The role of bronchoscopy in the diagnosis of early lung cancer: a review
Introduction
Lung cancer is the leading cause of cancer-related mortality worldwide (1). Despite improvements in diagnosis, staging and treatment, the overall 5-year survival for lung cancer is still low (range, 10–20%), due to the very poor prognosis of many patients diagnosed at advanced stage. On the other hand, patients with early stage lung cancer have a 5-year survival of more than 70%, thus screening and detection of early lung cancer is crucial to improve survival (2). Screening with low dose computed tomography (LDCT) have been shown to reduce the mortality by 20% in the high-risk population even if it is not sensitive enough to detect small tumours arising in the central airway (3,4). As shown by Rivera, flexible bronchoscopy became the recommended procedure for all patient suspected of having lung cancer, showing a sensitivity for central airway lesions of 88% and overall sensitivity for all modalities in the diagnosis of peripheral disease of 78% (it varied from 36% to 88%, according to the biopsy method used) (5). Furthermore, it allows the correct surgical planning through the evaluation of the surface, site and extent of the tumour, vocal cord motility and airway lumen (6).
Nevertheless, early cancers and precancerous lesions, such as angiogenic squamous dysplasia (ASD) or squamous cell carcinoma (SqCC) in situ (CIS) can be missed when examined with conventional white light bronchoscopy (WLB) alone (7,8). Two studies reported that only 29% of CIS and 69% of microinvasive tumours were detectable by an experienced bronchoscopist using WLB alone (9,10).
Considering that early detection followed by prompt treatment can improve the outcome for patients with lung cancer, great efforts have been made to overcome these limitations and innovative bronchoscopic techniques capable of detecting these pre-invasive malignancies were developed, such as autofluorescence bronchoscopy (AFB), narrow band imaging (NBI) and high magnification bronchovideoscopy (HMB). Unfortunately, the majority of lesions found on computed tomography (CT) are beyond the scope of examination, occupying the outer two-thirds of the bronchial tree (11). Hence, new probe-based technologies have been introduced: radial endobronchial ultrasound (R-EBUS), optical coherence tomography (OCT), confocal laser endomicroscopy (CLE) and laser Raman spectroscopy (LRS). In the following sections, we report the most important and interesting articles in the field which assess the value of the various techniques based on the evidences published to date.
AFB
AFB exploits the fluorescence recorded in normal bronchial tissue, dysplasia and CIS (12). Indeed normal tissue contains endogenous fluorophores, such as tryptophan, collagen, elastin and porphyrins, which absorb and emit green fluorescence when illuminated by violet or blue light. Alternatively, abnormal areas, containing a different concentration of the fluorophores, emit red, purple or magenta light (Figure 1). In addition to single and multicentre studies, three meta-analyses demonstrated that AFB and AFB + WLB have more advantages than WLB alone for detection of lung cancer and precancerous lesions, showing a higher sensitivity but a lower specificity than WLB alone (13-16). Sun et al. reported that the pool relative sensitivity of WLB + AFB versus WLB alone was 2.04 for detecting intraepithelial lesions and 1.15 for invasive cancer, whereas the pool relative specificity of WLB + AFB versus WLB was 0.65 (13). Indeed AFB provides many false positives (such as inflammation, infection or trauma), due to the high blood flow and metabolism that increase light absorption, diminishing green autofluorescence in tissue.
Several types of AFB system have been designed and developed. Laser-induced fluorescence endoscopy (LIFE) system (Xillix Technologies, Vancouver, Canada), consists of a helium-cadmium laser (wavelength 442 nm) that produces a blue-light. A multicentre clinical trial found that the sensitivity of LIFE + WLB versus WLB alone was 6.3 for intraepithelial neoplastic lesions and 2.71 including invasive carcinomas. Thus, the addition of LIFE to WLB increased the detection of early intraepithelial neoplastic lesions that would be missed using WLB alone (9). In view of these results, Tremblay et al. hypothesized to use AFB (Onco-LIFE system) in addition to LDCT for lung cancer screening, but only one case of CIS and one CT occult carcinoid tumor were detected by bronchoscopic procedure, for a prevalence of 2/1,300 or 0.15% (95% confidence interval, 0.0–0.6%). Hence, the study failed to show a significant increased benefit of AFB, that, to date, cannot be justified in a screening program (17).
