The U-shaped relationship between admission peripheral oxygen saturation and all-cause hospital mortality in acute exacerbation of chronic obstructive pulmonary disease: a retrospective analysis using the MIMIC III database
Highlight box
Key findings
• This study investigated the U-shaped nonlinear relationship between admission oxygen saturation (SpO2) and all-cause hospital mortality in patients with acute exacerbation of chronic obstructive pulmonary disease (AECOPD). The results showed that the lowest all-cause hospital mortality was observed at an SpO2 of 89.5%. Additionally, SpO2 was identified as an independent risk factor for predicting all-cause hospital mortality in AECOPD patients, providing valuable guidance for optimizing oxygen therapy in this population.
What is known and what is new?
• Most studies indicate that maintaining SpO2 levels between 88–92% provides significant benefits for AECOPD patients. However, there is limited research on the relationship between SpO2 and all-cause hospital mortality in AECOPD patients.
• This study investigated the nonlinear U-shaped relationship between SpO2 and all-cause hospital mortality in AECOPD patients. It offers a readily available indicator for assessing the severity of AECOPD and provides evidence to guide oxygen therapy in clinical practice.
What is the implication, and what should change now?
• More prospective studies are urgently needed to explore the relationship between SpO2 and all-cause hospital mortality in AECOPD patients, providing further evidence to improve the prognosis of these patients.
Introduction
Chronic obstructive pulmonary disease (COPD) stands out as a chronic respiratory condition marked by diverse clinical manifestations arising from the interplay between genetic factors and environmental influences (1). It is recognized globally as a substantial public health challenge, with China contributing a significant share, encompassing a quarter of the worldwide COPD population (2). This prevalence imposes a noteworthy social and economic burden on healthcare systems. Throughout the course of COPD development, acute exacerbation of chronic obstructive pulmonary disease (AECOPD), triggered by various factors, emerge as critical contributors to adverse outcomes such as patient mortality, intensive care unit (ICU) admissions, mechanical ventilation necessity, prolonged hospital stays, and impaired lung function (3).
Hence, the scientific management and precise assessment of AECOPD severity assume paramount importance in enhancing the prognosis of individuals experiencing such exacerbations. The measurement of peripheral capillary oxygen saturation (SpO2) emerges as a potentially crucial indicator for evaluating the severity of AECOPD. Its attributes, including cost-effectiveness, non-invasiveness, and ease of repetitive measurement, position it as a valuable tool in this regard.
Most recent studies propose that maintaining SpO2 levels within the range of 88% to 92% is advantageous for individuals undergoing AECOPD (4-8). Conversely, in clinical settings, healthcare providers often prioritize sustaining SpO2 levels between 94% and 98% for AECOPD patients (9), a practice inconsistent with established theoretical research. Consequently, there is a need for further investigation into the correlation between SpO2 levels and adverse outcomes, including mortality, in AECOPD patients, to furnish evidence for evaluating patient outcomes.
For patients with AECOPD, higher SpO2 levels do not necessarily equate to better outcomes. The relationship between SpO2 levels at admission and all-cause hospital mortality in AECOPD patients remains unclear, with limited research addressing this connection. Further investigation is needed to understand this association and its implications for patient prognosis.
Objectives
This study aimed to explore the relationship between SpO2 levels and all-cause hospital mortality in AECOPD patients, with the goal of identifying safe and practical SpO2 thresholds for clinical diagnosis and assessing disease severity in this population. The findings from this study were anticipated to provide a foundation for subsequent research into the complex relationship between SpO2 levels and all-cause hospital mortality in AECOPD patients. We present this article in accordance with the STROBE reporting checklist (available at https://jtd.amegroups.com/article/view/10.21037/jtd-24-1404/rc).
Methods
Study design and setting
Data source and ethical statement
The data for this study originates exclusively from the Medical Information Mart for Intensive Care (MIMIC) III database (version 1.3), a freely accessible repository of intensive care information. The database encompasses details on 38,645 adults and 7,875 neonates admitted to various ICUs, including surgical, trauma surgical, coronary, and cardiac surgery recovery units at Boston’s Beth Israel Deaconess Medical Center (BIDMC) during the period spanning 2001 to 2012 (10). Comprehensive records within the MIMIC III database encompass demographic information, vital signs, laboratory results, blood gas analyses, prognostic scoring systems, and survival data. Diagnoses are documented using International Classification of Diseases, ninth revision (ICD-9) codes assigned by hospital staff at the time of patient discharge. Hourly, ICU nurses verify and record physiological readings obtained from bedside monitoring devices.
