Clinical management of chronic obstructive pulmonary disease patients with muscle dysfunction
Introduction
Chronic obstructive pulmonary disease (COPD) is a complex and highly prevalent disorder. Its clinical presentation is very heterogeneous being characterized not only by pulmonary involvement but by many different systemic manifestations and comorbidities (1-3). Probably one of the most common and best studied systemic manifestations of COPD is muscle dysfunction, which is also frequently associated with nutritional abnormalities. Muscle dysfunction affects both respiratory and limb muscles, having important consequences on the quality of life and prognosis of COPD patients (4,5). In a previous review different physiological and pathophysiological aspects related to COPD muscle dysfunction, as well as those factors and mechanisms involved in its occurrence were analyzed in depth (6). The present review aims to describe the diagnostic methods most frequently employed in the clinical setting as well as those treatments that can be used for muscle dysfunction in COPD patients.
Respiratory and limb muscle dysfunction
Muscle dysfunction occurring in COPD patients can involve muscles from different parts of the body. However, those involved in ventilation and those located in the lower limbs have particular importance due to their clinical implications. Among the former, the diaphragm, external intercostals and parasternal muscles act predominantly in inspiration, generating a more negative pleural and alveolar pressure with their contraction and allowing the air to enter into the lungs (6,7). Under normal circumstances, the relaxation of inspiratory muscles is sufficient to cause expiration, but when an extra expulsive effort is needed another muscle group is recruited (expiratory muscles). This happens in different circumstances, being specially relevant in the advanced stages of COPD, especially during exercise and exacerbations (6-9). The most important expiratory muscles are those located in the abdominal wall and the internal intercostals (6,7). In addition, many other muscles of the upper chest and shoulder girdle can also contribute to the breathing effort (6,10,11). In turn, limb muscles are essential for ambulation and other important activities of daily life, and therefore, their functional loss will entail significant limitations to patient physical activity and social life, impacting negatively on quality of life (5,6).
Muscle dysfunction involves the loss of strength (i.e. the ability to develop a maximal effort) and/or endurance (i.e., ability to maintain a submaximal effort through time) (6). These functional impairments generate two different pathophysiological situations: weakness, which is a relatively stable muscle malfunction that can only be reversed with long-term measures; and fatigue, defined as a temporary muscle dysfunction that disappears with rest (6). Both may, or may not, be present together in the same patient. Muscle dysfunction is highly prevalent among COPD patients (12-15), and appears to be caused by multiple factors, although lung hyperinflation seems to play the most important role for respiratory muscle deterioration (6,16), whereas deconditioning, resulting mainly from reduction in physical activity, is critical to limb muscle impairment (5,17). In both muscle groups, different systemic factors such as smoking, nutritional disorders, systemic inflammation, hypoxemia, hypercapnia/acidosis and some drugs such as steroids, which are harmful for skeletal muscles, also play an important role (5,6,17). Respiratory muscle dysfunction aggravates the already unfavorable situation caused by changes occurring in the airways and lung parenchyma, negatively influences symptoms and participates in exercise limitation (6,18). Fortunately, the diaphragm and other respiratory muscles undergo a process of functional and structural adaptation, which partially counterbalances the effects of the above mentioned negative factors (13,19-21). This adaptive process is probably the result of the overactivity of these muscles in COPD patients, which probably mimics a “training effect” (6,22). This circumstance is radically different to that experienced by the lower limb muscles, which also show a deterioration in their functional properties (4-6,23), but in the absence of any sign of adaptation (24-29). As will be seen in detail later, limb muscle dysfunction has a great impact on the patient’s life (5,6,30). It is interesting to note that a maintenance of the normal phenotype and even some adaptive phenomena have been observed in the upper limb muscles, probably because of both their occasional participation in the ventilatory effort and a better preservation of their activity than that of lower limb muscles (31-33). This in addition, results in a less pronounced functional impairment (5,6).
Clinical manifestations of muscle dysfunction
Respiratory muscles
As previously mentioned, respiratory muscle function is most probably the result of the balance between multiple deleterious factors and a “training effect” resulting from enhanced activity due to the lung disease. The resulting phenotype is more efficient than that of limb muscles but still implies a significant functional limitation. Thus, many COPD patients actually show a decrease in the strength and/or endurance of their respiratory muscles (12,14,17,34,35). This circumstance is relatively common (20–45%) in patients with a stable disease (12,14,36), reaching 80–90% in those with multiple hospital admissions due to exacerbations (36). Respiratory muscle dysfunction is associated with increased dyspnea (6,18), also conditioning a worse ventilatory response to both exercise and exacerbations (18,37,38). As a result, daily physical activity decreases, quality of life deteriorates and exacerbations and hospitalizations increase. Moreover, the latter are prolonged, involve special care after discharge (38), with a higher risk of a hospital readmission (36,39), and a worse prognosis (40,41). In more severe cases, complete respiratory muscle failure may occur and the patient will require mechanical support to maintain ventilation (see next sections). In addition, in those patients requiring invasive mechanical ventilation (IMV), respiratory muscle dysfunction can dramatically hinder the process of weaning (42,43).
Respiratory muscle weakness can be present due to relatively stable negative circumstances such as poor nutritional status, hypoxemia, steroid myopathy or oxidative stress. However, this situation can also be impaired by intercurrent acute events such as exacerbations or intense exercise, which produce an increase in the ventilatory effort (44,45), adding a component of acute fatigue to muscle weakness. It is clinically relevant to differentiate these two phenomena because while therapeutic interventions for weakness require a medium-long-term interventions (e.g., nutritional supplements, training), fatigue requires mainly rest (i.e., ventilatory support).
Limb muscles
As already mentioned limb muscle dysfunction affects predominantly those muscles located in the lower limbs, where deconditioning phenomena are more obviously present. Limb muscle dysfunction is also very common, affecting 20–30% of COPD patients (15,46,47), with a quadriceps strength averaging 25% less than in healthy subjects (15). Moreover, the functional impairment of peripheral muscles is present even in the less severe stages of the lung disease (15). However, when the severity of COPD is not only classified by the degree of airway obstruction but through multidimensional indices such as BODE, it becomes directly related with the presence and intensity of muscle dysfunction (15).
As in the case of respiratory muscles, limb muscle dysfunction is characterized by the loss of strength and/or endurance (4,48). Most clinical laboratories only determine the former functional dimension due to the fact that the technical procedure is easier. However, it should be noted that the loss of endurance cannot always be predicted by the strength status (48), potentially leading to underdiagnosis of limb muscle dysfunction. Moreover, COPD patients show early muscle fatigue even with normal walking (49,50). Limb muscle dysfunction also has important consequences for the patient, because it contributes to exercise limitation and reduction of daily activities and social life, leading to a quality of life impairment, greater utilization of social and health resources and a worse prognosis (23,51-55). It is worth noting that besides the reduction in the level of physical activity and the perception of disability that this entails, patients frequently associate psychological symptoms such as anxiety and depression, which will further reduce their social life (56), generating a vicious circle that worsens their prognosis even more (57-59).
Another point to consider is that limb muscle dysfunction is often associated with a decreased body weight and more specifically with a reduced lean mass (i.e., muscle mass). In fact, COPD patients have on average a 20–25% decrease in their quadriceps muscle size (60). As a result of this circumstance, the clinical evaluation of COPD patients should include not only muscle functional assessment but also instruments to determine their nutritional status (anthropometry and techniques providing body composition).
Muscle dysfunction diagnosis
Respiratory muscles
The functional evaluation of respiratory muscles can be addressed with multiple techniques, both invasive and noninvasive, volitional or non-volitional, and more or less specific (Table 1 and Figure 1).
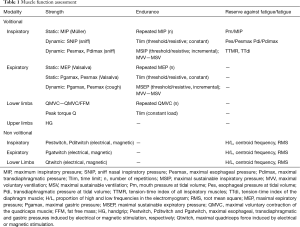
Full table
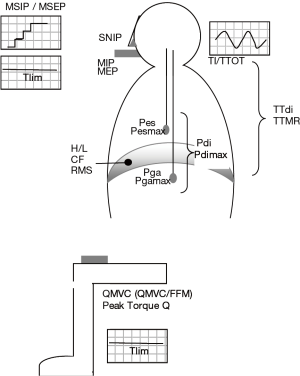
Clinical history and physical examination
Different symptoms and signs can suggest the presence of a functional problem in respiratory muscles. For example, a patient suffering from a level of dyspnea disproportionately high for the degree of lung function impairment, or a relevant worsening of this symptom in the supine position (61). Muscle dysfunction can also be suggested by a poor quality of sleep in the absence of sleep apnea syndrome. As already mentioned, difficulties in the weaning process in COPD patients requiring mechanical ventilation may also indicate respiratory muscle dysfunction (62). Tachypnea, is also suggestive, especially if the respiratory frequency is very high and is accompanied by shallow breathing. The use of accessory muscles for ventilation or the presence of signs of thoraco-abdominal or upper-lower chest (Hoover’s sign) incoordination is also important for diagnosis of respiratory muscle dysfunction. The same applies to difficulties to cough and eliminate secretions.
Imaging
Radiology and ultrasound techniques can be useful in the diagnosis of diaphragm paralysis, detecting the absence of movement in normal breathing or a paradoxical muscle movement during a sniff maneuvers (63). A detailed analysis of the echographic findings may also be useful in the early diagnosis of muscle fatigue (64,65). Moreover, some imaging techniques may even allow for an approximation to the metabolic status of the respiratory muscles in both COPD patients and healthy individuals (66,67).
Lung function
- Breathing pattern: A high respiratory rate (f) at rest, especially when accompanied by a low tidal volume (VT), indicates a ventilatory overeffort and the possibility of the development of muscle fatigue. The same accounts for an elongated inspiratory time (TI) or an increase in the ratio between the latter and the total time of the duty cycle (TTOT). In this regard, it has been shown that a TI/TTOT above 40% can only be held for a short time (68);
- Forced spirometry: Marked declines in forced vital capacity (FVC), with a rise in the FEV1/FVC ratio may be indicative of a respiratory muscle problem in COPD patients. The same can be applied to a 25% decrease in FVC when changing the sitting position for the supine position (69);
- Body plethysmography and transfer factor for carbon monoxide (DLco): Static lung volumes, such as total lung capacity (TLC) or the intrathoracic gas volume [ITGV, taken as a value representing functional residual capacity (FRC)] can be decreased for the same reasons as FVC. Similarly, DLco may be affected by respiratory muscle dysfunction, although the Krogh ratio (DLco/VA) remains stable;
- Arterial blood gases: In early stages of muscle dysfunction patients may show moderate hypoxemia and hypocapnia due to hyperventilation but later on gases will move to a marked hypoxemia and hypercapnia with a decreased alveolar-arterial gradient, especially at night (70,71).
Sleep studies
The use of a pulsioximeter and a capnograph can help in the early detection of alterations in blood gases, while the polysomnography would evidence a poor quality of sleep in the absence of apneas.
More specific functional techniques
The maximum force of respiratory muscles cannot be directly determined because of their location. It is assessed indirectly through the measurement of pressures that they generate. Such pressures can be obtained in different body locations and using different maneuvers.