Three more AFB imaging systems have been designed: SAFE 1000 system (Pentax; Asahi Optical, Tokyo, Japan) used as an excitation light source instead of laser light; SAFE 3000 system (Pentax; HOYA Corp., Tokyo, Japan), able to use both a xenon lamp and a monochromatic diode laser at the same time; and autofluorescence imaging (AFI) bronchovideoscope system (Olympus Optical Corporation, Tokyo, Japan). The AFI system incorporates three signals: autofluorescence, reflected green and red light. Chiyo was the first author to study the role of AFI for the early detection of preinvasive bronchial lesions, comparing its diagnostic performance to that of WLB and LIFE. The sensitivities for dysplasia by AFI, LIFE and WLB were 80%, 96.7% and 53.3%, respectively, while the specificities were 83.3% for AFI, 36.6% for LIFE and 50% for WLB (18). Seven more studies evaluated the effectiveness of AFI: six studies found its sensitivity significantly higher than that of WLB, whereas the remaining study recorded the same value for AFI and WLB. Regarding the specificities of AFI compared to that of WLB, no univocal data were available (19) (Figure 1).
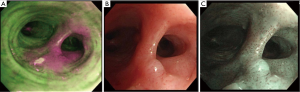
In view of these results and the effectiveness of AFI to evaluate abnormal bronchial mucosa, some authors investigated the role of AFB as a helpful tool to detect potential post-surgical complication as well. Indeed, a Japanese study showed the usefulness of AFI for detection of latent airway ischemic damage in pigs that had undergone tracheal autotransplantation, allowing early treatment of the anastomotic site and preventing stricture formation (20). Lastly, AFB may be a recommended procedure in addition with WLB for follow-up in patients with known severe dysplasia or CIS in the central airway (21), helping to guide surgical resection range when necessary, and reducing postoperative recurrence rate.
NBI
NBI is an image-enhanced endoscopy technology, able to enhance visualization of endobronchial microvasculature architecture when the tissue is irradiated by the two narrow wavebands of light: blue narrow band (390–445 nm), absorbed by surface mucosal layer capillaries and green narrow band (530–550 nm), absorbed by the hemoglobin in the deeper submucosal thick blood vessels (22). On the basis of these properties and the vascular network of the bronchial wall, NBI allows the differentiation of ASD, CIS, micro-invasive tumour and invasive SqCC (“Shibuya’s descriptors”) (23) and facilitates discrimination between adenocarcinoma (dotted blood vessels) and squamous histology (tortuous blood vessels and abrupt-ending vessel pattern) (24), exploiting the so-called angiogenic switch that occurs during tumorigenesis (25) (Figure 2).
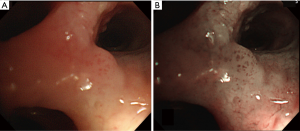
A recent and unique meta-analysis published by Iftikhar et al. (8 studies, n=632 patients), studied the diagnostic performance of NBI alone and combined NBI + AFI (4 studies, n=413 patients). The results suggested that NBI is better than AFI in the detection of premalignant airway lesions, showing a pooled sensitivity, specificity and the diagnostic odds ratio of 80%, 84% and 31.49%, respectively. Furthermore, data showed that the combination of AFI and NBI did not significantly improve the diagnostic performance, yielding a pooled sensitivity, specificity and the diagnostic odds ratio of 86%, 75% and 27.96%, respectively (22) (Figure 1). Finally, in addition to the ability of NBI to detect premalignant and invasive neoplastic lesions, it is also able to assess tumour extension and to guide therapeutic surgical strategy in central airway lesions (26).
In conclusion, although the primary role of NBI for detection of premalignant airway lesions and early lung cancer has been largely studied and confirmed, it remains to better define how NBI could potentially improve its diagnostic accuracy in combination with other bronchoscopic techniques such as HMB (24,27,28), endocytoscopy system (29) and CLE (30).
HMB
HMB is a direct-view WLB system able to observe vascular networks of the bronchial mucosa with a 55 to 110 times magnification power on a 14-inch video monitor, thanks to the combination of a video observation system for high magnification view and a fibre observation system for the bronchoscope tip’s orientation (31). It has an outer diameter of 6 mm and an observation depth of 1–3 mm.