To ensure adherence to ethical guidelines, we successfully completed the “Protection of Human Research Participants” exam (certificate ID 52148401) and acquired official authorization to access the dataset. The study conformed to the provisions of the Declaration of Helsinki (as revised in 2013).
Inclusion and exclusion criteria
This research included patients admitted to the ICU diagnosed with AECOPD based on the ICD-9 code from the MIMIC III database, with exclusion criteria applied to those with incomplete clinical data.
Data extraction
Utilizing structured query language (SQL), patient data were retrieved from the database for this study. Extracted variables included demographic information, vital signs, comorbidities, and laboratory parameters. Key cardiopulmonary variables, such as heart rate, respiratory rate, SpO2 levels, and temperature, were meticulously recorded by ICU nursing staff. Demographic data included gender, age, and race, while vital signs covered SpO2, heart rate, respiratory rate, and blood pressure. Vital signs encompassed SpO2, heart rate, respiratory rate, and blood pressure. Comorbidities included chronic kidney failure, chronic heart failure, hypertension, and diabetes. The laboratory parameters considered comprised arterial oxygen partial pressure (PaO2), arterial carbon dioxide partial pressure (PaCO2), arterial blood pH, albumin, hemoglobin, white blood cell count, serum potassium concentration, sodium concentration, and chloride concentration.
Study outcome
This study primarily centers on the overall mortality within the hospital setting, regardless of the cause.
Statistical methods
In this study, continuous variables are expressed using medians and interquartile ranges (IQRs), while categorical variables are conveyed through total counts and percentages. Baseline characteristics among the included patients were compared using Kruskal-Wallis rank tests for continuous variables and Pearson chi-square tests for categorical variables.
To examine the non-linear correlation between admission SpO2 levels and all-cause hospital mortality, generalized additive models (GAMs) were applied. GAMs extend generalized linear models, allowing for flexible modeling of relationships between independent and dependent variables without assuming linearity, thus providing a more accurate description of these associations. Analyses were conducted using the “mgcv” package in R software (version 4.1.1). Additionally, receiver operating characteristic (ROC) curves and Kaplan-Meier survival curves were used to further investigate this relationship. ROC analysis was employed to identify the optimal SpO2 cutoff value for predicting all-cause hospital mortality, allowing for subsequent categorization of SpO2 values into two ranges for further analysis.
Based on the aforementioned SpO2 categorizations, our analysis included age, gender, race, heart rate, respiratory rate, blood pressure, PaO2, PaCO2, pH, chronic kidney failure, chronic heart failure, hypertension, and diabetes as covariates. Cox proportional hazards models were employed to assess the relationship between SpO2 and all-cause hospital mortality rates, both in univariate and multivariate analyses. The results are depicted as hazard ratios (HRs) along with 95 % confidence intervals (CIs).
To further validate the relationship between SpO2 and all-cause hospital mortality rates, we classified the covariates into different categories, constructing various Cox survival analysis models. These models include: Model 1 (SpO2, gender), Model 2 (SpO2, hypertension, diabetes, chronic kidney failure, chronic heart failure), Model 3 (SpO2, age, PaO2, PaCO2, pH, heart rate, respiratory rate), and Model 4 (SpO2, age, white blood cell count).
The statistical analysis was carried out using SPSS version 26 and R version 4.1.1, with significance set at P<0.05.
Results
Participants and descriptive data
Baseline characteristics
Out of 1,198 patients identified with AECOPD in the MIMIC III database using the ICD-9 code, 996 met the inclusion and exclusion criteria for this study. The flowchart outlining the patient selection process is depicted in Figure 1. Patients included in the study were divided into two categories: those who succumbed (134 patients) and those who survived (862 patients). Table 1 provides a summary of baseline information for both groups.
