum of TB patients (26), involving in early events of immune response formation, granuloma formation, and lung structural damage (27). In addition, lymphocytes, especially T lymphocyte subgroups, have been proven to be important immune cells in combating TBI, with their levels negatively correlated with the severity of clinical TB (28). Currently, NLR is reported to be able to effectively differentiate between TBI patients and healthy children with a cut-off value of 1.5 [area under the curve (AUC): 0.72, 95% CI: 0.65–0.79], with good sensitivity (61%) and specificity (79%) (14). In the present study, when NLR >1.899, the risk of TBI was significantly reduced (OR <1). The result suggested that the NLR above this threshold may indicate a higher inflammatory response and may be linked with stronger immune defense ability (29). In addition, our results also indicated that NLR >2.328 was linked with poor survival rates in TBI patients. Former studies have reported that higher NLR represents higher clinical stages, with a close relationship between high NLR and the severity of TB (30-32), which can increase the risk of death for patients. Excessive inflammatory response may lead to tissue damage and treatment resistance (33,34). These findings have potential clinical significance for the treatment and management of TBI. The NLR level may serve as a potential indicator for personalized medicine, helping to tailor treatment plans for TBI patients with different NLR levels (35-37). For patients with lower NLR, fewer intervention measures may be required, while for patients with high NLR, more aggressive anti-inflammatory or immunomodulatory therapy may be needed.
Furthermore, compared to females, males seemed to have a lower risk of TBI. A previous study preliminarily supports our research, with a Japanese cross-sectional study indicating that TBI risk in females was higher than that in males, with 57.5% of TBI cases being females (38). This may be associated with the expression of sex hormones. Neutrophils are the most common phagocytes that play an essential part in the early response to TBI. A former animal experiment has uncovered that neutrophils can enhance the control of TBI in lipopolysaccharide (LPS) mice and increase phagocytosis of Mtb (39). There is evidence that estrogen can limit the recruitment and activity of neutrophils (40). In particular, estradiol represses neutrophil activation by reducing oxidative metabolism (41). The effects of androgens seem to be opposite to this, as a study involving male testosterone administration unveiled minimal impact on lymphocyte numbers but substantially increased levels of neutrophils in the body (42). Consistent with this, compared to normal females, hyperandrogenism in females with polycystic ovary syndrome leads to higher neutrophil counts (43). Collectively, a strong positive correlation between androgen levels and neutrophil infiltration can be indicated. Furthermore, a study on the distribution of inflammatory markers in the bodies of healthy Chinese adults has also uncovered that males exhibit higher NLR compared to females (44). Given the above extensive research, we believe that androgens can increase the value of NLR by considerably increasing neutrophils. Higher NLR is linked with a lower risk of TBI. Therefore, the linkage is significant in males.
In the pathogenesis of hypertension, the immune system mechanism is activated, in which pro-inflammatory innate and adaptive immune responses are essential for the pathogenesis of hypertension and related to elevated blood pressure and end-organ damage (45,46). Neutrophils and lymphocytes are important immune cells that affect vascular tone and blood pressure changes in the body. Lymphocytes, especially CD4+T and CD8+T cells, represent the regulatory arm of the immune system, functioning as important components of cellular immunity that can affect blood pressure by modulating the release of cytokines throughout the cardiovascular system (47). The elevated levels of interferon-gamma (IFN-γ), a key effector molecule, contribute to the exacerbation of hypertension. Moreover, the genetic polymorphisms of IFN-γ are associated with susceptibility to TB (48,49). Neutrophils, as another type of innate immune cell, may regulate the microenvironment in blood vessels by increasing oxidative stress, exacerbating endothelial dysfunction, and thus modulating blood pressure (50). In comparison to healthy individuals, the high level of inflammation in hypertensive patients may be one of the reasons protecting them from the effects of Mtb.
There are some limitations in this study. Firstly, since NHANES is a cross-sectional study, it cannot provide evidence for a causal relationship between NLR and TBI risk nor identify the direction of the causality. Secondly, the definitions of hypertension and diabetes in this study depended on the self-reported data of participants, which may introduce bias because participants may not be able to accurately recall or describe their health status. Furthermore, though the representativeness of the NHANES database, the findings based on it cannot be applied to other populations or groups except for the U.S. population. Future studies should include different races and regions to strengthen the generalizability of findings. At last, the diagnosis of TBI relies on TST and QFT-GIT, which may have limitations in specificity and sensitivity and thus may affect our accurate assessment of TBI status. Future research should consider using more precise diagnostic tools or combining multiple diagnostic methods to improve the accuracy of TBI diagnosis.