Although several reports studied the use of HMB in gastrointestinal endoscopy, Shibuya was the first to publish a report to evaluate the usefulness of this procedure for the detection of bronchial dysplasia at site of abnormal fluorescence (LIFE system). In this study, the author found that HMB could detect dysplasia more accurately than fluorescence bronchoscopy alone, showing a sensitivity and a specificity of 71.4% and 90.9%, respectively. In particular, increased vessel growth and complex networks of tortuous vessels were assumed to be suggestive for bronchial dysplasia, and the vascular area ratio was significantly higher in premalignant lesions than in bronchitis (31).
Subsequently three studies (24,27,28) focused their attention on the clinical usefulness of NBI combined with HMB to detect premalignant bronchial lesions and early lung cancer on the basis of the vascular irregularities (Figure 3), showing a significantly increase in capillary blood vessel diameter from ASD to invasive SqCC. In particular they demonstrated that NBI + HMB was capable of detecting the onset of angiogenesis in the multi-step carcinogenesis of the lung, firstly in only bronchial dysplastic lesions established by fluorescence bronchoscopy (28), secondly by including CIS, microinvasive carcinoma and invasive carcinoma without using fluorescence techniques (24).
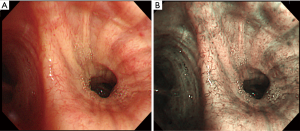
Finally, considering the fundamental role of angiogenesis in lung cancer and the tight relationship between smokers and lung cancer, Yamada et al. used HMB to evaluate the smoking influence on subepithelial microvessels in the large airway. The results suggested that vessel density was decreased in the smokers as compared to the non-smokers, concluding that smoking would affect not only lung parenchyma but also systemic angiogenesis (32).
OCT
OCT is a non-invasive, optical imaging technique based on the properties of light waves, with microscopic resolution (1–20 µm) and a penetration depth of maximum 2–3 mm that allows high-definition cross-sectional images of the airway luminal wall (33). Although the OCT system is analogous to that of B-mode ultrasound, the procedure requires no contact between the small optical probe and the tissue and it is able to delineate, in real time, cellular and extracellular structures at a resolution that is 20 times as high as that of ultrasound (34).
There are several clinical studies aimed at determining the effectiveness of OCT to differentiate between normal and pathological bronchial tissue, suggesting that OCT can be a well-tolerated, useful and effective tool in the early diagnosis of neoplastic lesions. Tsuboi et al. published the first report of endobronchial OCT for lung cancer in clinical practice (35). OCT findings showed layered structure in normal bronchi and high backscattering areas with loss of layered structure and glandular tissues in all lung tumors (35,36). In particular Hariri et al. tried to use the OCT technique to diagnose primary lung cancer (adenocarcinoma, SqCC and poorly differentiated carcinoma) in 82 ex-vivo tumour samples, according to the presence/lack of rounded or irregularly shaped and signal-intense nests. Although the results showed an overall average accuracy of 82.6%, with sensitivity and specificity ranges of 80.3–85.7% and 87–97.6% respectively, OCT could not replace tissue biopsy (37).
Since then, some authors have demonstrated the effectiveness of the OCT in combination with AFB to distinguish invasive cancer from CIS and dysplasia from metaplasia, hyperplasia, or normal tissue, using the quantitative measurement of the epithelial thickness (38,39). Furthermore Pahlevaninezhad et al. recently reported the combination of Doppler OCT with AFI in 31 patients to guide and improve biopsy of pulmonary nodules, resulting a powerful tool to evaluate pulmonary parenchyma as well (40). Finally, OCT has been described as an useful procedure also in other bronchoscopic settings, such as for real-time measurements of airway dimensions (41) and elastic airway wall remodeling in individuals with and without chronic obstructive pulmonary diseases (COPD), appearing more sensitive than CT scans (42,43).
In conclusion OCT is a non-invasive imaging method, feasible, safe, and capable of providing the microanatomy of the central airway both alone and in association with AFB, making it a promising tool for in vivo imaging of preneoplastic bronchial lesions and airway wall changes in patients with COPD. However, although the diagnostic yield of OCT is encouraging, it remains investigational and further studies are needed to determine sensibility and specificity of this technology in the evaluation of endobronchial or peripheral pulmonary lesions (PPLs) during routine diagnostic bronchoscopy.