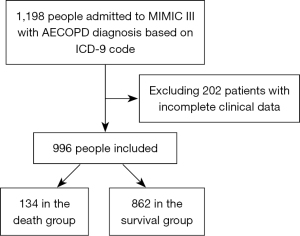
Table 1
Characters | Total (n=996) | Survival (n=862) | No survival (n=134) | Z/χ2† | P value |
---|---|---|---|---|---|
Age (years) | 71.09 (64.23–78.17) | 70.32 (63.62–77.67) | 76.28 (69.47–82.43) | 3.28 | <0.001 |
Gender, male | 366 (36.7) | 295 (34.2) | 71 (53.0) | 17.56 | <0.001 |
Ethnicity, White | 721 (72.4) | 619 (71.8) | 101 (75.4) | 0.72 | 0.66 |
Time in ICU (day) | 2.97 (1.58–5.97) | 2.94 (1.58–5.84) | 3.28 (1.59–7.30) | 0.98 | 0.32 |
SpO2 (%) | 91.00 (88.00–93.00) | 91.00 (88.00–93.25) | 89.00 (85.00–93.00) | 2.66 | 0.002 |
SBP (mmHg) | |||||
Min-SBP | 91.00 (82.00–102.00) | 92.00 (82.00–102.75) | 87.00 (76.00–98.75) | 2.69 | <0.001 |
Max-SBP | 150.00 (134.00–165.00) | 150.00 (135.00–165.00) | 147.50 (131.25–160.00) | 1.52 | 0.08 |
Average-SBP | 117.59 (107.90–129.53) | 118.18 (108.42–130.73) | 112.62 (105.15–123.55) | 2.73 | <0.001 |
DBP (mmHg) | |||||
Min-DBP | 43.00 (35.00–49.00) | 43.00 (36.00–49.00) | 41.00 (30.25–46.75) | 2.61 | <0.001 |
Max-DBP | 83.00 (72.00–94.00) | 83.00 (73.00–94.00) | 79.00 (68.00–91.00) | 2.17 | 0.02 |
Average-DBP | 59.59 (53.88–66.26) | 60.13 (54.13–66.60) | 56.85 (51.18–62.85) | 3.20 | <0.001 |
Temperature (℃) | |||||
Min-temperature | 36.11 (35.67–36.44) | 36.11 (35.72–36.44) | 36.00 (35.56–36.50) | 1.17 | 0.25 |
Max-temperature | 37.17 (36.89–37.67) | 37.17 (36.89–37.67) | 37.11 (36.72–37.56) | 1.44 | 0.09 |
Average-temperature | 36.68 (36.36–37.01) | 36.68 (36.37–37.02) | 36.67 (36.26–37.00) | 0.80 | 0.14 |
PaO2 (mmHg) | 97.00 (71.00–157.00) | 97.00 (71.00–157.00) | 90.00 (67.00–161.00) | 0.30 | 0.69 |
PaCO2 (mmHg) | 55.00 (44.00–71.00) | 55.00 (44.00–71.00) | 54.00 (43.00–73.00) | 0.62 | 0.57 |
pH | 7.34 (7.26–7.40) | 7.34 (7.27–7.40) | 7.32 (7.24–7.39) | 1.24 | 0.15 |
Breath frequency (min−1) | 20.00 (17.00–23.00) | 20.00 (17.00–22.00) | 20.00 (17.00–23.00) | 1.22 | 0.16 |
Heart frequency (min−1) | 89.00 (79.00–99.00) | 89.00 (79.00–99.00) | 88.00 (75.00–100.00) | 0.76 | 0.46 |
Mechanical ventilation | 499 (50.1) | 424 (49.2) | 75 (56.0) | 2.13 | 0.16 |
Albumin (g/dL) | 3.30 (2.80–3.60) | 3.30 (2.90–3.70) | 2.90 (2.58–3.50) | 2.69 | <0.001 |
Min-BG (mg/dL) | 117.00 (96.00–140.00) | 117.50 (97.00–142.75) | 112.50 (92.75–135.25) | 0.29 | 0.72 |
Max-BG (mg/dL) | 166.00 (137.00–213.00) | 166.50 (140.00–211.75) | 165.00 (124.25–219.25) | 0.63 | 0.52 |
Min-hemoglobin (g/dL) | 10.40 (9.20–11.93) | 10.40 (9.20–12.00) | 10.20 (9.10–11.50) | 1.12 | 0.22 |
Max-hemoglobin (g/dL) | 11.60 (10.30–13.10) | 11.60 (10.30–13.20) | 11.50 (10.40–12.80) | 0.64 | 0.53 |
Min-PLT (k/μL) | 227.00 (176.00–295.00) | 229.00 (179.00–296.00) | 214.00 (145.00–289.00) | 2.09 | 0.03 |
Max-PLT (k/μL) | 256.50 (199.75–37.00) | 261.00 (201.75–337.00) | 241.00 (183.00–333.00) | 1.34 | 0.10 |
Min-Cl (mmol/L) | 99.00 (96.00–104.00) | 99.00 (96.00–103.00) | 100.00 (96.00–105.00) | 1.63 | 0.09 |
Max-Cl (mmol/L) | 103.00 (99.00–107.00) | 103.00 (99.00–107.00) | 105.00 (100.00–109.00) | 2.00 | 0.04 |
Min-K (mmol/L) | 4.00 (3.60–4.40) | 4.00 (3.60–4.40) | 4.00 (3.60–4.40) | 0.83 | 0.49 |