- Maximum pressures at the mouth: This is the most commonly used clinical determination for respiratory muscle strength, being usually performed during a static maneuver (in the absence of airflow). The Müller maneuver, usually done from residual volume (RV), which can also be performed from FRC, is used to determine the maximum inspiratory pressure (MIP) (72). The Valsalva maneuver in turn, usually made from TLC, which can also be performed from FRC, is used to measure the maximum expiratory pressure (MEP) (72). Validated reference values exist for both MIP and MEP (72,73). It is usually considered that values below 65% of the reference or lower than 80 cmH2O indicate respiratory muscle dysfunction (4,74);
- Other pressures: It is also possible to determine the maximum pressures in dynamic conditions (while there is airflow). This is the case for the sniff nasal inspiratory pressure (SNIP), determined in the nostrils during a forced inhalation. This maneuver has been proposed as a good alternative to the static one for the evaluation of the inspiratory strength (75,76), and validated reference values are also available (77,78). Different authors consider that for Caucasians a SNIP lower than −70 cmH2O in men and −60 cmH2O in women indicate inspiratory muscle dysfunction (4). The simple and dynamic whistle maneuver has also been proposed to determine expiratory muscle strength (79), although its use is still limited and there are no reference equations. A point to be considered is that pressure determinations in the upper airways may not be valid if a problem such as inspiratory collapse or incoordination occurs at this anatomic level. Unfortunately, this is relatively frequent in COPD patients (80). Thus, it may be necessary to perform a more invasive technique with a catheter, determining the esophageal pressure that closely reflects pleural pressure (81). The maximal esophageal pressure (Pesmax) obtained during a sniff maneuver will express faithfully the overall strength of inspiratory muscles (82). A Pesmax higher than −80 cmH2O in men and −70 cmH2O in women discards inspiratory muscle weakness (82,83). Moreover, the ratio between mean esophageal pressure at tidal volume at VT and Pesmax (Pes/Pesmax) indicates the relative degree of effort that inspiratory muscles use in each inspiration and can be useful to anticipate fatigue. It is also possible to determine the specific pressure exerted by the diaphragm muscle. For this, another catheter should be placed in the stomach to obtain gastric pressure at tidal volume (Pga). The difference between esophageal and gastric pressures is known as transdiaphragmatic pressure at tidal volume (Pdi), and when this is determined during a forced inhalation it is called maximal transdiaphragmatic pressure (Pdimax), which reflects the strength of the diaphragm (84). While a Pdimax above 100 cmH2O in men and 70 cmH2O in women rule out diaphragmatic dysfunction, values lower than 75 cmH2O in the former and 50 cmH2O in the latter are highly suggestive of such alteration (4). Again, the Pdi/Pdimax ratio indicates the degree of the muscle effort in each ventilatory cycle, and values above 40% will be difficult to be sustained for a long time (68). Both Pesmax and Pdimax can also be determined during a static maneuver (Müller), but this method is less used. Finally, the maximal gastric pressure (Pgamax) can also be used to assess the specific strength of expiratory muscles from the abdominal wall (85). For this, a maximum dynamic (coughing) or static maneuver (Valsalva) should be performed (86,87). The strength of the expiratory muscles can also be evaluated through Pesmax obtained during an expiratory effort, but in this case the pressure obtained will reflect Pgamax plus the contribution of the expiratory muscles of the ribcage;
- Non-volitional (instrumental) techniques: A common limitation to all previously mentioned maneuvers is the need for a high patient compliance, which is not always present. To obviate this limitation electrical or magnetic stimulation (‘twitch’) techniques can be used. These methods directly activate centers and nerves involved in the action of different muscles. Electrical stimulation is more widespread, but has the disadvantage of being painful and poorly reproducible. In addition, it is difficult to achieve a supramaximal level of stimulation with this technique (88,89). By contrast, magnetic stimulation achieves better results and is better tolerated, so it will probably have a progressively broader use in the clinical setting (89-92). Approximatively normal values are available for diaphragm and quadriceps muscles with both stimulation modalities. These values are lower than those obtained with voluntary maneuvers. In the case of magnetic stimulation normal reference values have been established specifically for the technique performed at the cervical and phrenic levels (4,93,94). If Pdimax (twitch) is obtained by direct stimulation of the phrenic nerve, values below 15 cmH2O indicate a serious diaphragmatic dysfunction (4), although some authors consider that weakness already exists with values lower than 50−60 cmH2O in men and 35−40 cm H2O in women (4,95);
- Respiratory muscle endurance: Although the evaluation of muscle endurance is probably more relevant to clinical purposes than that of muscle strength, the tests designed for this purpose are much less widespread. Among the simplest tests, but with very little specificity, is the determination of maximal voluntary ventilation (MVV). For this, the subject should try to get the maximum ventilatory volume during a maneuver that usually lasts 15 seconds, the result being then extrapolated to 1 minute (L/min). Another simple but still unspecific alternative is to obtain the maximum sustainable ventilation (MSV), which is done at a percentage of VVM (usually 60−80%) (4). The more specific tests designed to determine the endurance of respiratory muscles are generally based on repeated maneuvers against incremental loads or a constant submaximal load (34,35,96-99). The outcome on the former modality is the maximal pressure that the patient can sustain for a specific time, being in fact a mixed surrogate of both endurance and strength. The outcome of the latter, which is probably a better reflection of endurance, is the time elicited sustaining a percentage of the maximum load until task failure (Tlim) (34,35,98,99). The loads or resistances, which can be either inspiratory or expiratory, can be applied through resistive or threshold devices. The second device will ensure a certain level of pressure to generate airflow (97,98). This is a better choice since resistive resistance require an strict control of the breathing pattern to ensure a target respiratory pressure (97). There are some approaches to the reference values for both inspiratory and expiratory endurance variables (99,100), but since they have not been unified each laboratory uses their own equations. Finally, it should be mentioned that endurance tests can also provide the metabolic consumption of respiratory muscles during the effort (101).
Closely related to the concept of muscle endurance is that of muscle reserve against fatigue, a progressive condition that can lead to task failure. Nowadays, only the concept of acute fatigue is widely accepted, with the classical theory of ‘chronic fatigue’ having been virtually abandoned. The reserve against fatigue can be evaluated from breathing pattern and respiratory pressures, obtaining the tension-time indices, which in turn may be calculated for all inspiratory muscles or specifically for the diaphragm (102). The former [tension-time index of all inspiratory muscles (TTMR)] is the result of the product between TI/TTOT and either mouth or esophageal pressure ratios (IP/MIP or Pes/Pesmax, respectively) (103). The tension-time index for the diaphragm in turn uses transdiaphragmatic pressures in the equation [tension-time index of the diaphragm muscle (TTdi) = (TI/TTOT) * (Pdi/Pdimax)] (68,84,102). Values close to 0.15 indicate a high risk of fatigue in the next 45 minutes, while values above this limit predict a faster ventilatory failure (68). A second method for detecting respiratory muscle fatigue is to determine the maximum relaxation rate (MRR) (104), which is obtained from the inspiratory pressure curve. Under experimental conditions fatigue can also be detected by a decrease in maximum respiratory pressure induced by stimulation (twitch) (105,106). Another method used for the diagnosis of fatigue, which is complex and restricted to specialized laboratories, is the analysis of the electromyographic signal, and more specifically the evaluation of the relationships between high and low frequencies, the centroid frequency and/or the root mean square (RMS) (107,108).
Limb muscles
Clinical history and physical examination
Muscle dysfunction should be suspected in COPD patients presenting symptoms in the lower limbs during exercise (109,110). Moreover, physical examination may reveal a loss of muscle mass at this level, a circumstance that negatively influences muscle strength.
Muscle mass
The impact of nutritional status is especially relevant in the limb muscles. Anthropometric measurements are the simplest way to detect an impairment in nutritional status. Both the percentage of the ideal body weight (% IBW) and the body mass index (BMI) are the most widely used variables. Values lower than 80−85% in IBW or 18−18.5 kg/m2 in BMI are the most widely accepted limits to define “low weight” (111,112). A more direct anthropometric approach to muscle mass are determinations of the triceps skinfold or thigh circumference, but both can be misleading and have fallen into disuse in recent decades (113,114). Much more specific is to determine the body composition, generally through either bioelectrical impedance or dual energy densitometry (DEXA) (115,116). The most useful parameter is the fat free mass index (FFMI), which defines a major loss of muscle mass with values under 16 kg/m2 for men and 15 kg/m2 for women (117). Both techniques are appropriate for COPD patients (5), but it should be noted that values obtained with DEXA are somewhat higher than those provided by impedance (114,118). Moreover, DEXA has an additional advantage since it can differentiate muscle mass from different body areas, allowing a specific assessment of limb muscles. This is important since regional muscle mass has a bad correlation with the overall body muscle mass. Different imaging techniques such as computed tomography, magnetic resonance imaging, positron emission tomography (PET) and ultrasound can also be useful to assess the muscle mass of specific areas in COPD patients (53,119-123).
Limb muscle strength
As previously mentioned determination of the maximum strength of a muscle is not the best clinical approach to the assessment of its function, but is simple and easily performed, also being the most common approach used for limbs. In this case, maximal force is usually evaluated through maximal isometric maneuvers, and is expressed in kilograms or Newtons. The handgrip is the maneuver most often used for the upper limbs in the clinical environment (23). Reference values are available (124-126), and although the threshold for the diagnosis of muscle weakness is not clearly defined (127), several authors have proposed values below 80−85% pred. (128-130). In turn, quadriceps strength is usually determined to assess muscle function of the lower limbs, since this muscle is essential for ambulation. In this case there are also reference values, which are specific for different populations (15,54,131-133). Some authors propose that values of quadriceps strength should be normalized by the body weight or lean mass of the subject to better express the intrinsic force of the muscle (134,135). Other methods used to assess limb muscle strength are to determine the maximum weight that a subject is able to lift or their ability against a hydraulic resistance, but both are complex and not used in the clinical setting. Finally, a method that has a progressive acceptance is to measure the peak torque at fixed joint angle speeds using special dynamometers (134,136), with the results being expressed in newtonmeters (Nm) in this case.
Electrical or magnetic stimulation can be used in subjects unable to collaborate (137,138). The maximum muscle strength is obtained through a supramaximal stimulus of the corresponding peripheral nerve (138,139), and reference values have also been published (123,140). An alternative method is to administer progressive stimuli to get a force-frequency curve (5).
Limb muscle endurance
As in respiratory muscles, the determination of limb muscle endurance is much less widespread than that of strength. However, different techniques have been proposed, being generally based on the repetition of maneuvers against submaximal loads (10−80% according to different protocols) at a preset rate until task failure (48,141,142). Repeated maximal isometric maneuvers have also be employed (143-146). Unfortunately, there are no reference values for these methods to date (4,5), so each laboratory should develop its own equations or have at least a control group of healthy subjects to make comparisons. It is noteworthy that there are also methods based on changes in the electromyographic signal, but they are more cumbersome and only used in very specialized environments (5). In patients unable to collaborate electrical or magnetic repeated stimulation of the femoral nerve may be performed (4,143,144,146,147).
Treatment
There are many factors involved in muscle dysfunction, and therefore its treatment is necessarily multidimensional. Triggers should be avoided and those negative circumstances already established should be counterbalanced with pharmacological and nonpharmacological interventions (Table 2). Many of the latter measures are included in the general concept of Pulmonary Rehabilitation: abstinence from tobacco smoking, educational activities, a healthier lifestyle, training, etc. (148).
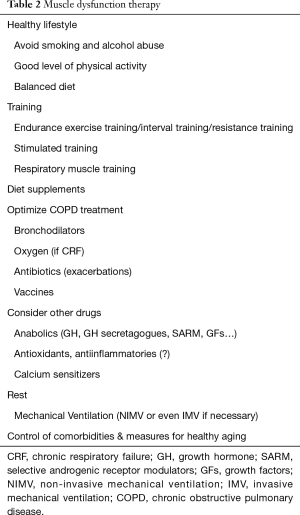
Full table
To stop tobacco smoking
This simple measure will target different objectives. It will improve the overall nutritional status of the patient by eliminating the anorectic effect of tobacco, while reducing oxidative stress, lung and systemic inflammation and the negative protein balance, which are characteristic of COPD patients (149). In addition, it will prevent other deleterious effects of tobacco on muscle function and structure (150).
To increase physical activity
Reduction in physical activity and subsequent deconditioning is a key factor for peripheral muscle dysfunction, generating a process where other deleterious cofactors also became involved. Therefore, this vicious circle must be broken by recommending an increase in physical activities that should be outdoors if possible (to avoid vitamin D deficiency), and treating depressive symptoms if necessary (151,152). With these measures, hospital admissions will decrease (58), and in selected groups of patients even the risk of death will be reduced (153). In addition, many of the structural and metabolic alterations shown by limb muscles (6) will diminish or even disappear with exercise (151,154). Recovering a good level of activity is also essential for respiratory muscles in patients who have undergone mechanical ventilation, especially those who have received controlled modalities. This is the only situation in which respiratory muscles of COPD have not been overactive but have suffered from inactivity and deconditioning (42,155).