CLE
Probe-based CLE (pCLE) is a new optical technology that permits real-time non-invasive histological imaging, using a laser with a wavelength of 488 nm directed into the alveolar space (44). The thin semiflexible probe can be introduced into the distal airway via the working channel of the bronchoscope and thanks to the lateral resolution of 3 µm and the depth of focus of 50 µm, it can be considered as a three dimensional endomicroscopy. CLE yielded informations exploiting the autofluorescence characteristics of elastic fibers present in the tracheobronchial tree (30,44), in the absence of the contrast agent normally used in gastrointestinal endoscopy.
Sorokina et al. recently reported the distinctive features of adenocarcinoma, SqCC and small-cell lung cancer in 18 lobectomy specimens. The pCLE images showed the differences among various subtypes of lung cancer according to the stromal and parenchymal component, allowing to identify lung carcinoma in ex-vivo samples (45). On the basis of these results, Wellikoff et al. described the pCLE changes for in-vivo diagnoses of lung cancer. He showed that the elastin composition was disrupted to varying degrees in relation to tumor differentiation, from mild in the lepidic-type to significant in poorly differentiated adenocarcinoma (46).
Some authors tried to use contrast agents such as fluorescein and acriflavine hydrochloride to improve the detection of epithelial bronchial cells during bronchoscopy in patients with suspected lung cancer (47,48). In particular Fuchs et al. demonstrated that the diagnostic value of acriflavine-aided CLE was comparable to the histology of lung biopsies, predicting the presence of neoplastic changes with a sensitivity of 96%, a specificity of 87.1% and an accuracy of 91% (48).
Finally, considering the ability of pCLE to detect not only elastin fibers but also blood vessels, alveolar macrophages and neutrophils, pCLE was used in the diagnostics of pulmonary alveolar proteinosis, invasive pulmonary aspergillosis and amiodarone-related pneumonia, revealing highly fluorescent complexes of lipo-proteinaceous/macrophages in the distal bronchioles and alveolar lumens (49). However, to date only a few reports are available on the real diagnostic yield of CLE and more ex-vivo and in-vivo studies are needed to validate the proposed data.
LRS
LRS is an experimental probe-system that provides a real-time in vivo diagnosis, collecting fingerprint-like spectral bands and light scattered from tissue with different molecular composition. Considering its ability to differentiate several biomarkers, LRS has been considered potentially helpful to improve the specificity of early lung cancer detection. Therefore, some authors started to use this technology in combination with AFB + WLB examination for reducing the high rate of false-positives obtained by AFB (14,50,51).
Indeed Short et al. demonstrated an improvement in the diagnostic accuracy for detecting lung cancer and preneoplastic lesions when LRS analysis was added to AFB + WLB imaging, showing a sensitivity and specificity of 96% and 91%, respectively (50). Furthermore, in view of the progressive growing interest in this diagnostic system, McGregor et al. recently reported that high grade dysplasia and malignant lung lesions could be differentiated from benign lesions and normal tissue using LRS with high sensitivity (90%) and good specificity (65%) (51).
To date, although the role of LRS seems to be growing, it still remains investigational and more studies are needed to validate its effectiveness in the diagnosis of early lung cancer.
EBUS
EBUS combines the bronchoscopic system and ultrasound to define mediastinal structures and parabronchial anatomy, in order to reduce biopsy errors during transbronchial sampling (52).
It is currently used in the staging of lung cancer, in the evaluation of endobronchial tumours, PPLs and mediastinal masses (53,54).
Two types of EBUS are currently used for clinical purposes: radial-probe EBUS (RP-EBUS) and convex-probe EBUS (CP-EBUS). RP-EBUS, first described in 1992, is an important tool in the evaluation of tracheo-bronchial wall structure; it is also used to drive transbronchial needle aspiration (TBNA) as well as to detect PPLs in combination with navigation bronchoscopy. CP-EBUS, first described in 2004, allows real-time TBNA of mediastinal and hilar lymph nodes (55).
The correct assessment of bronchial wall involvement by the tumour is necessary in order to evaluate the best treatment for each patient, considering photodynamic therapy (PDT) as an alternative therapy for patients with central type early stage lung cancer and surgery or radio-chemotherapy if the tumour extends beyond the cartilaginous layer (56) (Figure 4).