Max-K (mmol/L) | 4.60 (4.20–5.10) | 4.60 (4.20–5.10) | 4.80 (4.20–5.40) | 2.01 | 0.04 |
Min-Na (mmol/L) | 138.00 (135.00–141.00) | 138.00 (135.00–141.00) | 138.00 (135.00–141.00) | 0.35 | 0.74 |
Max-Na (mmol/L) | 141.00 (138.00–143.00) | 141.00 (138.00–143.00) | 141.00 (138.00–144.00) | 1.41 | 0.19 |
Min-WBC (k/μL) | 9.90 (7.30–13.60) | 9.70 (7.30–13.08) | 11.30 (7.50–16.50) | 3.27 | 0.001 |
Max-WBC (k/μL) | 12.45 (9.00–17.10) | 12.20 (9.00–16.50) | 14.70 (10.80–20.70) | 3.67 | <0.001 |
CHF | 494 (49.6) | 418 (48.5) | 75 (56.0) | 3.14 | 0.16 |
Hypertension | 563 (56.5) | 488 (56.6) | 74 (55.2) | 0.09 | 0.64 |
Diabetes | 43 (4.3) | 37 (4.3) | 5 (3.7) | 0.09 | 0.82 |
CKF | 147 (14.8) | 124 (14.4) | 23 (17.2) | 0.71 | 0.52 |
†, the Kruskal-Wallis rank test and Pearson χ2 test were used to compare the baseline characteristics of the enrolled patients. Continuous variables are presented as median (interquartile range), and categorical variables are presented as count (percentage). ICU, intensive care unit; SpO2, pulse oximetry-derived oxygen saturation; PaO2, arterial oxygen partial pressure; PaCO2, arterial carbon dioxide partial pressure; SBP, systolic blood pressure; DBP, diastolic blood pressure; min, minimum; max, maximum; BG, blood glucose; PLT, platelet count; WBC, white blood cell; CHF, chronic heart failure; CKF, chronic kidney failure.
Patients in the deceased group exhibited higher mean age (76.28 vs. 70.32 years; P<0.001) and elevated white blood cell counts (11.30 vs. 9.70 k/µL; P=0.001) in contrast to those in the survival group. Furthermore, the deceased group had lower levels of albumin (2.90 vs. 3.30 g/dL; P<0.001) and admission SpO2 levels (89% vs. 91%; P=0.002) compared to the survivors.
Outcome data and main results
The relationship between SpO2 and all-cause hospital mortality in patients with AECOPD
The correlation between the admission SpO2 level and all-cause hospital mortality demonstrated a non-linear relationship, resembling a U-shaped curve. Notably, the analysis revealed that the lowest all-cause hospital mortality occurred at an SpO2 level of 89.5%. When SpO2 levels exceeded or fell below 89.5%, the all-cause hospital mortality rate for AECOPD patients demonstrated an upward trend, as shown in Figure 2. To further evaluate this relationship, a ROC curve was plotted, revealing an AUC of 0.565 (P<0.001). This analysis determined that the optimal cutoff value for predicting the mortality of AECOPD patients based on admission SpO2 was 89.5%, depicted in Figure 3. Consequently, we categorized SpO2 into two groups: ≥89.5% and <89.5% for subsequent statistical analysis.
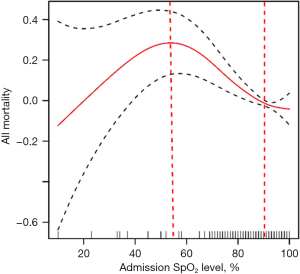
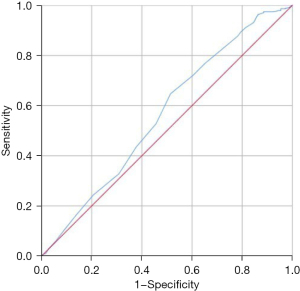
Survival analysis
Figure 4 displays the Kaplan-Meier survival curve for patients within distinct SpO2 groups, with a log-rank test indicating statistical significance (P<0.001). Cox univariate and multivariate regression analyses were employed to initially investigate the correlation between admission SpO2 and all-cause hospital mortality in AECOPD patients as shown in Table 2.