Training
A good general training program improves cardiovascular and muscle function and increases exercise capacity although not substantially altering lung function variables (48,154,156-159). General training also reduces ventilatory and cardiocirculatory requirements, since the body muscles become more efficient in their use of the oxygen provided by these systems (148,160). Moreover, it also has psychosocial effects, improving symptoms of anxiety and depression (161,162). Training programs vary in their characteristics depending on the objectives: an overall improvement of exercise capacity, ameliorations in the strength, endurance or both in a particular muscle group, etc. General training has different modalities including endurance exercise training, interval training and resistance training, among others. The endurance exercise training is a crucial element of most rehabilitation programs designed for COPD patients. Its main objective is to improve the overall aerobic capacity of the subject. In other words, the ability to maintain a moderate level of exercise through time. Therefore, the objective is not only to achieve an improvement in muscle function but also to positively condition the cardiovascular system. For this, cycling or walking at a high intensity (more than 60% of the maximum tolerated load), 20−60 minutes, and 3 to 5 times per week is recommended (148). When this training modality has the right duration, it can result in an increase in muscle mass, strength and endurance (156,163-168). These improvements are also accompanied by increases in the exercise capacity, both at maximal and submaximal levels (148,154,169), and lower levels of dyspnea (148). There is a general agreement that an endurance exercise training program requires a minimum of 8 weeks to get these benefits (154,169-171). Some authors consider that the exercise intensity must also cause a high level of respiratory and general symptoms (172). However, it is also possible to use lower intensities of exercise or even an 'interval' training (interspersing periods of high and relatively low intensities) (148) in severe COPD patients, both obtaining benefits and reducing risks. The so-called “whole-body training” in turn combines different activities such as cycling, walking (both at ground and treadmill), weight lifting and gymnastic conventional exercises. Its objective is similar to the endurance exercise training, trying to improve in parallel the cardiocirculatory, ventilatory and muscle components.
Strength training and resistance training consist of heavy weight lifting or exercising against submaximal resistances, respectively, for specific muscle groups. The intensity of the loads and the pattern of repetitions determine that improvements would be in strength, endurance or both. In fact, the intensity seems to have more influence on strength, while the duration of the exercise has more impact on endurance (54,164,166,167,173,174). It is well known that loads lower than around 30% of the maximum muscle force have no significant effects, while those higher than 40% can already induce an improvement in endurance (a mainly aerobic property, which depends mainly on the muscle composition: fiber type percentages, capillary and mitochondrial densities, etc.). If loads reach 70% of the maximal force and are applied with few repetitions they can result in specific increases in strength (anaerobic activity that is mainly dependent on muscle size) (175). It has been demonstrated that strength training really gets improvements in muscle mass and maximal force in limb muscles of COPD patients (23,46). These improvements are higher than those obtained with the endurance exercise training (148), whose objectives and potential benefits are more general.
An important point is to take into consideration the right moment to initiate high intensity exercise in training programs, since it is known that an excessively intense exercise can cause muscle oxidative stress in untrained COPD patients (174). This negative effect does not occur in patients who have already achieved an acceptable level of conditioning (176). However, the level of exercise during training must exceed that of daily activities to achieve positive effects on muscle strength and/or endurance as well as on the cardiovascular system (148). The conclusion of all these findings is that the intensity of exercise should be sufficient enough to induce benefits, but established progressively to avoid adverse side-effects.
One training option is to focus on a particular portion of the body. This is the case of the programs of upper limb training (177,178). This modality is designed to improve the function of muscles of this location but also to get indirect benefits to ventilation because many of these muscles (especially the more proximal) also collaborate on breathing (179). In fact, this type of training has been occasionally shown to reduce dyspnea in COPD patients (178), although this is still controversial (40). Neuromuscular stimulation is another form of limb muscle training consisting in the instrumental induction of repeated muscle contractions. It is particularly appropriate for patients with very limited exercise capacity, which cannot follow volitional training modalities (148,180). The stimulation can be electrical or magnetic but there is still more extensive experience with the former. The stimulation is performed transcutaneously and can result in improvements in peripheral muscle function and exercise capacity in stable COPD patients (180-182), with its effects not being lower than those of conventional limb muscle training (183). Magnetic stimulation training, which is achieved by passing a high intensity electric current through a copper coil, generating a magnetic and relatively focal field of action (91), has been introduced in the last decade. It avoids some of the drawbacks of electrical stimulation, which can be painful and cause skin discomfort. The effects of magnetic stimulation training are similar to those of electrical stimulation (92,184,185), although a more extensive experience is still needed. As occurs with conventional training, it is also important to control the intensity (in this case amplitude), frequency and duration of the electrical or magnetic stimuli.
It is also possible to specifically train respiratory muscles (148). This training modality can be done by repeated maximum maneuvers, isocapnic hyperventilation or application of submaximal loads through resistive or threshold systems. Training of respiratory muscles with the latter type of loads has demonstrated improvements in their strength and endurance (35). This is clearly indicated in patients with moderate or severe disease, presenting dysfunction of these muscles or significant breathlessness during exercise (148).
Another interesting point to consider is whether to train patients during or immediately following exacerbations is beneficial or not. There is some evidence suggesting that to train during these acute episodes prevents loss of muscle function and exercise capacity after discharge (186). This training modality can be performed conventionally or by neuromuscular stimulation, depending on the actual condition of the patient (180,187).
Correction of nutritional abnormalities
Since the loss of body weight and muscle mass is an important factor in muscle dysfunction it must be corrected. Moreover, many of actions that are beneficial for the nutritional status also act directly on muscle function. This is the case of abstinence from tobacco smoking or increased physical activity. In addition, a healthy diet (188,189) and, in some cases, nutritional supplements or even anabolic drugs should be recommended (115,188,190-192). Moreover, there are some other nutritional elements which can also be of interest. This is the case of creatine, whose administration can improve exercise capacity in other populations (193), most likely by facilitating the sustained availability of energy by ATP resynthesis (5). However, creatine has not shown any clear effects in COPD patients (5). L-carnitine in turn, is necessary for fatty acid oxidation in mitochondria, and therefore to obtain energy. Its benefits are clear in athletes but are more modest in patients (5). In summary, with the mentioned improvements in lifestyle and dietary supplements, if necessary, it is possible to maintain a metabolic balance in many COPD patients. This, along with exercise, will facilitate the synthesis of structural proteins in the muscle, which is the basis for muscle function (192,194-196).
The use of anabolic drugs deserves a specific comment, given the relevance they may have for muscle function. This group includes androgens like testosterone and oxandrolone as well as selective androgenic receptor modulators (SARM). In general their effects are positive for muscle mass, but no clear benefit has been obtained on muscle function (5,197). In addition, except probably for some of SARM, their negative side effects are too relevant (115,198). Other anabolic drugs are those related to growth hormone (GH), which acts through the insulin-like growth factor 1 (IGF-1). As is the case of androgens, the administration of GH or its secretagogues (substances that induce release of endogenous GH from the pituitary gland) is able to increase muscle mass but without clear functional benefits (5,199,200). Finally, there are promising results with the administration of IGF-1, the mechano growth factor (MGF) and other growth factors in various chronic conditions that affect muscle mass (201-203), but there is still a lack of large studies in COPD patients.
Antiinflammatory drugs and antioxidants
Both systemic and muscle inflammation and oxidative stress appear to play an important role in either muscle dysfunction or nutritional deficiencies associated with COPD (5,12,40). Consequently, different biological agents, such as anti-cytokines and antioxidants, have been tested both in animal models and patients. However, the results of these studies have been negative or relatively modest (204-208).
Optimization of the general treatment of COPD
The use of bronchodilators, and probably of double bronchodilation, allows us to obtain different improvements in symptoms and lung function (reductions in airway obstruction, pulmonary hyperinflation and work of breathing) (3,209-213). This will facilitate the work of respiratory muscles contributing to a higher level of physical activity, which in turn will have beneficial effects on limb muscles. In addition, metabolic requirements will be lower promoting a better nutritional status. At present, there are wide studies that have been designed to assess the likely synergistic effect of an optimized bronchodilator therapy associated with training programs (209,214). Lung volume reduction by surgery or endoscopy may also have beneficial effects on respiratory muscle function by reducing hyperinflation and improving exercise capacity and daily physical activity of COPD patients (215-217). All these treatments and oxygen therapy, if necessary, will also reduce the level of hypoxemia and muscle hypoxia. This in turn will favour aerobic metabolism in different muscles (218,219), while decreasing competition between respiratory and peripheral muscles for oxygen supply during exercise (220). Moreover, correction of tissue hypoxia also reduces oxidative stress, a relevant deleterious factor for both nutritional status and muscle function. Since tissue hypoxia can coexist with relatively conserved levels of PaO2 or SaO2, the technique of near-infrared spectroscopy (NIRS) can be useful for monitoring the actual muscle oxygenation (5,221). Systemic steroids should be avoided or at least reduced when possible in most severe COPD patients and during exacerbations since they can induce muscle atrophy and even cause a true myopathy (222-224).
Antibiotics and prevention of exacerbations
These acute episodes have several negative consequences on factors involved in muscle dysfunction. On the one hand, exacerbations lead to an increase in pulmonary and systemic inflammation (225). On the other, they are associated with a more sedentary behaviour that can be extreme in bedridden patients, and a frequent need of treatment with systemic steroids (17,187,224,226,227). Moreover, exacerbations induce an increase in the work of breathing and therefore, in the overall energy consumption by respiratory muscles. Thus, an adequate prevention and treatment of these acute episodes is also essential to prevent deterioration of muscle function in COPD patients.
Mechanical ventilation
Rest is definitely the best therapeutic measure when a component of acute-subacute muscle fatigue is present. Ventilatory support reduces the workload of respiratory muscles (228,229), while having other beneficial effects on ventilation and perfusion. It also decreases the metabolic burden of these muscles, which can redirect energy resources to limb muscles and other body territories. There are two main types of non-invasive mechanical ventilation (NIMV), which is usually applied by a nasal or nasobucal mask, and invasive ventilation (IMV) that requires endotracheal intubation. The clearest utility of NIMV is in severe exacerbations, where there is an acute-subacute increase of respiratory muscle work, in a pulmonary and systemic inflammatory context. The concrete indication of NIMV in COPD patients is the hypercapnic respiratory failure with severe acidosis but consciousness preservation (230,231). In this situation, this technique prevents endotracheal intubation and reduces mortality (230-232). However, there are a number of factors that can determine the failure of NIMV in acute patients. The main ones are a poor nutritional status, a deterioration in consciousness or a poor general condition (230,233). The evidence of benefits with NIMV is much lower in stable COPD patients, although it could improve survival in those with hypercapnia (234), reducing their admissions in intensive care units (235). Nevertheless, IMV may be necessary if NIMV fails. The beneficial effects of both modalities of ventilatory support on respiratory muscles and other physiological components as well as on clinical outcomes are similar, but IMV increases the number of complications (236). Moreover, fully controlled forms of IMV should be avoided as far as possible, since they have serious negative effects on respiratory muscles, which suffer from deconditioning and oxidative stress, and become atrophic and functionally impaired (237-239).
To optimize the control of comorbidities
Several of the most frequent comorbidities associated with COPD such as chronic heart failure, diabetes or cancer can also involve muscle abnormalities themselves (5,17,240-242). Therefore, a good control of these entities should be ensured.
Measures for a healthy aging
Advanced age is associated with sarcopenia and a worsening of muscle function (243,244). Consequently, it is essential to prevent as far as possible these changes through a healthy diet, nutritional supplements if necessary, and the maintenance of an appropriate level of physical activity (245).