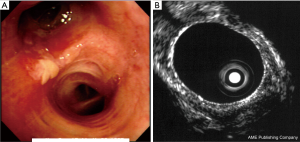
RP-EBUS is a 20 MHz rotating probe that is passed through the working channel of a standard bronchoscope, capable of producing 360° images with a high spatial resolution (less than 1 mm) and a penetration depth of 5 cm. By using the balloon sheath in combination with the RP-EBUS, the multilayer-structure of the central airway can be identified. Furthermore this technology is able to detect the compression/infiltration degree of lung cancer and the relationships between the tumour and adjacent structures, such as lymph node or vessels (57,58).
Indeed Kurimoto et al. showed in a prospective study, a 95.8% correlation between EBUS and histopathological findings in the determination of the depth of tumour invasion (59).
Based on these results, Miyazu et al. evaluated the depth of tumour invasion by EBUS to drive the best therapy in eighteen patients with SqCC. Nine patients had intra-cartilaginous tumours identified by EBUS and underwent PDT; the remaining nine patients had extra-cartilaginous tumours and were treated with surgical resection (six patients) and chemo-radiotherapy (three patients). Using EBUS, long-term complete remission was achieved in the PDT-treated group (60). Similar results were obtained in a recent study by Li et al. who detected the depth of tumour extension in 30 patients. Sensitivity, specificity and accuracy of RP-EBUS in evaluating tracheobronchial wall involvement were 88.89%, 100% and 90%, respectively (61). Finally EBUS improved the specificity (from 50% to 90%) for predicting lung cancer in small lesions detected by AFB but invisible on WLB (62).
Conclusions
Early detection of lung cancer in the airway is crucial for avoiding the multi-step progression to invasive cancer. Although tissue biopsy is the gold standard for diagnosing neoplastic and preneoplastic disease, bronchoscopic technologies are the safest and most accurate tools to evaluate both central and distal airway mucosa. To date, most of the evidence recommends the use of AFB and NBI for central airway evaluation and screening in patients with suspected/known lung cancer, exploiting the high sensitivity of these endoscopic procedures. However, considering the low specificity and the limitation to detect lesions only in the proximal bronchial tree, other techniques are suggested in combination with the latter, such as OCT, CLE and LRS, although more ex-vivo and in-vivo studies are needed to validate data and image interpretation criteria. In the near future, these technologies may enable bronchoscopists to obtain an “optical biopsy” of bronchial structures, providing high resolution and three-dimensional virtual images. These technologies may revolutionize the diagnostic and therapeutic assessment of airway disease. As Dr. Shigeto Ikeda said: “There is more hope with the bronchoscope”.
Acknowledgements
None.
Footnote
Conflicts of Interest: The authors have no conflicts of interest to declare.
References
- Siegel R, Ward E, Brawley O, et al. Cancer statistics, 2011: the impact of eliminating socioeconomic and racial disparities on premature cancer deaths. CA Cancer J Clin 2011;61:212-36. [Crossref] [PubMed]
- Allemani C, Weir HK, Carreira H, et al. Global surveillance of cancer survival 1995-2009: analysis of individual data for 25,676,887 patients from 279 population-based registries in 67 countries (CONCORD-2). Lancet 2015;385:977-1010. [Crossref] [PubMed]
- National Lung Screening Trial Research Team, Aberle DR, Adams AM, et al. Reduced lung-cancer mortality with low-dose computed tomographic screening. N Engl J Med 2011;365:395-409. [Crossref] [PubMed]
- Yasufuku K. Early diagnosis of lung cancer. In: Mehta A. editor. Clinics in chest medicine. Interventional Pulmonology 2010;31:40-7.
- Rivera MP, Mehta AC, Wahidi MM. Establishing the diagnosis of lung cancer: Diagnosis and management of lung cancer, 3rd ed: American College of Chest Physicians evidence-based clinical practice guidelines. Chest 2013;143:e142S-65S.