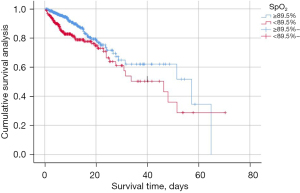
Table 2
Characters | Univariate | Multivariate | |||
---|---|---|---|---|---|
HR (95% CI) | P value | HR (95% CI) | P value | ||
Age | 1.05 (1.03–1.07) | <0.001 | 1.06 (1.03–1.08) | <0.001 | |
Gender | |||||
Male | 0.84 (0.45–1.55) | 0.57 | |||
Female | Ref | Ref | |||
Race | |||||
White | 1.39 (0.66–2.93) | 0.39 | |||
Other | 0.40 (0.09–1.84) | 0.24 | |||
Unknown | Ref | Ref | |||
Heart frequency | 1.00 (0.98–1.01) | 0.40 | |||
Breath frequency | 1.04 (0.99–1.08) | 0.11 | |||
Temperature | 1.26 (0.68–2.32) | 0.46 | |||
SpO2 | 0.98 (0.97–1.00) | 0.02 | 0.97 (0.95–0.99) | 0.001 | |
PaO2 | 1.00 (0.99–1.00) | 0.73 | |||
PaCO2 | 1.00 (0.99–1.01) | 0.83 | |||
pH | 0.21 (0.04–1.08) | 0.06 | |||
WBC | 1.04 (1.02–1.05) | <0.001 | 1.04 (1.02–1.05) | <0.001 | |
Hb | 1.02 (0.93–1.11) | 0.71 | |||
ALB | 0.79 (0.53–1.17) | 0.24 | |||
Na+ | |||||
Min-Na+ | 1.01 (0.97–1.04) | 0.67 | |||
Max-Na+ | 1.03 (0.99–1.07) | 0.19 | |||
K+ | |||||
Min-K+ | 1.19 (0.88–1.60) | 0.26 | |||
Max-K+ | 1.13 (0.96–1.33) | 0.15 | |||
Cl− | |||||
Min-Cl− | 1.01 (0.98–1.04) | 0.49 | |||
Max-Cl− | 1.02 (0.99–1.05) | 0.15 | |||
Comorbidity | |||||
Hypertension | 1.06 (0.75–1.50) | 0.75 | |||
Diabetes | 0.78 (0.32–1.91) | 0.59 | |||
CHF | 1.11 (0.79–1.56) | 0.55 | |||
CKF | 1.12 (0.71–1.76) | 0.62 |
HR, hazard ratio; CI, confidence interval; ref, reference; SpO2, pulse oximetry-derived oxygen saturation; WBC, white blood cell; CHF, chronic heart failure; CKF, chronic kidney failure; ALB, albumin; Hb, hemoglobin; PaO2, arterial oxygen partial pressure; PaCO2, arterial carbon dioxide partial pressure.
The Cox univariate regression analysis highlighted age, SpO2, and white blood cell count as potential predictors of all-cause hospital mortality in AECOPD patients. Subsequently, in the multivariate analysis incorporating age, SpO2, and white blood cell count into the Cox regression model, SpO2 emerged as an independent risk factor for death in AECOPD patients.
To further substantiate the relationship between SpO2 and all-cause hospital mortality in AECOPD patients, different covariates were introduced into distinct Cox regression models. Results were as follows: Model 1 (SpO2, gender: HR =0.5; 95% CI: 0.36–0.71; P<0.001), Model 2 (SpO2, hypertension, diabetes, chronic renal insufficiency, chronic heart failure: HR =0.55; 95% CI: 0.39–0.78; P<0.001), Model 3 (SpO2, age, PaO2, PaCO2, pH, heart rate, respiratory rate: HR =0.57; 95% CI: 0.39–0.82; P<0.001), and Model 4 (SpO2, age, white blood cell count: HR =0.52; 95% CI: 0.37–0.74; P<0.001). Results can be summarized in Table 3.
Table 3
Model | P value | HR | 95% CI |
---|---|---|---|
Model 1 | <0.001 | 0.5 | 0.36–0.71 |
Model 2 | <0.001 | 0.55 | 0.39–0.78 |
Model 3 | <0.001 | 0.57 | 0.39–0.82 |
Model 4 | <0.001 | 0.52 | 0.37–0.74 |
Model 1: SpO2, gender; Model 2: SpO2, hypertension, diabetes, chronic kidney failure, chronic heart failure; Model 3: SpO2, age, PaO2, PaCO2, pH, heart rate, respiratory rate; Model 4: SpO2, age, white blood cell count. SpO2, pulse oximetry-derived oxygen saturation; AECOPD, acute exacerbation of chronic obstructive pulmonary disease; HR, hazard ratio; CI, confidence interval; PaO2, arterial oxygen partial pressure; PaCO2, arterial carbon dioxide partial pressure.