Other drugs
It has been recently shown that 'calcium sensitizers', as levosimendan, are able to improve the strength of the diaphragm and prevent fatigue both in healthy subjects and COPD patients (246,247). However, studies on its potential effects on the limb muscles are still lacking. Transcription factors such as NF-kappa B and MAPK in turn appear to have positive effects on muscle mass and therefore, may also be useful in muscle dysfunction (248). Again, there is a lack of studies in COPD patients. Some vasoactive drugs such as dobutamine have also been used in the past to improve the aerobic capacity of the muscle by increasing blood flow and therefore, the oxygen supply. However, the results are controversial (249,250). Something similar has happened with terbutaline (251,252). In fact, other beta-agonists such as clenbuterol or formoterol have demonstrated anabolic effects on muscle mass (253-255). It has very recently been shown that iron administration results in improvements in limb muscle function and exercise capacity, even in COPD patients without anemia (256). This would likely be the result of an improved transport and use of oxygen in the muscle by the action of proteins such as myoglobin and cytochrome C (mitochondrial respiratory chain), respectively (256,257).
Finally, in the past decades there has been some interest in the possible beneficial effects of almitrine on the function of the diaphragm and other muscles (258,259). Unfortunately, no clear effects have been demonstrated in COPD patients. The same has occurred with theophylline, which showed some promising initial results (260) that have not been widely reproduced.
Conclusions (Figure 2)
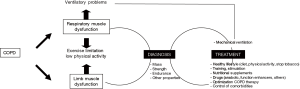
Muscle dysfunction is highly prevalent in COPD patients and affects both respiratory and peripheral muscles, conditioning their symptoms, quality of life and mortality. Whereas respiratory muscle dysfunction is found mainly in patients with severe disease, limb muscle dysfunction can be present even in patients with mild-to-moderate stages. Therefore, an early diagnosis is necessary to ensure a good therapeutic management. Respiratory muscle dysfunction can be easily detected in the clinical setting through determination of maximum respiratory pressures at the mouth, which are a good expression of their strength. The maximal voluntary isometric contraction of the quadriceps is the most used technique for limb muscles. In non-totally compliant patients electrical or magnetic stimulation can be used to induce maximal maneuvers in both muscle groups. Although the assessment of muscle endurance would probably be more useful than that of strength from a clinical point of view, its implementation is more complex and limited to specialized laboratories. The main factor involved in respiratory muscle dysfunction is pulmonary hyperinflation, while deconditioning plays a key role in limb muscle dysfunction. In addition, many systemic factors such as tobacco, nutritional abnormalities, systemic inflammation and exacerbations target both muscle groups. Accordingly, the therapy should be multidimensional, including abstinence from smoking, improvement of the nutritional status (healthy diet and eventually supplements or even anabolic drugs), good control of the respiratory disease, increased level of physical activity and training. Some drugs have recently shown promising results on improving muscle function in COPD patients, but there is still a lack of conclusive studies.
Acknowledgements
To Jonathan McFarland for his editing support.
Funding: This study was funded by SAF2011-26908 & SAF2014-54371, 2014SGR424, FUCAP 2011, SEPAR 2015 & 2016, Menarini 2015-16 and CIBERES.
Footnote
Conflicts of Interest: The authors have no conflicts of interest to declare.
References
- Global Initiative for Chronic Obstructive Lung Disease (GOLD). Available online: http://www.goldcopd.org/, accessed in August 2016.
- Miravitlles M, Soler-Cataluña JJ, Calle M, et al. Spanish guideline for COPD (GesEPOC). Update 2014. Arch Bronconeumol 2014;50 Suppl 1:1-16. [PubMed]
- Montes de Oca M, López Varela MV, Acuña A, et al. ALAT-2014 Chronic Obstructive Pulmonary Disease (COPD) Clinical Practice Guidelines: questions and answers. Arch Bronconeumol 2015;51:403-16. [PubMed]
- Barreiro E, Bustamante V, Cejudo P, et al. Guidelines for the evaluation and treatment of muscle dysfunction in patients with chronic obstructive pulmonary disease. Arch Bronconeumol 2015;51:384-95. [PubMed]
- Maltais F, Decramer M, Casaburi R, et al. An official American Thoracic Society/European Respiratory Society statement: update on limb muscle dysfunction in chronic obstructive pulmonary disease. Am J Respir Crit Care Med 2014;189:e15-62. [Crossref] [PubMed]
- Gea J, Pascual S, Casadevall C, et al. Muscle dysfunction in chronic obstructive pulmonary disease: update on causes and biological findings. J Thorac Dis 2015;7:E418-38. [PubMed]
- Edwards RH, Faulkner JA. Structure and function of the respiratory muscles. In: Roussos C. editor. The Thorax. New York: Marcel Dekker Inc., 1995:185-217.
- Bellemare F, Bono D, D’Angelo E. Electrical and mechanical output of the expiratory muscles in anesthetized dogs. Respir Physiol 1991;84:171-83. [Crossref] [PubMed]
- Chang AB. The physiology of cough. Paediatr Respir Rev 2006;7:2-8. [Crossref] [PubMed]
- Orozco-Levi M, Gea J, Monells J, et al. Activity of latissimus dorsi muscle during inspiratory thershold loads. Eur Respir J 1995;8:441-5. [Crossref] [PubMed]
- Hug F, Raux M, Prella M, et al. Optimized analysis of surface electromyograms of the scalenes during quiet breathing in humans. Respir Physiol Neurobiol 2006;150:75-81. [Crossref] [PubMed]
- Barreiro E, de la Puente B, Minguella J, et al. Oxidative stress and respiratory muscle dysfunction in severe chronic obstructive pulmonary disease. Am J Respir Crit Care Med 2005;171:1116-24. [Crossref] [PubMed]
- Levine S, Kaiser L, Leferovich J, et al. Cellular adaptations in the diaphragm in chronic obstructive pulmonary disease. N Engl J Med 1997;337:1799-806. [Crossref] [PubMed]
- Polkey MI, Kyroussis D, Hamnegard CH, et al. Diaphragm strength in chronic obstructive pulmonary disease. Am J Respir Crit Care Med 1996;154:1310-7. [Crossref] [PubMed]
- Seymour JM, Spruit MA, Hopkinson NS, et al. The prevalence of quadriceps weakness in COPD and the relationship with disease severity. Eur Respir J 2010;36:81-8. [Crossref] [PubMed]
- Goldman MD, Grassino A, Mead J, et al. Mechanics of the human diaphragm during voluntary contraction: dynamics. J Appl Physiol Respir Environ Exerc Physiol 1978;44:840-8. [PubMed]
- Gea J, Agustí A, Roca J. Pathophysiology of muscle dysfunction in COPD. J Appl Physiol (1985) 2013;114:1222-34. [PubMed]
- Ferrari K, Goti P, Misuri G, et al. Chronic exertional dyspnea and respiratory muscle function in patients with chronic obstructive pulmonary disease. Lung 1997;175:311-9. [Crossref] [PubMed]
- Similowski T, Yan S, Gauthier AP, et al. Contractile properties of the human diaphragm during chronic hyperinflation. N Engl J Med 1991;325:917-23. [Crossref] [PubMed]
- Orozco-Levi M, Gea J, Lloreta JL, et al. Subcellular adaptation of the human diaphragm in chronic obstructive pulmonary disease. Eur Respir J 1999;13:371-8. [Crossref] [PubMed]
- Levine S, Nguyen T, Friscia M, et al. Parasternal intercostal muscle remodeling in severe chronic obstructive pulmonary disease. J Appl Physiol (1985) 2006;101:1297-302. [PubMed]
- Gea J, Hamid Q, Czaika G, et al. Expression of myosin heavy-chain isoforms in the respiratory muscles following inspiratory resistive breathing. Am J Respir Crit Care Med 2000;161:1274-8. [Crossref] [PubMed]
- Gosselink R, Troosters T, Decramer M. Distribution of muscle weakness in patients with stable chronic obstructive pulmonary disease. J Cardiopulm Rehabil 2000;20:353-60. [Crossref] [PubMed]
- Caron MA, Debigaré R, Dekhuijzen PN, et al. Comparative assessment of the quadriceps and the diaphragm in patients with COPD. J Appl Physiol (1985) 2009;107:952-61. [PubMed]
- Jakobsson P, Jorfeldt L, Brundin A. Skeletal muscle metabolites and fiber types in patients with advanced chronic obstructive pulmonary disease (COPD), with and without chronic respiratory failure. Eur Respir J 1990;3:192-6. [PubMed]
- Jakobsson P, Jordfelt L. Henriksson. Metabolic enzyme activity in the quadriceps femoris muscle in patients with severe chronic obstructive pulmonary disease. Am J Respir Crit Care Med 1995;151:374-7. [Crossref] [PubMed]
- Simard C, Maltais F, Leblanc P, et al. Mitochondrial and capillarity changes in vastus lateralis muscle of COPD patients: electron microscopy study. Med Sci Sports Exerc 1996;28:S95. [Crossref]
- Natanek SA, Gosker HR, Slot IG, et al. Pathways associated with reduced quadriceps oxidative fibres and endurance in COPD. Eur Respir J 2013;41:1275-83. [Crossref] [PubMed]
- Engelen MP, Schols AM, Does JD, et al. Exercise-induced lactate increase in relation to muscle substrates in patients with Chronic Obstructive Pulmonary Disease. Am J Respir Crit Care Med 2000;162:1697-704. [Crossref] [PubMed]
- Jones SE, Maddocks M, Kon SS, et al. Sarcopenia in COPD: prevalence, clinical correlates and response to pulmonary rehabilitation. Thorax 2015;70:213-8. [Crossref] [PubMed]
- Hernández N, Orozco-Levi M, Belalcazar V, et al. Dual morphometrical changes of the deltoid muscle in patients with COPD. Respir Physiol Neurobiol 2003;134:219-29. [Crossref] [PubMed]
- Sato Y, Asoh T, Honda Y, et al. Morphological and histochemical evaluation of biceps muscle in patients with chronic pulmonary emphysema manifesting generalized emaciation. Eur Neurol 1997;37:116-21. [Crossref] [PubMed]
- Gea JG, Pasto M, Carmona M, et al. Metabolic characteristics of the deltoid muscle in patients with chronic obstructive pulmonary disease. Eur Respir J 2001;17:939-45. [Crossref] [PubMed]
- Ramírez-Sarmiento A, Orozco-Levi M, Barreiro E, et al. Expiratory muscle endurance in chronic obstructive pulmonary disease. Thorax 2002;57:132-6. [Crossref] [PubMed]
- Ramirez-Sarmiento A, Orozco-Levi M, Guell R, et al. Inspiratory muscle training in patients with chronic obstructive pulmonary disease: structural adaptation and physiologic outcomes. Am J Respir Crit Care Med 2002;166:1491-7. [Crossref] [PubMed]
- Vilaró J, Ramirez-Sarmiento A, Martínez-Llorens JM, et al. Global muscle dysfunction as a risk factor of readmission to hospital due to COPD exacerbations. Respir Med 2010;104:1896-902. [Crossref] [PubMed]
- Killian KJ, Leblanc P, Martin DH, et al. Exercise capacity and ventilatory, circulatory, and symptom limitation in patients with chronic airflow limitation. Am Rev Respir Dis 1992;146:935-40. [Crossref] [PubMed]
- Roche N, Zureik M, Soussan D, et al. Predictors of outcomes in COPD exacerbation cases presenting to the emergency department. Eur Respir J 2008;32:953-61. [Crossref] [PubMed]
- Liu D, Peng SH, Zhang J, et al. Prediction of short term re-exacerbation in patients with acute exacerbation of chronic obstructive pulmonary disease. Int J Chron Obstruct Pulmon Dis 2015;10:1265-73. [PubMed]
- Barreiro E, Gea J. Respiratory and Limb Muscle Dysfunction in COPD. COPD 2015;12:413-26. [Crossref] [PubMed]
- Piquet J, Chavaillon JM, David P, et al. High-risk patients following hospitalisation for an acute exacerbation of COPD. Eur Respir J 2013;42:946-55. [Crossref] [PubMed]
- Díaz MC, Ospina-Tascón GA, Salazar C BC. Respiratory muscle dysfunction: a multicausal entity in the critically ill patient undergoing mechanical ventilation. Arch Bronconeumol 2014;50:73-7. [Crossref] [PubMed]
- Ambrosino N. Weaning and respiratory muscle dysfunction: the egg-chicken dilemma. Chest 2005;128:481-3. [Crossref] [PubMed]
- Gayan-Ramirez G, Decramer M. Mechanisms of striated muscle dysfunction during acute exacerbations of COPD. J Appl Physiol (1985) 2013;114:1291-9. [PubMed]