Conclusions
Our project suggests that NLR levels are substantially negatively correlated with individual TBI risk, indicating NLR may be a biomarker for assessing TBI risk. In clinical practice, monitoring of NLR may help identify high-risk individuals early. At the public health level, the accessibility of this indicator provides a potential tool for screening TBI risk in resource-limited areas. However, these findings need to be further validated in different populations to evaluate their universality and feasibility for application in different health systems. In summary, our findings emphasize the potential role of NLR in TBI risk assessment and provide valuable insights for future clinical applications and public health strategies.
Acknowledgments
None.
Footnote
Reporting Checklist: The authors have completed the TRIPOD reporting checklist. Available at https://jtd.amegroups.com/article/view/10.21037/jtd-24-750/rc
Peer Review File: Available at https://jtd.amegroups.com/article/view/10.21037/jtd-24-750/prf
Funding: None.
Conflicts of Interest: All authors have completed the ICMJE uniform disclosure form (available at https://jtd.amegroups.com/article/view/10.21037/jtd-24-750/coif). The authors have no conflicts of interest to declare.
Ethical Statement: The authors are accountable for all aspects of the work in ensuring that questions related to the accuracy or integrity of any part of the work are appropriately investigated and resolved. The study was conducted in accordance with the Declaration of Helsinki (as revised in 2013).
Open Access Statement: This is an Open Access article distributed in accordance with the Creative Commons Attribution-NonCommercial-NoDerivs 4.0 International License (CC BY-NC-ND 4.0), which permits the non-commercial replication and distribution of the article with the strict proviso that no changes or edits are made and the original work is properly cited (including links to both the formal publication through the relevant DOI and the license). See: https://creativecommons.org/licenses/by-nc-nd/4.0/.
References
- Furin J, Cox H, Pai M. Tuberculosis. Lancet 2019;393:1642-56. [Crossref] [PubMed]
.World Health Organization Tuberculosis 2023 . Available online: https://www.who.int/news-room/fact-sheets/detail/tuberculosis- Sterling TR, Njie G, Zenner D, et al. Guidelines for the Treatment of Latent Tuberculosis Infection: Recommendations from the National Tuberculosis Controllers Association and CDC, 2020. MMWR Recomm Rep 2020;69:1-11. [Crossref] [PubMed]
- Mushtaq A. New guidelines for latent tuberculosis. Lancet Infect Dis 2020;20:414. [Crossref] [PubMed]
- Cohen A, Mathiasen VD, Schön T, et al. The global prevalence of latent tuberculosis: a systematic review and meta-analysis. Eur Respir J 2019;54:1900655. [Crossref] [PubMed]
- WHO consolidated guidelines on tuberculosis: tuberculosis preventive treatment: Module 1: prevention. WHO Guidelines Approved by the Guidelines Review Committee. Geneva; 2020.
- Jilani TN, Avula A, Zafar Gondal A, et al. Active Tuberculosis. In: StatPearls. Treasure Island (FL): StatPearls Publishing; January 26, 2023.