- Andolfi M, Vaccarili M, Crisci R, et al. Management of tracheal chondrosarcoma almost completely obstructing the airway: a case report. J Cardiothorac Surg 2016;11:101. [Crossref] [PubMed]
- Wahidi MM, Herth FJ, Ernst A. State of the art: interventional pulmonology. Chest 2007;131:261-74. [Crossref] [PubMed]
- Ikeda N, Hayashi A, Iwasaki K, et al. Comprehensive diagnostic bronchoscopy of central type early stage lung cancer. Lung Cancer 2007;56:295-302. [Crossref] [PubMed]
- Piehler JM, Taylor WF. Roentgenographically occult lung cancer: pathologic findings and frequency of multicentricity during a 10-year period. Mayo Clin Proc 1984;59:453-66. [Crossref] [PubMed]
- Lam S, Kennedy T, Unger M, et al. Localization of bronchial intraepithelial neoplastic lesions by fluorescence bronchoscopy. Chest 1998;113:696-702. [Crossref] [PubMed]
- Bose S, Ghatol A, Eberlein M, et al. Ultrathin bronchoscopy in the diagnosis of peripheral cavitary lung lesions. J Bronchology Interv Pulmonol 2013;20:167-70. [Crossref] [PubMed]
- Hung J, Lam S, LeRiche JC, et al. Autofluorescence of normal and malignant bronchial tissue. Lasers Surg Med 1991;11:99-105. [Crossref] [PubMed]
- Sun J, Garfield DH, Lam B, et al. The value of autofluorescence bronchoscopy combined with white light bronchoscopy compared with white light alone in the diagnosis of intraepithelial neoplasia and invasive lung cancer: a meta-analysis. J Thorac Oncol 2011;6:1336-44. [Crossref] [PubMed]
- Chen W, Gao X, Tian Q, et al. A comparison of autofluorescence bronchoscopy and white light bronchoscopy in detection of lung cancer and preneoplastic lesions: a meta-analysis. Lung Cancer 2011;73:183-8. [Crossref] [PubMed]
- Wang Y, Wang Q, Feng J, et al. Comparison of autofluorescence imaging bronchoscopy and white light bronchoscopy for detection of lung cancers and precancerous lesions. Patient Prefer Adherence 2013;7:621-31. [PubMed]
- Liu Z, Zhang Y, Li YP, et al. Clinical relevance of using autofluorescence bronchoscopy and white light bronchoscopy in different types of airway lesions. J Cancer Res Ther 2016;12:69-72. [Crossref] [PubMed]
- Tremblay A, Taghizadeh N, McWilliams AM, et al. Low Prevalence of High-Grade Lesions Detected With Autofluorescence Bronchoscopy in the Setting of Lung Cancer Screening in the Pan-Canadian Lung Cancer Screening Study. Chest 2016;150:1015-22. [Crossref] [PubMed]
- Chiyo M, Shibuya K, Hoshino H, et al. Effective detection of bronchial preinvasive lesions by a new autofluorescence imaging bronchovideoscope system. Lung Cancer 2005;48:307-13. [Crossref] [PubMed]
- He Q, Wang Q, Wu Q, et al. Value of autofluorescence imaging videobronchoscopy in detecting lung cancers and precancerous lesions: a review. Respir Care 2013;58:2150-9. [Crossref] [PubMed]
- Iga N, Oto T, Okada M, et al. Detection of airway ischaemic damage after lung transplantation by using autofluorescence imaging bronchoscopy. Eur J Cardiothorac Surg 2014;45:509-13. [Crossref] [PubMed]
- Houston KA, Henley SJ, Li J, et al. Patterns in lung cancer incidence rates and trends by histologic type in the United States, 2004-2009. Lung Cancer 2014;86:22-8. [Crossref] [PubMed]
- Iftikhar IH, Musani AI. Narrow-band imaging bronchoscopy in the detection of premalignant airway lesions: a meta-analysis of diagnostic test accuracy. Ther Adv Respir Dis 2015;9:207-16. [Crossref] [PubMed]
- Shibuya K, Nakajima T, Fujiwara T, et al. Narrow band imaging with high-resolution bronchovideoscopy: a new approach for visualizing angiogenesis in squamous cell carcinoma of the lung. Lung Cancer 2010;69:194-202. [Crossref] [PubMed]