- O'Donnell DE, Laveneziana P. The clinical importance of dynamic lung hyperinflation in COPD. COPD 2006;3:219-32. [Crossref] [PubMed]
- Bernard S, LeBlanc P, Whittom F, et al. Peripheral muscle weakness in patients with chronic obstructive pulmonary disease. Am J Respir Crit Care Med 1998;158:629-34. [Crossref] [PubMed]
- Clark CJ, Cochrane LM, Mackay E, et al. Skeletal muscle strength and endurance in patients with mild COPD and the effects of weight training. Eur Respir J 2000;15:92-7. [Crossref] [PubMed]
- Coronell C, Orozco-Levi M, Méndez R, et al. Relevance of assessing quadriceps endurance in patients with COPD. Eur Respir J 2004;24:129-36. [Crossref] [PubMed]
- Gagnon P, Maltais F, Bouyer L, et al. Distal leg muscle function in patients with COPD. COPD 2013;10:235-42. [Crossref] [PubMed]
- Marquis N, Debigaré R, Bouyer L, et al. Physiology of walking in patients with moderate to severe chronic obstructive pulmonary disease. Med Sci Sports Exerc 2009;41:1540-8. [Crossref] [PubMed]
- Gosselink R, Troosters T, Decramer M. Peripheral muscle weakness contributes to exercise limitation in COPD. Am J Respir Crit Care Med 1996;153:976-80. [Crossref] [PubMed]
- Swallow EB, Reyes D, Hopkinson NS, et al. Quadriceps strength predicts mortality in patients with moderate to severe chronic obstructive pulmonary disease. Thorax 2007;62:115-20. [Crossref] [PubMed]
- Marquis K, Debigaré R, Lacasse Y, et al. Midthigh muscle cross-sectional area is a better predictor of mortality than body mass index in patients with chronic obstructive pulmonary disease. Am J Respir Crit Care Med 2002;166:809-13. [Crossref] [PubMed]
- Decramer M, Gosselink R, Troosters T, et al. Muscle weakness is related to utilization of health care resources in COPD patients. Eur Respir J 1997;10:417-23. [Crossref] [PubMed]
- Mostert R, Goris A, Weling-Scheepers C, et al. Tissue depletion and health related quality of life in patients with chronic obstructive pulmonary disease. Respir Med 2000;94:859-67. [Crossref] [PubMed]
- Pumar MI, Gray CR, Walsh JR, et al. Anxiety and depression-Important psychological comorbidities of COPD. J Thorac Dis 2014;6:1615-31. [PubMed]
- Garcia-Aymerich J, Farrero E, Félez MA, et al. Risk factors of readmission to hospital for a COPD exacerbation: a prospective study. Thorax 2003;58:100-5. [Crossref] [PubMed]
- Garcia-Aymerich J, Lange P, Benet M, et al. Regular physical activity reduces hospital admission and mortality in chronic obstructive pulmonary disease: a population based cohort study. Thorax 2006;61:772-8. [Crossref] [PubMed]
- Gimeno-Santos E, Frei A, Steurer-Stey C, et al. Determinants and outcomes of physical activity in patients with COPD: a systematic review. Thorax 2014;69:731-9. [Crossref] [PubMed]
- Shrikrishna D, Patel M, Tanner RJ, et al. Quadriceps wasting and physical inactivity in patients with COPD. Eur Respir J 2012;40:1115-22. [Crossref] [PubMed]
- Mier-Jedrzejowicz A, Brophy C, Moxham J, et al. Assessment of diaphragm weakness. Am Rev Respir Dis 1988;137:877-83. [Crossref] [PubMed]
- Song Y, Chen R, Zhan Q, et al. The optimum timing to wean invasive ventilation for patients with AECOPD or COPD with pulmonary infection. Int J Chron Obstruct Pulmon Dis 2016;11:535-42. [Crossref] [PubMed]
- Hughes PD, Polkey MI, Moxham J, et al. Long-term recovery of diaphragm strength in neuralgic amyotrophy. Eur Respir J 1999;13:379-84. [Crossref] [PubMed]
- Orozco-Levi M, Gayete A, Rodríguez C, et al. Non-invasive functional evaluation of the reserve in fatigue and the diaphragm structure using transthoracic echography in B and M modes. Arch Bronconeumol 2010;46:571-9. [PubMed]
- Scheibe N, Sosnowski N, Pinkhasik A, et al. Sonographic evaluation of diaphragmatic dysfunction in COPD patients. Int J Chron Obstruct Pulmon Dis 2015;10:1925-30. [PubMed]
- Sancho-Muñoz A, Trampal C, Pascual S, et al. Muscle glucose metabolism in chronic obstructive pulmonary disease patients. Arch Bronconeumol 2014;50:221-7. [Crossref] [PubMed]
- Osman MM, Tran IT, Muzaffar R, et al. Does 18F-FDG uptake by respiratory muscles on PET/CT correlate with chronic obstructive pulmonary disease? J Nucl Med Technol 2011;39:252-7. [Crossref] [PubMed]
- Bellemare F, Grassino A. Effect of pressure and timing of contraction on human diaphragm fatigue. J Appl Physiol Respir Environ Exerc Physiol 1982;53:1190-5. [PubMed]
- Lechtzin N, Wiener CM, Shade DM, et al. Spirometry in the supine position improves the detection of diaphragmatic weakness in patients with amyotrophic lateral sclerosis. Chest 2002;121:436-42. [Crossref] [PubMed]
- White JE, Drinnan MJ, Smithson AJ, et al. Respiratory muscle activity and oxygenation during sleep in patients with muscle weakness. Eur Respir J 1995;8:807-14. [PubMed]
- Mustfa N, Moxham J. Respiratory muscle assessment in motor neurone disease. QJM 2001;94:497-502. [Crossref] [PubMed]
- Black LF, Hyatt RE. Maximal respiratory pressures: normal values and relationship to age and sex. Am Rev Respir Dis 1969;99:696-702. [PubMed]
- Morales P, Sanchis J, Cordero PJ, et al. Maximum static respiratory pressures in adults. The reference values for a Mediterranean Caucasian population. Arch Bronconeumol 1997;33:213-9. [Crossref] [PubMed]
- Polkey MI, Green M, Moxham J. Measurement of respiratory muscle strength. Thorax 1995;50:1131-5. [Crossref] [PubMed]
- Martínez-Llorens J, Ausín P, Roig A, et al. Nasal inspiratory pressure: an alternative for the assessment of inspiratory muscle strength? Arch Bronconeumol 2011;47:169-75. [Crossref] [PubMed]
- Héritier F, Rahm F, Pasche P, et al. Sniff nasal inspiratory pressure. A noninvasive assessment of inspiratory muscle strength. Am J Respir Crit Care Med 1994;150:1678-83. [Crossref] [PubMed]
- Kamide N, Ogino M, Yamashina N, et al. Sniff nasal inspiratory pressure in healthy Japanese subjects: mean values and lower limits of normal. Respiration 2009;77:58-62. [Crossref] [PubMed]
- Araújo PR, Resqueti VR, Nascimento J Junior, et al. Reference values for sniff nasal inspiratory pressure in healthy subjects in Brazil: a multicenter study. J Bras Pneumol 2012;38:700-7. [PubMed]
- Chetta A, Harris ML, Lyall RA, et al. Whistle mouth pressure as test of expiratory muscle strength. Eur Respir J 2001;17:688-95. [Crossref] [PubMed]
- Uldry C, Janssens JP, de Muralt B, et al. Sniff nasal inspiratory pressure in patients with chronic obstructive pulmonary disease. Eur Respir J 1997;10:1292-6. [Crossref] [PubMed]
- Cherniack RM, Farhi LE, Armstrong BW, et al. A comparison of esophageal and intrapleural pressure in man. J Appl Physiol 1955;8:203-11. [PubMed]
- Laroche CM, Mier AK, Moxham J, et al. The value of sniff esophageal pressures in the assessment of global inspiratory muscle strength. Am Rev Respir Dis 1988;138:598-603. [Crossref] [PubMed]
- Steier J, Kaul S, Seymour J, et al. The value of multiple tests of respiratory muscle strength. Thorax 2007;62:975-80. [Crossref] [PubMed]
- Laporta D, Grassino A. Assessment of transdiaphragmatic pressure in humans. J Appl Physiol (1985) 1985;58:1469-76. [PubMed]
- Tzelepis GE, Nasiff L, McCool FD, et al. Transmission of pressure within the abdomen. J Appl Physiol (1985) 1996;81:1111-4. [PubMed]
- Gallart L, Gea J, Aguar MC, et al. Effects of interpleural bupivacaine on respiratory muscle strength and pulmonary function. Anesthesiology 1995;83:48-55. [Crossref] [PubMed]
- Man WD, Kyroussis D, Fleming TA, et al. Cough gastric pressure and maximum expiratory mouth pressure in humans. Am J Respir Crit Care Med 2003;168:714-7. [Crossref] [PubMed]
- Mier A, Brophy C, Moxham J, et al. Twitch pressures in the assessment of diaphragm weakness. Thorax 1989;44:990-6. [Crossref] [PubMed]
- Mills GH, Kyroussis D, Hamnegard CH, et al. Unilateral magnetic stimulation of the phrenic nerve. Thorax 1995;50:1162-72. [Crossref] [PubMed]
- Watson AC, Hughes PD, Louise Harris M, et al. Measurement of twitch transdiaphragmatic, esophageal, and endotracheal tube pressure with bilateral anterolateral magnetic phrenic nerve stimulation in patients in the intensive care unit. Crit Care Med 2001;29:1325-31. [Crossref] [PubMed]
- Gea J, Espadaler JM, Guiu R, et al. Diaphragmatic activity induced by cortical stimulation: surface versus esophageal electrodes. J Appl Physiol (1985) 1993;74:655-8. [PubMed]
- Man WD, Moxham J, Polkey MI. Magnetic stimulation for the measurement of respiratory and skeletal muscle function. Eur Respir J 2004;24:846-60. [Crossref] [PubMed]
- Polkey MI, Harris ML, Hughes PD, et al. The contractile properties of the elderly human diaphragm. Am J Respir Crit Care Med 1997;155:1560-4. [Crossref] [PubMed]
- Wragg S, Aquilina R, Moran J, et al. Comparison of cervical magnetic stimulation and bilateral percutaneous electrical stimulation of the phrenic nerves in normal subjects. Eur Respir J 1994;7:1788-92. [Crossref] [PubMed]
- Bai TR, Rabinovitch BJ, Pardy RL. Near-maximal voluntary hyperpnea and ventilatory muscle function. J Appl Physiol Respir Environ Exerc Physiol 1984;57:1742-8. [PubMed]
- Larson JL, Covey MK, Berry J, et al. Discontinuous incremental threshold loading test: measure of respiratory muscle endurance in patients with COPD. Chest 1999;115:60-7. [Crossref] [PubMed]
- Martyn JB, Moreno RH, Paré PD, et al. Measurement of inspiratory muscle performance with incremental threshold loading. Am Rev Respir Dis 1987;135:919-23. [Crossref] [PubMed]
- Orozco-Levi M, Gea J, Ferrer A, et al. Expiratory muscle endurance in middle-aged healthy subjects. Lung 2001;179:93-103. [Crossref] [PubMed]
- Fiz JA, Romero P, Gomez R, et al. Indices of respiratory muscle endurance in healthy subjects. Respiration 1998;65:21-7. [Crossref] [PubMed]
- Johnson PH, Cowley AJ, Kinnear WJ. Incremental threshold loading: a standard protocol and establishment of a reference range in naive normal subjects. Eur Respir J 1997;10:2868-71. [Crossref] [PubMed]
- Ferrer A, Orozco-Levi M, Gea J, et al. Mechanical and metabolic reproducibility of resistance test of expiratory muscles with incremental threshold loading. Arch Bronconeumol 2000;36:303-12. [Crossref] [PubMed]
- Bellemare F, Grassino A. Evaluation of human diaphragm fatigue. J Appl Physiol Respir Environ Exerc Physiol 1982;53:1196-206. [PubMed]
- González C, Servera E, Celli B, et al. A simple noninvasive pressure-time index at the mouth to measure respiratory load during acute exacerbation of COPD A comparison with normal volunteers. Respir Med 2003;97:415-20. [Crossref] [PubMed]
- Esau SA, Bellemare F, Grassino A, et al. Changes in relaxation rate with diaphragmatic fatigue in humans. J Appl Physiol Respir Environ Exerc Physiol 1983;54:1353-60. [PubMed]
- Walker DJ, Farquharson F, Klenze H, et al. Diaphragmatic fatigue during inspiratory muscle loading in normoxia and hypoxia. Respir Physiol Neurobiol 2016;227:1-8. [Crossref] [PubMed]
- Mador MJ, Kufel TJ, Pineda LA, et al. Diaphragmatic fatigue and high-intensity exercise in patients with chronic obstructive pulmonary disease. Am J Respir Crit Care Med 2000;161:118-23. [Crossref] [PubMed]
- Sinderby C, Weinberg J, Sullivan L, et al. Electromyographical evidence for exercise-induced diaphragm fatigue in patients with chronic cervical cord injury or prior poliomyelitis infection. Spinal Cord 1996;34:594-601. [Crossref] [PubMed]
- Aldrich TK, Adams JM, Arora NS, et al. Power spectral analysis of the diaphragm electromyogram. J Appl Physiol Respir Environ Exerc Physiol 1983;54:1579-84. [PubMed]
- Hamilton AL, Killian KJ, Summers E, et al. Muscle strength, symptom intensity, and exercise capacity in patients with cardiorespiratory disorders. Am J Respir Crit Care Med 1995;152:2021-31. [Crossref] [PubMed]
- Killian KJ, Summers E, Jones NL, et al. Dyspnea and leg effort during incremental cycle ergometry. Am Rev Respir Dis 1992;145:1339-45. [Crossref] [PubMed]
- Mahan LK, Escott-Stump S. editors. Krause’s food, nutrition & diet therapy. 10th ed. Philadelphia (Pa): W.B. Saunders Co; 2000;970-86.