- Uplekar M, Weil D, Lonnroth K, et al. WHO's new end TB strategy. Lancet 2015;385:1799-801. [Crossref] [PubMed]
- Lönnroth K, Jaramillo E, Williams BG, et al. Drivers of tuberculosis epidemics: the role of risk factors and social determinants. Soc Sci Med 2009;68:2240-6. [Crossref] [PubMed]
- Krug S, Parveen S, Bishai WR. Host-Directed Therapies: Modulating Inflammation to Treat Tuberculosis. Front Immunol 2021;12:660916. [Crossref] [PubMed]
- Starshinova A, Malkova A, Kudryavtsev I, et al. Tuberculosis and autoimmunity: Common features. Tuberculosis (Edinb) 2022;134:102202. [Crossref] [PubMed]
- Kissling M, Fritschi N, Baumann P, et al. Monocyte, Lymphocyte and Neutrophil Ratios - Easy-to-Use Biomarkers for the Diagnosis of Pediatric Tuberculosis. Pediatr Infect Dis J 2023;42:520-7. [Crossref] [PubMed]
- Jeon Y, Lee WI, Kang SY, et al. Neutrophil-to-Monocyte-Plus-Lymphocyte Ratio as a Potential Marker for Discriminating Pulmonary Tuberculosis from Nontuberculosis Infectious Lung Diseases. Lab Med 2019;50:286-91. [Crossref] [PubMed]
- Cursi L, Lancella L, Mariani F, et al. Monocyte-to-lymphocyte, neutrophil-to-lymphocyte and neutrophil-to-monocyte plus lymphocyte ratios in children with active tuberculosis: A multicentre study. Acta Paediatr 2023;112:2418-25. [Crossref] [PubMed]
- National Center for Health Statistics-National Health and Nutrition Examination Survey. Available online: https://www.cdc.gov/nchs/nhanes/index.htm
. Available online: https://www.cdc.gov/nchs/nhanes/irba98.htmNational Center for Health Statistics-NCHS Ethics Review Board (ERB) Approval - Chen S, Sun X, Jin J, et al. Association between inflammatory markers and bone mineral density: a cross-sectional study from NHANES 2007-2010. J Orthop Surg Res 2023;18:305. [Crossref] [PubMed]
- Zhao R, Miao W, Li B. The Relation Between Trace Elements and Latent Tuberculosis Infection: a Study Based on National Health and Nutritional Examination Survey 2011-2012. Biol Trace Elem Res 2023;201:1080-9. [Crossref] [PubMed]
- Barron MM, Shaw KM, Bullard KM, et al. Diabetes is associated with increased prevalence of latent tuberculosis infection: Findings from the National Health and Nutrition Examination Survey, 2011-2012. Diabetes Res Clin Pract 2018;139:366-79. [Crossref] [PubMed]
- Zhao Y, Li H. Association of serum vitamin C with liver fibrosis in adults with nonalcoholic fatty liver disease. Scand J Gastroenterol 2022;57:872-7. [Crossref] [PubMed]
- Li C, Shang S. Relationship between Sleep and Hypertension: Findings from the NHANES (2007-2014). Int J Environ Res Public Health 2021;18:7867. [Crossref] [PubMed]
- Miao H, Liu Y, Tsai TC, et al. Association Between Blood Lead Level and Uncontrolled Hypertension in the US Population (NHANES 1999-2016). J Am Heart Assoc 2020;9:e015533. [Crossref] [PubMed]
- Yang J, Lin JL, Liu J, et al. Estimates of prevalence, time-trend, and association of smoking in adults living with HIV, HBV, and HCV (NHANES 1999-2018). Sci Rep 2022;12:19925. [Crossref] [PubMed]
- Shojaan H, Kalami N, Ghasempour Alamdari M, et al. Diagnostic value of the neutrophil lymphocyte ratio in discrimination between tuberculosis and bacterial community acquired pneumonia: A meta-analysis. J Clin Tuberc Other Mycobact Dis 2023;33:100395. [Crossref] [PubMed]
- Berhane M, Melku M, Amsalu A, et al. The Role of Neutrophil to Lymphocyte Count Ratio in the Differential Diagnosis of Pulmonary Tuberculosis and Bacterial Community-Acquired Pneumonia: a Cross-Sectional Study at Ayder and Mekelle Hospitals, Ethiopia. Clin Lab 2019.
- Hilda JN, Das S, Tripathy SP, et al. Role of neutrophils in tuberculosis: A bird's eye view. Innate Immun 2020;26:240-7. [Crossref] [PubMed]
- Parker HA, Forrester L, Kaldor CD, et al. Antimicrobial Activity of Neutrophils Against Mycobacteria. Front Immunol 2021;12:782495. [Crossref] [PubMed]
- Li F, Chen D, Zeng Q, et al. Possible Mechanisms of Lymphopenia in Severe Tuberculosis. Microorganisms 2023;11:2640. [Crossref] [PubMed]