Discussion
AECOPD emerges as a pivotal factor leading to adverse outcomes like death, ICU admission, mechanical ventilation, and impaired lung function in COPD patients. Consequently, evaluating the severity of AECOPD becomes essential to curtail unnecessary hospitalizations and excessive testing, particularly in low-risk AECOPD patients. Established prognostic tools, including the DECAF score, Glasgow prognostic score (GPS), MAGENTA model, and CURB-65 score, exhibit predictive capabilities for adverse events in AECOPD patients (11-15). However, their operational complexity poses challenges for swift assessments of critically ill patients, warranting further validation for their effectiveness in prognostic evaluations.
To the best of our knowledge, this study is the first to systematically examine the relationship between admission SpO2 levels and all-cause hospital mortality in AECOPD patients using GAM. Our findings reveal a non-linear, U-shaped relationship between admission SpO2 levels and hospital mortality in these patients. Notably, an SpO2 level of 89.5% at admission was identified as the point associated with the lowest mortality rate. This result suggests that targeting an SpO2 level of 89.5% could serve as an optimal reference for oxygen therapy and prognosis assessment in AECOPD patients. Additionally, our study supports the role of admission SpO2 as an independent prognostic factor for all-cause hospital mortality, as confirmed by Cox multivariate regression analysis.
Recently, numerous studies have investigated the correlation between SpO2 levels, oxygen therapy modes, and the mortality rate of AECOPD patients. The oxygen therapy guideline of the British Thoracic Society suggests maintaining SpO2 at 88–92% for patients at risk of hypercapnia and respiratory failure, such as those with COPD, where the greatest survival benefit is observed (5). Echevarria et al. categorized AECOPD patients into three groups based on admission SpO2 levels (97–100%, 93–96%, and 88–92%), finding that patients in the 88–92% group had the highest survival rate, irrespective of comorbid hypercapnia (4). Pilcher et al. derived a curve from previous research indicating the relationship between SpO2 levels and mortality rates in AECOPD patients, concluding that the optimal SpO2 target is 88–92% (6). A randomized controlled study demonstrated a 58% reduction in the death risk of AECOPD patients by maintaining oxygen therapy with SpO2 titration between 88% and 92% before admission, along with decreased hospital stays and hypercapnia risk (7,8). Unlike prior studies, however, this study specifically highlights SpO2’s role as an independent prognostic marker, rather than only as a therapeutic target, thus providing new perspectives on the value of SpO2 levels in assessing disease severity and prognosis in AECOPD.
In our investigation, we employed GAM curves to scrutinize the correlation between SpO2 levels and all-cause hospital mortality in AECOPD patients, establishing a fundamental framework for assessing the severity and prognosis of AECOPD. Anderson et al. noted a markedly higher median proportion of SpO2 exceeding 92% among AECOPD patients experiencing adverse events (16). Another study proposed that the oxygen hemoglobin value (hemoglobin value × SpO2) independently predicts long-term mortality in AECOPD patients, indirectly suggesting the possible role of SpO2 as an independent factor in prognosis assessment (17). Our use of Cox multivariate analysis further confirmed SpO2 as an independent predictor of all-cause hospital mortality in AECOPD patients, underscoring its relevance in clinical prognostication.
The non-linear correlation between admission SpO2 levels and all-cause in-hospital mortality in AECOPD patients underscores the physiological significance of maintaining normal oxygenation while avoiding both hypoxia and hyperoxia. This relationship may be linked to various mechanisms. Excessive oxygen can induce the overproduction of cytotoxic reactive oxygen species in cells, overwhelming physiological antioxidant defense mechanisms and resulting in an imbalance in the “oxidative stress response”, potentially leading to lung injury (18,19). This could explain the observed increase in mortality when SpO2 surpasses 89.5% in AECOPD patients.
When oxygen levels surpass 89.5%, excessive oxygenation may counteract hypoxic pulmonary vasoconstriction, disrupting ventilation-perfusion matching. This over-oxygenation could exacerbate hypercapnia and elevate the risk of adverse events, including death, due to the Haldane effect and the “oxygen-driven” elimination of CO2. Additionally, elevated oxygen saturation can contribute to direct pulmonary toxicity, increased atelectasis, and vasoconstriction in key vascular beds, such as those of the brain and coronary arteries, causing cellular damage (20-23).