- World Health Organization (WHO). BMI classification. Available online: apps.who.int/bmi/index.jsp?introPage=intro_3.html, accessed in August 2016.
- Steiner MC, Barton RL, Singh SJ, et al. Bedside methods versus dual energy X-ray absorptiometry for body composition measurement in COPD. Eur Respir J 2002;19:626-31. [Crossref] [PubMed]
- Lerario MC, Sachs A, Lazaretti-Castro M, et al. Body composition in patients with chronic obstructive pulmonary disease: which method to use in clinical practice? Br J Nutr 2006;96:86-92. [Crossref] [PubMed]
- Gea J, Martínez-Llorens J, Barreiro E, et al. Alteraciones nutricionales en la enfermedad pulmonar obstructiva crónica. Med Clin (Barc) 2014;143:78-84. [Crossref] [PubMed]
- Schols AM, Wouters EF, Soeters PB, et al. Body composition by bioelectrical-impedance analysis compared with deuterium dilution and skin-fold anthropometry in patients with chronic obstructive pulmonary disease. Am J Clin Nutr 1991;53:421-4. [PubMed]
- Vermeeren MA, Creutzberg EC, Schols AM, et al. Prevalence of nutritional depletion in a large out-patient population of patients with COPD. Respir Med 2006;100:1349-55. [Crossref] [PubMed]
- Rutten EP, Spruit MA, Wouters EF. Critical view on diagnosing muscle wasting by single-frequency bio-electrical impedance in COPD. Respir Med 2010;104:91-8. [Crossref] [PubMed]
- Greening NJ, Harvey-Dunstan TC, Chaplin EJ, et al. Bedside assessment of quadriceps muscle by ultrasound after admission for acute exacerbations of chronic respiratory disease. Am J Respir Crit Care Med 2015;192:810-6. [Crossref] [PubMed]
- Hamaoka T, Tatsumi K, Saito Y, et al. Metabolic activity in skeletal muscles of patients with non-hypoxaemic chronic obstructive pulmonary disease studied by 31P-magnetic resonance spectroscopy. Respirology 2005;10:164-70. [Crossref] [PubMed]
- Debigaré R, Marquis K, Côté CH, et al. Catabolic/anabolic balance and muscle wasting in patients with COPD. Chest 2003;124:83-9. [Crossref] [PubMed]
- Mathur S, Takai KP, Macintyre DL, et al. Estimation of thigh muscle mass with magnetic resonance imaging in older adults and people with chronic obstructive pulmonary disease. Phys Ther 2008;88:219-30. [Crossref] [PubMed]
- Seymour JM, Ward K, Sidhu PS, et al. Ultrasound measurement of rectus femoris cross-sectional area and the relationship with quadriceps strength in COPD. Thorax 2009;64:418-23. [Crossref] [PubMed]
- Spruit MA, Sillen MJ, Groenen MT, et al. New normative values for handgrip strength: results from the UK Biobank. J Am Med Dir Assoc 2013;14:775.e5-11. [Crossref] [PubMed]
- Luna-Heredia E, Martín-Peña G, Ruiz-Galiana J. Handgrip dynamometry in healthy adults. Clin Nutr 2005;24:250-8. [Crossref] [PubMed]
- Bohannon RW. Reference values for extremity muscle strength obtained by hand-held dynamometry from adults aged 20 to 79 years. Arch Phys Med Rehabil 1997;78:26-32. [Crossref] [PubMed]
- Stone CA, Nolan B, Lawlor PG, et al. Hand-held dynamometry: tester strength is paramount, even in frail populations. J Rehabil Med 2011;43:808-11. [Crossref] [PubMed]
- Klidjian AM, Foster KJ, Kammerling RM, et al. Relation of anthropometric and dynamometric variables to serious postoperative complications. Br Med J 1980;281:899-901. [Crossref] [PubMed]
- Webb AR, Newman LA, Taylor M, et al. Hand grip dynamometry as a predictor of postoperative complications reappraisal using age standardized grip strengths. JPEN J Parenter Enteral Nutr 1989;13:30-3. [Crossref] [PubMed]
- Russell DM, Leiter LA, Whitwell J, et al. Skeletal muscle function during hypocaloric diets and fasting: a comparison with standard nutritional assessment parameters. Am J Clin Nutr 1983;37:133-8. [PubMed]
- Hurley MV, Rees J, Newham DJ. Quadriceps function, proprioceptive acuity and functional performance in healthy young, middle-aged and elderly subjects. Age Ageing 1998;27:55-62. [Crossref] [PubMed]
- Nellessen AG, Donária L, Hernandes NA, et al. Analysis of three different equations for predicting quadriceps femoris muscle strength in patients with COPD. J Bras Pneumol 2015;41:305-12. [Crossref] [PubMed]
- Neder JA, Nery LE, Shinzato GT, et al. Reference values for concentric knee isokinetic strength and power in nonathletic men and women from 20 to 80 years old. J Orthop Sports Phys Ther 1999;29:116-26. [Crossref] [PubMed]
- Beauchamp MK, Sibley KM, Lakhani B, et al. Impairments in systems underlying control of balance in COPD. Chest 2012;141:1496-503. [Crossref] [PubMed]
- Ausin P, Martinez-Llorens J, Sabate-Bresco M, et al. Sex differences in function and structure of the quadriceps muscle in COPD patients. Chron Resp Dis 2016. In press.
- Malaguti C, Napolis LM, Villaça D, et al. Relationship between peripheral muscle structure and function in patients with chronic obstructive pulmonary disease with different nutritional status. J Strength Cond Res 2011;25:1795-803. [Crossref] [PubMed]
- Beretta-Piccoli M, D'Antona G, Barbero M, et al. Evaluation of central and peripheral fatigue in the quadriceps using fractal dimension and conduction velocity in young females. PLoS One 2015;10:e0123921. [Crossref] [PubMed]
- Polkey MI, Kyroussis D, Hamnegard CH, et al. Quadriceps strength and fatigue assessed by magnetic stimulation of the femoral nerve in man. Muscle Nerve 1996;19:549-55. [Crossref] [PubMed]
- Saey D, Debigare R, LeBlanc P, et al. Contractile leg fatigue after cycle exercise: a factor limiting exercise in patients with chronic obstructive pulmonary disease. Am J Respir Crit Care Med 2003;168:425-30. [Crossref] [PubMed]
- Hamnegård CH, Sedler M, Polkey MI, et al. Quadriceps strength assessed by magnetic stimulation of the femoral nerve in normal subjects. Clin Physiol Funct Imaging 2004;24:276-80. [Crossref] [PubMed]
- Serres I, Gautier V, Varray A, et al. Impaired skeletal muscle endurance related to physical inactivity and altered lung function in COPD patients. Chest 1998;113:900-5. [Crossref] [PubMed]
- Van't Hul A, Harlaar J, Gosselink R, et al. Quadriceps muscle endurance in patients with chronic obstructive pulmonary disease. Muscle Nerve 2004;29:267-74. [Crossref] [PubMed]
- Bachasson D, Decorte N, Wuyam B, et al. Original Research: Central and peripheral quadriceps fatigue in young and middle-aged untrained and endurance-trained men: A comparative study. Exp Biol Med (Maywood) 2016;241:1844-52. [Crossref] [PubMed]
- Swallow EB, Gosker HR, Ward KA, et al. A novel technique for nonvolitional assessment of quadriceps muscle endurance in humans. J Appl Physiol (1985) 2007;103:739-46. [PubMed]
- Gagnon P, Saey D, Vivodtzev I, et al. Impact of preinduced quadriceps fatigue on exercise response in chronic obstructive pulmonary disease and healthy subjects. J Appl Physiol (1985) 2009;107:832-40. [PubMed]
- Mador MJ, Deniz O, Aggarwal A, et al. Quadriceps fatigability after single muscle exercise in patients with chronic obstructive pulmonary disease. Am J Respir Crit Care Med 2003;168:102-8. [Crossref] [PubMed]
- Saey D, Côté CH, Mador MJ, et al. Assessment of muscle fatigue during exercise in chronic obstructive pulmonary disease. Muscle Nerve 2006;34:62-71. [Crossref] [PubMed]
- Spruit MA, Singh SJ, Garvey C, et al. An official American Thoracic Society/European Respiratory Society statement: key concepts and advances in pulmonary rehabilitation. Am J Respir Crit Care Med 2013;188:e13-64. [Crossref] [PubMed]
- Harris KK, Zopey M, Friedman TC. Metabolic effects of smoking cessation. Nat Rev Endocrinol 2016;12:299-308. [Crossref] [PubMed]
- Rom O, Reznick AZ, Keidar Z, et al. Smoking cessation-related weight gain--beneficial effects on muscle mass, strength and bone health. Addiction 2015;110:326-35. [Crossref] [PubMed]
- Watz H, Pitta F, Rochester CL, et al. An official European Respiratory Society statement on physical activity in COPD. Eur Respir J 2014;44:1521-37. [Crossref] [PubMed]
- González-Gutiérrez MV, Guerrero Velázquez J, Morales García C, et al. Predictive model for anxiety and depression in Spanish patients with stable Chronic Obstructive Pulmonary Disease. Arch Bronconeumol 2016;52:151-7. [Crossref] [PubMed]
- Loprinzi PD, Walker JF. Increased daily movement associates with reduced mortality among COPD patients having systemic inflammation. Int J Clin Pract 2016;70:286-91. [Crossref] [PubMed]