- Zaric B, Perin B, Stojsic V, et al. Relation between vascular patterns visualized by Narrow Band Imaging (NBI) videobronchoscopy and histological type of lung cancer. Med Oncol 2013;30:374. [Crossref] [PubMed]
- Hanahan D, Folkman J. Patterns and emerging mechanisms of the angiogenic switch during tumorigenesis. Cell 1996;86:353-64. [Crossref] [PubMed]
- Li M, Izumo T, Zhang G, et al. Narrow-band imaging bronchoscopy improves assessment of tumor extent and affects therapeutic strategy for central lung cancer. Zhonghua Yi Xue Za Zhi 2014;94:3497-500. [PubMed]
- Yamada G, Kitamura Y, Kitada J, et al. Increased microcirculation in subepithelial invasion of lung cancer. Intern Med 2011;50:839-43. [Crossref] [PubMed]
- Shibuya K, Hoshino H, Chiyo M, et al. High magnification bronchovideoscopy combined with narrow band imaging could detect capillary loops of angiogenic squamous dysplasia in heavy smokers at high risk for lung cancer. Thorax 2003;58:989-95. [Crossref] [PubMed]
- Shibuya K, Fujiwara T, Yasufuku K, et al. In vivo microscopic imaging of the bronchial mucosa using an endo-cytoscopy system. Lung Cancer 2011;72:184-90. [Crossref] [PubMed]
- Thiberville L, Moreno-Swirc S, Vercauteren T, et al. In vivo imaging of the bronchial wall microstructure using fibered confocal fluorescence microscopy. Am J Respir Crit Care Med 2007;175:22-31. [Crossref] [PubMed]
- Shibuya K, Hoshino H, Chiyo M, et al. Subepithelial vascular patterns in bronchial dysplasias using a high magnification bronchovideoscope. Thorax 2002;57:902-7. [Crossref] [PubMed]
- Yamada G, Shijubo N, Kitada J, et al. Decreased subepithelial microvasculature observed by high magnification bronchovideoscope in the large airways of smokers. Intern Med 2008;47:1579-83. [Crossref] [PubMed]
- Ohtani K, Lee AM, Lam S. Frontiers in bronchoscopic imaging. Respirology 2012;17:261-9. [Crossref] [PubMed]
- Han S, El-Abbadi NH, Hanna N, et al. Evaluation of tracheal imaging by optical coherence tomography. Respiration 2005;72:537-41. [Crossref] [PubMed]
- Tsuboi M, Hayashi A, Ikeda N, et al. Optical coherence tomography in the diagnosis of bronchial lesions. Lung Cancer 2005;49:387-94. [Crossref] [PubMed]
- Michel RG, Kinasewitz GT, Fung KM, et al. Optical coherence tomography as an adjunct to flexible bronchoscopy in the diagnosis of lung cancer: a pilot study. Chest 2010;138:984-8. [Crossref] [PubMed]
- Hariri LP, Mino-Kenudson M, Lanuti M, et al. Diagnosing lung carcinomas with optical coherence tomography. Ann Am Thorac Soc 2015;12:193-201. [Crossref] [PubMed]
- Lam S, Standish B, Baldwin C, et al. In vivo optical coherence tomography imaging of preinvasive bronchial lesions. Clin Cancer Res 2008;14:2006-11. [Crossref] [PubMed]
- Pahlevaninezhad H, Lee AM, Lam S, et al. Coregistered autofluorescence-optical coherence tomography imaging of human lung sections. J Biomed Opt 2014;19:36022. [Crossref] [PubMed]
- Pahlevaninezhad H, Lee AM, Ritchie A, et al. Endoscopic Doppler optical coherence tomography and autofluorescence imaging of peripheral pulmonary nodules and vasculature. Biomed Opt Express 2015;6:4191-9. [Crossref] [PubMed]
- Williamson JP, Armstrong JJ, McLaughlin RA, et al. Measuring airway dimensions during bronchoscopy using anatomical optical coherence tomography. Eur Respir J 2010;35:34-41. [Crossref] [PubMed]
- Williamson JP, McLaughlin RA, Noffsinger WJ, et al. Elastic properties of the central airways in obstructive lung diseases measured using anatomical optical coherence tomography. Am J Respir Crit Care Med 2011;183:612-9. [Crossref] [PubMed]