Further in-depth basic research is essential to elucidate these underlying mechanisms, establishing a solid research foundation for the standardization of oxygen therapy and prognostic evaluation in AECOPD patients.
Limitations of the study
We recognize several limitations within our study. Firstly, it is imperative to recognize that this study is a single-center retrospective observational analysis, inherently susceptible to selection bias. Consequently, further comprehensive cohort studies are warranted to delve deeper into our findings and validate their robustness.
A significant limitation of this study was the substantial data loss, which prevented the evaluation of certain potential confounding variables. The exclusion of these variables, which may hold predictive value for clinical outcomes, could impact the robustness of our findings (23). Consequently, external validation is essential to confirm the reliability of our results. Additionally, missing data limited the number of samples included, leading to a potentially lower AUC in our analysis. In future studies, we aim to incorporate more covariates to enhance the accuracy of the results.
Lastly, our study exclusively concentrated on all-cause in-hospital mortality in AECOPD patients. However, it is imperative to acknowledge that other effects, including long-term mortality and the incidence of other adverse events, are equally significant and warrant further investigation.
Conclusions
In conclusion, our retrospective study utilizing the MIMIC III database aimed to investigate the relationship between peripheral SpO2 levels and all-cause hospital mortality in patients experiencing AECOPD. Among the 1,198 identified AECOPD patients, 996 met the study criteria, and their baseline characteristics revealed significant differences between survivors and non-survivors.
The study revealed a non-linear relationship between admission SpO2 levels and all-cause hospital mortality, represented by a U-shaped curve. Notably, the lowest mortality rate was associated with an SpO2 level of 89.5%. This optimal cutoff value was further supported by ROC curve analysis. The study employed Kaplan-Meier survival analysis and Cox univariate and multivariate regression analyses, establishing SpO2 as an independent prognostic predictor of all-cause hospital mortality in AECOPD patients.
The findings align with existing literature on oxygen therapy guidelines, emphasizing the importance of maintaining SpO2 within the 88–92% range for optimal survival benefits in AECOPD patients. This study enhances our understanding of the complex relationship between admission SpO2 levels and hospital mortality, offering valuable insights for guiding oxygen therapy and assessing prognosis in AECOPD patients. These findings provide a potential basis for improving patient outcomes through targeted oxygen management. However, acknowledging study limitations, including its retrospective nature and potential confounders, further research and external validation are essential to strengthen the robustness of these findings. Additionally, future investigations should explore other relevant outcomes such as long-term mortality and additional adverse events in AECOPD patients.
Acknowledgments
None.
Footnote
Reporting Checklist: The authors have completed the STROBE reporting checklist. Available at https://jtd.amegroups.com/article/view/10.21037/jtd-24-1404/rc
Peer Review File: Available at https://jtd.amegroups.com/article/view/10.21037/jtd-24-1404/prf
Funding: The study was funded by
Conflicts of Interest: All authors have completed the ICMJE uniform disclosure form (available at https://jtd.amegroups.com/article/view/10.21037/jtd-24-1404/coif). The authors have no conflicts of interest to declare.
Ethical Statement: The authors are accountable for all aspects of the work in ensuring that questions related to the accuracy or integrity of any part of the work are appropriately investigated and resolved. The study was approved by “Protection of Human Research Participants” exam (certificate ID 52148401) and obtained permission to access the dataset. The study conformed to the provisions of the Declaration of Helsinki (as revised in 2013).
Open Access Statement: This is an Open Access article distributed in accordance with the Creative Commons Attribution-NonCommercial-NoDerivs 4.0 International License (CC BY-NC-ND 4.0), which permits the non-commercial replication and distribution of the article with the strict proviso that no changes or edits are made and the original work is properly cited (including links to both the formal publication through the relevant DOI and the license). See: https://creativecommons.org/licenses/by-nc-nd/4.0/.