- Maltais F, LeBlanc P, Simard C, et al. Skeletal muscle adaptation to endurance training in patients with chronic obstructive pulmonary disease. Am J Respir Crit Care Med 1996;154:442-7. [Crossref] [PubMed]
- Le Bourdelles G, Viires N, Boczkowski J, et al. Effects of mechanical ventilation on diaphragmatic contractile properties in rats. Am J Respir Crit Care Med 1994;149:1539-44. [Crossref] [PubMed]
- Whittom F, Jobin J, Simard PM, et al. Histochemical and morphological characteristics of the vastus lateralis muscle in patients with chronic obstructive pulmonary disease. Med Sci Sports Exerc 1998;30:1467-74. [Crossref] [PubMed]
- Franssen FM, Broekhuizen R, Janssen PP, et al. Effects of whole-body exercise training on body composition and functional capacity in normal-weight patients with COPD. Chest 2004;125:2021-8. [Crossref] [PubMed]
- Spruit MA, Gosselink R, Troosters T, et al. Resistance versus endurance training in patients with COPD and peripheral muscle weakness. Eur Respir J 2002;19:1072-8. [Crossref] [PubMed]
- Güell Rous MR, Díaz Lobato S, Rodríguez Trigo G, et al. Pulmonary rehabilitation. Sociedad Española de Neumología y Cirugía Torácica (SEPAR). Arch Bronconeumol 2014;50:332-44. [PubMed]
- Porszasz J, Emtner M, Goto S, et al. Exercise training decreases ventilatory requirements and exercise-induced hyperinflation at submaximal intensities in patients with COPD. Chest 2005;128:2025-34. [Crossref] [PubMed]
- Ries AL, Bauldoff GS, Carlin BW, et al. Pulmonary Rehabilitation: Joint ACCP/AACVPR Evidence-Based Clinical Practice Guidelines. Chest. 2007;131:4S-42S. [Crossref] [PubMed]
- Güell R, Resqueti V, Sangenis M, et al. Impact of pulmonary rehabilitation on psychosocial morbidity in patients with severe COPD. Chest 2006;129:899-904. [Crossref] [PubMed]
- Franssen FM, Broekhuizen R, Janssen PP, et al. Limb muscle dysfunction in COPD: effects of muscle wasting and exercise training. Med Sci Sports Exerc 2005;37:2-9. [Crossref] [PubMed]
- Vogiatzis I, Terzis G, Nanas S, et al. Skeletal muscle adaptations to interval training in patients with advanced COPD. Chest 2005;128:3838-45. [Crossref] [PubMed]
- Vogiatzis I, Stratakos G, Simoes DC, et al. Effects of rehabilitative exercise on peripheral muscle TNFalpha, IL-6, IGF-I and MyoD expression in patients with COPD. Thorax 2007;62:950-6. [Crossref] [PubMed]
- Vogiatzis I, Simoes DC, Stratakos G, et al. Effect of pulmonary rehabilitation on muscle remodelling in cachectic patients with COPD. Eur Respir J 2010;36:301-10. [Crossref] [PubMed]
- Vogiatzis I, Terzis G, Stratakos G, et al. Effect of pulmonary rehabilitation on peripheral muscle fiber remodeling in patients with COPD in GOLD stages II to IV. Chest 2011;140:744-52. [Crossref] [PubMed]
- Ortega F, Toral J, Cejudo P, et al. Comparison of effects of strength and endurance training in patients with chronic obstructive pulmonary disease. Am J Respir Crit Care Med 2002;166:669-74. [Crossref] [PubMed]
- Maltais F, LeBlanc P, Jobin J, et al. Intensity of training and physiologic adaptation in patients with chronic obstructive pulmonary disease. Am J Respir Crit Care Med 1997;155:555-61. [Crossref] [PubMed]
- Maltais F, Simard AA, Simard C, et al. Oxidative capacity of the skeletal muscle and lactic acid kinetics during exercise in normal subjects and in patients with COPD. Am J Respir Crit Care Med 1996;153:288-93. [Crossref] [PubMed]
- Maltais F, LeBlanc P, Whittom F, et al. Oxidative enzyme activities of the vastus lateralis muscle and the functional status in patients with COPD. Thorax 2000;55:848-53. [Crossref] [PubMed]
- Horowitz MB, Littenberg B, Mahler DA. Dyspnea ratings for prescribing exercise intensity in patients with COPD. Chest 1996;109:1169-75. [Crossref] [PubMed]
- Kawamori N, Haff GG. The optimal training load for the development of muscular power. J Strength Cond Res 2004;18:675-84. [PubMed]
- Barreiro E, Rabinovich R, Marin-Corral J, et al. Chronic endurance exercise induces quadriceps nitrosative stress in patients with severe COPD. Thorax 2009;64:13-9. [Crossref] [PubMed]
- Baker D, Nance S, Moore M. The load that maximizes the average mechanical power output during explosive bench press throws in highly trained athletes. J Strength Cond Res 2001;15:20-4. [PubMed]
- Rodriguez DA, Kalko S, Puig-Vilanova E, et al. Muscle and blood redox status after exercise training in severe COPD patients. Free Radic Biol Med 2012;52:88-94. [Crossref] [PubMed]
- Janaudis-Ferreira T, Hill K, Goldstein R, et al. Arm exercise training in patients with chronic obstructive pulmonary disease: a systematic review. J Cardiopulm Rehabil Prev 2009;29:277-83. [Crossref] [PubMed]
- McKeough ZJ, Bye PT, Alison JA. Arm exercise training in chronic obstructive pulmonary disease: a randomised controlled trial. Chron Respir Dis 2012;9:153-62. [Crossref] [PubMed]
- Criner GJ, Celli BR. Effect of unsupported arm exercise on ventilatory muscle recruitment in patients with severe chronic airflow obstruction. Am Rev Respir Dis 1988;138:856-61. [Crossref] [PubMed]
- Neder JA, Sword D, Ward SA, et al. Home based neuromuscular electrical stimulation as a new rehabilitative strategy for severely disabled patients with chronic obstructive pulmonary disease (COPD). Thorax 2002;57:333-7. [Crossref] [PubMed]
- Bourjeily-Habr G, Rochester CL, Palermo F, et al. Randomised controlled trial of transcutaneous electrical muscle stimulation of the lower extremities in patients with chronic obstructive pulmonary disease. Thorax 2002;57:1045-9. [Crossref] [PubMed]
- Sillen MJ, Speksnijder CM, Eterman RM, et al. Effects of neuromuscular electrical stimulation of muscles of ambulation in patients with chronic heart failure or COPD: a systematic review of the English-language literature. Chest 2009;136:44-61. [Crossref] [PubMed]
- Sillen MJ, Franssen FM, Delbressine JM, et al. Efficacy of lower-limb muscle training modalities in severely dyspnoeic individuals with COPD and quadriceps muscle weakness: results from the DICES trial. Thorax 2014;69:525-31. [Crossref] [PubMed]
- Bustamante V, Casanova J, López de Santamaría E, et al. Redox balance following magnetic stimulation training in the quadriceps of patients with severe COPD. Free Radic Res 2008;42:939-48. [Crossref] [PubMed]
- Bustamante V, López de Santa María E, Gorostiza A, et al. Muscle training with repetitive magnetic stimulation of the quadriceps in severe COPD patients. Respir Med 2010;104:237-45. [Crossref] [PubMed]
- Troosters T, Probst VS, Crul T, et al. Resistance training prevents deterioration in quadriceps muscle function during acute exacerbations of chronic obstructive pulmonary disease. Am J Respir Crit Care Med 2010;181:1072-7. [Crossref] [PubMed]
- Abdellaoui A, Préfaut C, Gouzi F, et al. Skeletal muscle effects of electrostimulation after COPD exacerbation: a pilot study. Eur Respir J 2011;38:781-8. [Crossref] [PubMed]
- Weekes CE, Emery PW, Elia M. Dietary counselling and food fortification in stable COPD: a randomised trial. Thorax 2009;64:326-31. [Crossref] [PubMed]
- Berthon BS, Wood LG. Nutrition and respiratory health--feature review. Nutrients 2015;7:1618-43. [Crossref] [PubMed]
- Schols AM, Ferreira IM, Franssen FM, et al. Nutritional assessment and therapy in COPD: a European Respiratory Society statement. Eur Respir J 2014;44:1504-20. [Crossref] [PubMed]
- García de Tena J, El Hachem Debek A, Hernández Gutiérrez C, et al. The role of vitamin D in chronic obstructive pulmonary disease, asthma and other respiratory diseases. Arch Bronconeumol 2014;50:179-84. [Crossref] [PubMed]
- Creutzberg EC, Wouters EF, Mostert R, et al. Efficacy of nutritional supplementation therapy in depleted patients with chronic obstructive pulmonary disease. Nutrition 2003;19:120-7. [Crossref] [PubMed]
- Nissen SL, Sharp RL. Effect of dietary supplements on lean mass and strength gains with resistance exercise: a meta-analysis. J Appl Physiol (1985) 2003;94:651-9. [PubMed]
- Barreiro E, Gea J. Amino acid and protein metabolism in pulmonary diseases and nutritional abnormalities: A special focus on Chronic Obstructive Pulmonary Disease. Chapter 12. In: Dardevet D. editor. The molecular nutrition of amino acids and proteins. Amsterdam: Elsevier, 2016:145-60.