- Letourneau EJ, Brown DS, Fang X, et al. The economic burden of child sexual abuse in the United States. Child Abuse Negl 2018;79:413-22. [Crossref] [PubMed]
- Nakao M, Muramatsu H, Arakawa S, et al. Immunonutritional status and pulmonary cavitation in patients with tuberculosis: A revisit with an assessment of neutrophil/lymphocyte ratio. Respir Investig 2019;57:60-6. [Crossref] [PubMed]
- Gu Z, Liu B, Yu X, et al. Association of blood neutrophil-lymphocyte ratio with short-term prognosis and severity of tuberculosis meningitis patients without HIV infection. BMC Infect Dis 2023;23:449. [Crossref] [PubMed]
- Sun JY, Mu N, Mu J, et al. Significance of Neutrophil/Lymphocyte Ratio and Platelet/Lymphocyte Ratio in Patients with Multiple Myeloma. Zhongguo Shi Yan Xue Ye Xue Za Zhi 2019;27:1185-9. [PubMed]
- Hur K, Zhang P, Yu A, et al. Open Versus Endoscopic Approach for Sinonasal Melanoma: A Systematic Review and Meta-analysis. Am J Rhinol Allergy 2019;33:162-9. [Crossref] [PubMed]
- La HM, Chan AL, Legrand JMD, et al. GILZ-dependent modulation of mTORC1 regulates spermatogonial maintenance. Development 2018;145:dev165324. [Crossref] [PubMed]
- Delgado-Miguel C, Muñoz-Serrano AJ, Moratilla L, et al. Neutrophil-to-lymphocyte ratio as a predictor of surgical site infection in acute appendicitis. Transl Pediatr 2023;12:552-9. [Crossref] [PubMed]
- He Q, Du S, Wang X, et al. Development and validation of a nomogram based on neutrophil-to-lymphocyte ratio and fibrinogen-to-lymphocyte ratio for predicting recurrence of colorectal adenoma. J Gastrointest Oncol 2022;13:2269-81. [Crossref] [PubMed]
- Xiang Y, Zhang N, Lei H, et al. Neutrophil-to-lymphocyte ratio is a negative prognostic biomarker for luminal A breast cancer. Gland Surg 2023;12:415-25. [Crossref] [PubMed]
- Kawatsu L, Uchimura K, Ohkado A. Trend and treatment status of latent tuberculosis infection patients in Japan - Analysis of Japan TB Surveillance data. PLoS One 2017;12:e0186588. [Crossref] [PubMed]
- Piergallini TJ, Scordo JM, Pino PA, et al. Acute Inflammation Confers Enhanced Protection against Mycobacterium tuberculosis Infection in Mice. Microbiol Spectr 2021;9:e0001621. [Crossref] [PubMed]
- Averyanova M, Vishnyakova P, Yureneva S, et al. Sex hormones and immune system: Menopausal hormone therapy in the context of COVID-19 pandemic. Front Immunol 2022;13:928171. [Crossref] [PubMed]
- Mauvais-Jarvis F, Klein SL, Levin ER. Estradiol, Progesterone, Immunomodulation, and COVID-19 Outcomes. Endocrinology 2020;161:bqaa127. [Crossref] [PubMed]
- Gagliano-Jucá T, Pencina KM, Guo W, et al. Differential effects of testosterone on circulating neutrophils, monocytes, and platelets in men: Findings from two trials. Andrology 2020;8:1324-31. [Crossref] [PubMed]
- Shabbir S, Khurram E, Moorthi VS, et al. The interplay between androgens and the immune response in polycystic ovary syndrome. J Transl Med 2023;21:259. [Crossref] [PubMed]
- Wang J, Zhang F, Jiang F, et al. Distribution and reference interval establishment of neutral-to-lymphocyte ratio (NLR), lymphocyte-to-monocyte ratio (LMR), and platelet-to-lymphocyte ratio (PLR) in Chinese healthy adults. J Clin Lab Anal 2021;35:e23935. [Crossref] [PubMed]
- Van Beusecum JP, Moreno H, Harrison DG. Innate immunity and clinical hypertension. J Hum Hypertens 2022;36:503-9. [Crossref] [PubMed]
- Mikolajczyk TP, Guzik TJ. Adaptive Immunity in Hypertension. Curr Hypertens Rep 2019;21:68. [Crossref] [PubMed]
- Rai A, Narisawa M, Li P, et al. Adaptive immune disorders in hypertension and heart failure: focusing on T-cell subset activation and clinical implications. J Hypertens 2020;38:1878-89. [Crossref] [PubMed]
- Benson LN, Liu Y, Deck K, et al. IFN-γ Contributes to the Immune Mechanisms of Hypertension. Kidney360. 2022;3:2164-73. [Crossref] [PubMed]
- Hassuna NA, El Feky M, Hussein AARM, et al. Interleukin-18 and interferon-γ single nucleotide polymorphisms in Egyptian patients with tuberculosis. PLoS One 2021;16:e0244949. [Crossref] [PubMed]
- Araos P, Figueroa S, Amador CA. The Role of Neutrophils in Hypertension. Int J Mol Sci 2020;21:8536. [Crossref] [PubMed]