- Coxson HO, Quiney B, Sin DD, et al. Airway wall thickness assessed using computed tomography and optical coherence tomography. Am J Respir Crit Care Med 2008;177:1201-6. [Crossref] [PubMed]
- Thiberville L, Salaün M, Lachkar S, et al. Human in vivo fluorescence microimaging of the alveolar ducts and sacs during bronchoscopy. Eur Respir J 2009;33:974-85. [Crossref] [PubMed]
- Sorokina A, Danilevskaya O, Averyanov A, et al. Comparative study of ex vivo probe-based confocal laser endomicroscopy and light microscopy in lung cancer diagnostics. Respirology 2014;19:907-13. [Crossref] [PubMed]
- Wellikoff AS, Holladay RC, Downie GH, et al. Comparison of in vivo probe-based confocal laser endomicroscopy with histopathology in lung cancer: A move toward optical biopsy. Respirology 2015;20:967-74. [Crossref] [PubMed]
- Fuchs FS, Zirlik S, Hildner K, et al. Fluorescein-aided confocal laser endomicroscopy of the lung. Respiration 2011;81:32-8. [Crossref] [PubMed]
- Fuchs FS, Zirlik S, Hildner K, et al. Confocal laser endomicroscopy for diagnosing lung cancer in vivo. Eur Respir J 2013;41:1401-8. [Crossref] [PubMed]
- Danilevskaya O, Averyanov A, Lesnyak V, et al. Confocal laser endomicroscopy for diagnosis and monitoring of pulmonary alveolar proteinosis. J Bronchology Interv Pulmonol 2015;22:33-40. [Crossref] [PubMed]
- Short MA, Lam S, McWilliams AM, et al. Using laser Raman spectroscopy to reduce false positives of autofluorescence bronchoscopies: a pilot study. J Thorac Oncol 2011;6:1206-14. [Crossref] [PubMed]
- McGregor HC, Short MA, McWilliams A, et al. Real-time endoscopic Raman spectroscopy for in vivo early lung cancer detection. J Biophotonics 2016. [Epub ahead of print]. [Crossref] [PubMed]
- Jiang J, Browning R, Lechtzin N, et al. TBNA with and without EBUS: a comparative efficacy study for the diagnosis and staging of lung cancer. J Thorac Dis 2014;6:416-20. [PubMed]
- Yasufuku K, Chiyo M, Sekine Y, et al. Real-time endobronchial ultrasound-guided transbronchial needle aspiration of mediastinal and hilar lymph nodes. Chest 2004;126:122-8. [Crossref] [PubMed]
- Yasufuku K, Chiyo M, Koh E, et al. Endobronchial ultrasound guided transbronchial needle aspiration for staging of lung cancer. Lung Cancer 2005;50:347-54. [Crossref] [PubMed]
- Yasufuku K. Current clinical applications of endobronchial ultrasound. Expert Rev Respir Med 2010;4:491-8. [Crossref] [PubMed]
- Ikeda N, Usuda J, Kato H, et al. New aspects of photodynamic therapy for central type early stage lung cancer. Lasers Surg Med 2011;43:749-54. [Crossref] [PubMed]
- Czarnecka K, Yasufuku K. Interventional pulmonology: focus on pulmonary diagnostics. Respirology 2013;18:47-60. [Crossref] [PubMed]
- Leong S, Shaipanich T, Lam S, et al. Diagnostic bronchoscopy--current and future perspectives. J Thorac Dis 2013;5 Suppl 5:S498-510. [PubMed]
- Kurimoto N, Murayama M, Yoshioka S, et al. Assessment of usefulness of endobronchial ultrasonography in determination of depth of tracheobronchial tumor invasion. Chest 1999;115:1500-6. [Crossref] [PubMed]
- Miyazu Y, Miyazawa T, Kurimoto N, et al. Endobronchial ultrasonography in the assessment of centrally located early-stage lung cancer before photodynamic therapy. Am J Respir Crit Care Med 2002;165:832-7. [Crossref] [PubMed]
- Li J, Chen PP, Huang Y, et al. Radial probe endobronchial ultrasound scanning assessing invasive depth of central lesions in tracheobronchial wall. Chin Med J (Engl) 2012;125:3008-14. [PubMed]
- Herth FJ, Becker HD. EBUS for early lung cancer detection. J Bronchol 2003;10:249. [Crossref]