- Schols AM, Slangen J, Volovics L, et al. Weight loss is a reversible factor in the prognosis of chronic obstructive pulmonary disease. Am J Respir Crit Care Med 1998;157:1791-7. [Crossref] [PubMed]
- Schols AM, Soeters PB, Mostert R, et al. Energy balance in chronic obstructive pulmonary disease. Am Rev Respir Dis 1991;143:1248-52. [Crossref] [PubMed]
- Creutzberg EC, Casaburi R. Endocrinological disturbances in chronic obstructive pulmonary disease. Eur Respir J Suppl 2003;46:76s-80s. [Crossref] [PubMed]
- Ebner N, Springer J, Kalantar-Zadeh K, et al. Mechanism and novel therapeutic approaches to wasting in chronic disease. Maturitas 2013;75:199-206. [Crossref] [PubMed]
- Burdet L, de Muralt B, Schutz Y, et al. Administration of growth hormone to underweight patients with chronic obstructive pulmonary disease. A prospective, randomized, controlled study. Am J Respir Crit Care Med 1997;156:1800-6. [Crossref] [PubMed]
- Nagaya N, Itoh T, Murakami S, et al. Treatment of cachexia with ghrelin in patients with COPD. Chest 2005;128:1187-93. [Crossref] [PubMed]
- Goldspink G. Cloning of local growth factors involved in the determination of muscle mass. Br J Sports Med 2000;34:159-60. [Crossref] [PubMed]
- Bachl N, Derman W, Engebretsen L, et al. Therapeutic use of growth factors in the musculoskeletal system in sports-related injuries. J Sports Med Phys Fitness 2009;49:346-57. [PubMed]
- Creaney L, Hamilton B. Growth factor delivery methods in the management of sports injuries: the state of play. Br J Sports Med 2008;42:314-20. [Crossref] [PubMed]
- Barreiro E, Gáldiz JB, Mariñán M, et al. Respiratory loading intensity and diaphragm oxidative stress: N-acetyl-cysteine effects. J Appl Physiol (1985) 2006;100:555-63. [PubMed]
- Rennard SI, Fogarty C, Kelsen S, et al. The safety and efficacy of infliximab in moderate to severe chronic obstructive pulmonary disease. Am J Respir Crit Care Med 2007;175:926-34. [Crossref] [PubMed]
- Rogliani P, Calzetta L, Ora J, et al. Canakinumab for the treatment of chronic obstructive pulmonary disease. Pulm Pharmacol Ther 2015;31:15-27. [Crossref] [PubMed]
- Rossman MJ, Garten RS, Groot HJ, et al. Ascorbate infusion increases skeletal muscle fatigue resistance in patients with chronic obstructive pulmonary disease. Am J Physiol Regul Integr Comp Physiol 2013;305:R1163-70. [Crossref] [PubMed]
- Rahman I, MacNee W. Antioxidant pharmacological therapies for COPD. Curr Opin Pharmacol 2012;12:256-65. [Crossref] [PubMed]
- Troosters T, Bourbeau J, Maltais F, et al. Enhancing exercise tolerance and physical activity in COPD with combined pharmacological and non-pharmacological interventions: PHYSACTO randomised, placebo-controlled study design. BMJ Open 2016;6:e010106. [Crossref] [PubMed]
- Cohen JS, Miles MC, Donohue JF, et al. Dual therapy strategies for COPD: the scientific rationale for LAMA + LABA. Int J Chron Obstruct Pulmon Dis 2016;11:785-97. [PubMed]
- Rossi A, Aisanov Z, Avdeev S, et al. Mechanisms, assessment and therapeutic implications of lung hyperinflation in COPD. Respir Med 2015;109:785-802. [Crossref] [PubMed]
- Berton DC, Barbosa PB, Takara LS, et al. Bronchodilators accelerate the dynamics of muscle O2 delivery and utilisation during exercise in COPD. Thorax 2010;65:588-93. [Crossref] [PubMed]
- Nardini S, Camiciottoli G, Locicero S, et al. COPD: maximization of bronchodilation. Multidiscip Respir Med 2014;9:50. [Crossref] [PubMed]
- Watz H, Mailänder C, Baier M, et al. Effects of indacaterol/glycopyrronium (QVA149) on lung hyperinflation and physical activity in patients with moderate to severe COPD: a randomised, placebo-controlled, crossover study (The MOVE Study). BMC Pulm Med 2016;16:95. [Crossref] [PubMed]
- Hartman JE, Klooster K, Slebos DJ, et al. Improvement of physical activity after endobronchial valve treatment in emphysema patients. Respir Med 2016;117:116-21. [Crossref] [PubMed]
- Estenne M. Effect of lung transplant and volume reduction surgery on respiratory muscle function. J Appl Physiol (1985) 2009;107:977-86. [PubMed]
- Benditt JO, Lewis S, Wood DE, et al. Lung volume reduction surgery improves maximal O2 consumption, maximal minute ventilation, O2 pulse, and dead space-to-tidal volume ratio during leg cycle ergometry. Am J Respir Crit Care Med 1997;156:561-6. [Crossref] [PubMed]
- Payen JF, Wuyam B, Levy P, et al. Muscular metabolism during oxygen supplementation in patients with chronic hypoxemia. Am Rev Respir Dis 1993;147:592-8. [Crossref] [PubMed]
- Demelo-Rodríguez P, Olmedo Samperio M, Gaitán Tocora DG, et al. High-flow nasal cannula oxygen therapy: preliminary study in hospitalized patients. Arch Bronconeumol 2015;51:657-9. [Crossref] [PubMed]
- Vogiatzis I, Athanasopoulos D, Habazettl H, et al. Intercostal muscle blood flow limitation during exercise in chronic obstructive pulmonary disease. Am J Respir Crit Care Med 2010;182:1105-13. [Crossref] [PubMed]
- Rodríguez A, Claverias L, Marín J, et al. Regional oxygen saturation index (rSO2) in brachioradialis and deltoid muscle. Correlation and prognosis in patients with respiratory sepsis. Med Intensiva 2015;39:68-75. [Crossref] [PubMed]
- Goldberg AL, Goodman HM. Relationship between cortisone and muscle work in determining muscle size. J Physiol 1969;200:667-75. [Crossref] [PubMed]
- Decramer M, de Bock V, Dom R. Functional and histologic picture of steroid-induced myopathy in chronic obstructive pulmonary disease. Am J Respir Crit Care Med 1996;153:1958-64. [Crossref] [PubMed]
- Marcos PJ, Huerta A, Márquez-Martín E. 2014 GesEPOC and systemic steroids in chronic obstructive pulmonary disease (COPD) exacerbation: an update. Arch Bronconeumol 2015;51:360. [PubMed]
- Hill AT, Campbell EJ, Hill SL, et al. Association between airway bacterial load and markers of airway inflammation in patients with stable chronic bronchitis. Am J Med 2000;109:288-95. [Crossref] [PubMed]
- Vermeeren MA, Schols AM, Wouters EF. Effects of an acute exacerbation on nutritional and metabolic profile of patients with COPD. Eur Respir J 1997;10:2264-9. [Crossref] [PubMed]
- Pitta F, Troosters T, Probst VS, et al. Physical activity and hospitalization for exacerbation of COPD. Chest 2006;129:536-44. [Crossref] [PubMed]
- Nava S, Ambrosino N, Rubini F, et al. Effect of nasal pressure support ventilation and external PEEP on diaphragmatic activity in patients with severe stable COPD. Chest 1993;103:143-50. [Crossref] [PubMed]
- Renston JP, DiMarco AF, Supinski GS. Respiratory muscle rest using nasal BiPAP ventilation in patients with stable severe COPD. Chest 1994;105:1053-60. [Crossref] [PubMed]
- Ambrosino N, Vagheggini G. Non-invasive ventilation in exacerbations of COPD. Int J Chron Obstruct Pulmon Dis 2007;2:471-6. [PubMed]
- Plant PK, Owen JL, Elliott MW. Early use of non-invasive ventilation for acute exacerbations of chronic obstructive pulmonary disease on general respiratory wards: a multicentre randomised controlled trial. Lancet 2000;355:1931-5. [Crossref] [PubMed]
- McCurdy BR. Hospital-at-home programs for patients with acute exacerbations of chronic obstructive pulmonary disease (COPD): an evidence-based analysis. Ont Health Technol Assess Ser 2012;12:1-65. [PubMed]
- Moretti M, Cilione C, Tampieri A, et al. Incidence and causes of non-invasive mechanical ventilation failure after initial success. Thorax 2000;55:819-25. [Crossref] [PubMed]
- Köhnlein T, Windisch W, Köhler D, et al. Non-invasive positive pressure ventilation for the treatment of severe stable chronic obstructive pulmonary disease: a prospective, multicentre, randomised, controlled clinical trial. Lancet Respir Med 2014;2:698-705. [Crossref] [PubMed]
- Clini E, Sturani C, Porta R, et al. Outcome of COPD patients performing nocturnal non-invasive mechanical ventilation. Respir Med 1998;92:1215-22. [Crossref] [PubMed]
- Squadrone E, Frigerio P, Fogliati C, et al. Noninvasive vs. invasive ventilation in COPD patients with severe acute respiratory failure deemed to require ventilatory assistance. Intensive Care Med 2004;30:1303-10. [Crossref] [PubMed]
- Hussain SN, Cornachione AS, Guichon C, et al. Prolonged controlled mechanical ventilation in humans triggers myofibrillar contractile dysfunction and myofilament protein loss in the diaphragm. Thorax 2016;71:436-45. [Crossref] [PubMed]
- Powers SK, Wiggs MP, Duarte JA, et al. Mitochondrial signaling contributes to disuse muscle atrophy. Am J Physiol Endocrinol Metab 2012;303:E31-9. [Crossref] [PubMed]
- Dot I, Pérez-Teran P, Samper MA, et al. Diaphragm Dysfunction in Mechanically Ventilated Patients. Arch Bronconeumol 2016. [Epub ahead of print]. [PubMed]
- Portillo K, Abad-Capa J, Ruiz-Manzano J. Chronic obstructive pulmonary disease and left ventricle. Arch Bronconeumol 2015;51:227-34. [PubMed]
- Rehn TA, Munkvik M, Lunde PK, et al. Intrinsic skeletal muscle alterations in chronic heart failure patients: a disease-specific myopathy or a result of deconditioning? Heart Fail Rev 2012;17:421-36. [Crossref] [PubMed]
- Puig-Vilanova E, Rodriguez DA, Lloreta J, et al. Oxidative stress, redox signaling pathways, and autophagy in cachectic muscles of male patients with advanced COPD and lung cancer. Free Radic Biol Med 2015;79:91-108. [Crossref] [PubMed]
- Morley JE. Sarcopenia in the elderly. Fam Pract 2012;29 Suppl 1:i44-i48. [Crossref] [PubMed]
- Budui SL, Rossi AP, Zamboni M. The pathogenetic bases of sarcopenia. Clin Cases Miner Bone Metab 2015;12:22-6. [PubMed]
- Denison HJ, Cooper C, Sayer AA, et al. Prevention and optimal management of sarcopenia: a review of combined exercise and nutrition interventions to improve muscle outcomes in older people. Clin Interv Aging 2015;10:859-69. [PubMed]
- van Hees HW, Dekhuijzen PN, Heunks LM. Levosimendan enhances force generation of diaphragm muscle from patients with chronic obstructive pulmonary disease. Am J Respir Crit Care Med 2009;179:41-7. [Crossref] [PubMed]
- Doorduin J, Sinderby CA, Beck J, et al. The calcium sensitizer levosimendan improves human diaphragm function. Am J Respir Crit Care Med 2012;185:90-5. [Crossref] [PubMed]
- Chacon-Cabrera A, Fermoselle C, Urtreger AJ, et al. Pharmacological strategies in lung cancer-induced cachexia: effects on muscle proteolysis, autophagy, structure, and weakness. J Cell Physiol 2014;229:1660-72. [Crossref] [PubMed]
- Koike A, Kobayashi K, Adachi H, et al. Effects of dobutamine on critical capillary PO2 and lactic acidosis threshold in patients with cardiovascular disease. Chest 2001;120:1218-25. [Crossref] [PubMed]
- Mancini DM, Schwartz M, Ferraro N, et al. Effect of dobutamine on skeletal muscle metabolism in patients with congestive heart failure. Am J Cardiol 1990;65:1121-6. [Crossref] [PubMed]
- Hagström-Toft E, Enoksson S, Moberg E, et al. Beta-Adrenergic regulation of lipolysis and blood flow in human skeletal muscle in vivo. Am J Physiol 1998;275:E909-16. [PubMed]
- Stoller JK, Wiedemann HP, Loke J, et al. Terbutaline and diaphragm function in chronic obstructive pulmonary disease: a double-blind randomized clinical trial. Br J Dis Chest 1988;82:242-50. [Crossref] [PubMed]
- Greig CA, Johns N, Gray C, et al. Phase I/II trial of formoterol fumarate combined with megestrol acetate in cachectic patients with advanced malignancy. Support Care Cancer 2014;22:1269-75. [Crossref] [PubMed]
- Ryall JG, Lynch GS. The potential and the pitfalls of beta-adrenoceptor agonists for the management of skeletal muscle wasting. Pharmacol Ther 2008;120:219-32. [Crossref] [PubMed]
- Kamalakkannan G, Petrilli CM, George I, et al. Clenbuterol increases lean muscle mass but not endurance in patients with chronic heart failure. J Heart Lung Transplant 2008;27:457-61. [Crossref] [PubMed]
- Barberan-Garcia A, Rodríguez DA, Blanco I, et al. Non-anaemic iron deficiency impairs response to pulmonary rehabilitation in COPD. Respirology 2015;20:1089-95. [Crossref] [PubMed]
- Stugiewicz M, Tkaczyszyn M, Kasztura M, et al. The influence of iron deficiency on the functioning of skeletal muscles: experimental evidence and clinical implications. Eur J Heart Fail 2016;18:762-73. [Crossref] [PubMed]
- McGuire M, Carey MF, O'Connor JJ. Almitrine and doxapram decrease fatigue and increase subsequent recovery in isolated rat diaphragm. J Appl Physiol (1985) 1997;83:52-8. [PubMed]
- McGuire M, Cantillon D, Bradford A. Effects of almitrine on diaphragm contractile properties in young and old rats. Respiration 2002;69:75-80. [Crossref] [PubMed]
- Aubier M. Effect of theophylline on diaphragmatic and other skeletal muscle function. J Allergy Clin Immunol 1986;78:787-92. [Crossref] [PubMed]