References
- Yang J, Zhang Q, Zhang J, et al. Exploring the Change of Host and Microorganism in Chronic Obstructive Pulmonary Disease Patients Based on Metagenomic and Metatranscriptomic Sequencing. Front Microbiol 2022;13:818281. [Crossref] [PubMed]
- Yin P, Wu J, Wang L, et al. The Burden of COPD in China and Its Provinces: Findings From the Global Burden of Disease Study 2019. Front Public Health 2022;10:859499. [Crossref] [PubMed]
- Kim V, Aaron SD. What is a COPD exacerbation? Current definitions, pitfalls, challenges and opportunities for improvement. Eur Respir J 2018;52:1801261. [Crossref] [PubMed]
- Echevarria C, Steer J, Wason J, et al. Oxygen therapy and inpatient mortality in COPD exacerbation. Emerg Med J 2021;38:170-7. [Crossref] [PubMed]
- O'Driscoll BR. British Thoracic Society Oxygen Guidelines: another clinical brick in the wall. Thorax 2017;72:498-9. [Crossref] [PubMed]
- Pilcher JM, Kearns C, Beasley R. Searching for the optimal oxygen saturation range in acutely unwell patients. Emerg Med J 2021;38:168-9. [Crossref] [PubMed]
- Austin MA, Wills KE, Blizzard L, et al. Effect of high flow oxygen on mortality in chronic obstructive pulmonary disease patients in prehospital setting: randomised controlled trial. BMJ 2010;341:c5462. [Crossref] [PubMed]
- Ntoumenopoulos G. Using titrated oxygen instead of high flow oxygen during an acute exacerbation of chronic obstructive pulmonary disease (COPD) saves lives. J Physiother 2011;57:55. [Crossref] [PubMed]
- O'Driscoll BR, Howard LS, Earis J, et al. BTS guideline for oxygen use in adults in healthcare and emergency settings. Thorax 2017;72:ii1-ii90. [Crossref] [PubMed]
- Johnson AE, Pollard TJ, Shen L, et al. MIMIC-III, a freely accessible critical care database. Sci Data 2016;3:160035. [Crossref] [PubMed]
- Bansal AG, Gaude GS. Predictors of mortality in acute exacerbations of chronic obstructive pulmonary disease using the dyspnea, eosinopenia, consolidation, acidemia and atrial fibrillation score. Lung India 2020;37:19-23. [Crossref] [PubMed]
- Huang Q, He C, Xiong H, et al. DECAF score as a mortality predictor for acute exacerbation of chronic obstructive pulmonary disease: a systematic review and meta-analysis. BMJ Open 2020;10:e037923. [Crossref] [PubMed]
- Kuluöztürk M, Deveci F. The Glasgow prognostic score can be a predictor of mortality in acute exacerbation of chronic obstructive pulmonary disease. Expert Rev Respir Med 2020;14:521-5. [Crossref] [PubMed]
- Mekanimitdee P, Morasert T, Patumanond J, et al. The MAGENTA model for individual prediction of in-hospital mortality in chronic obstructive pulmonary disease with acute exacerbation in resource-limited countries: A development study. PLoS One 2021;16:e0256866. [Crossref] [PubMed]
- Gayaf M, Karadeniz G, Güldaval F, et al. Which one is superior in predicting 30 and 90 days mortality after COPD exacerbation: DECAF, CURB-65, PSI, BAP-65, PLR, NLR. Expert Rev Respir Med 2021;15:845-51. [Crossref] [PubMed]
- Anderson J, Hoang T, Hay K, et al. Evaluation of inpatient oxygen therapy in hypercapnic chronic obstructive pulmonary disease. Intern Med J 2021;51:654-9. [Crossref] [PubMed]
- Hinke CF, Jörres RA, Alter P, et al. Prognostic Value of Oxygenated Hemoglobin Assessed during Acute Exacerbations of Chronic Pulmonary Disease. Respiration 2021;100:387-94. [Crossref] [PubMed]
- Young PJ, Frei D. Oxygen therapy for critically Ill and post-operative patients. J Anesth 2021;35:928-38. [Crossref] [PubMed]
- Angus DC. Oxygen Therapy for the Critically Ill. N Engl J Med 2020;382:1054-6. [Crossref] [PubMed]
- Abdo WF, Heunks LM. Oxygen-induced hypercapnia in COPD: myths and facts. Crit Care 2012;16:323. [Crossref] [PubMed]
- Sarkar M, Madabhavi I, Kadakol N. Oxygen-induced hypercapnia: physiological mechanisms and clinical implications. Monaldi Arch Chest Dis 2022;
- van den Boom W, Hoy M, Sankaran J, et al. The Search for Optimal Oxygen Saturation Targets in Critically Ill Patients: Observational Data From Large ICU Databases. Chest 2020;157:566-73. [Crossref] [PubMed]
- Gottlieb J. Oxygen therapy in intensive care. Dtsch Med Wochenschr 2023;148:759-66. [Crossref] [PubMed]