In vitro effects of N-acetylcysteine alone and combined with tigecycline on planktonic cells and biofilms of Acinetobacter baumannii
Introduction
Acinetobacter baumannii (A. baumannii) is a common opportunistic pathogen among intensive care unit patients implicated in diverse nosocomial infections, including pneumonia, meningitis, bacteremia, urinary tract infection, and wound infection (1). Therapeutic options are limited for patients who are infected with this pathogen due to the increased resistant to commonly used antimicrobial agents (2). Biofilm formation is one mechanism for the bacteria to acquire resistance to most of the antibiotics (3-6); whereas, traditional antibiotics have restricted effects on preventing biofilm formation due to the failure to reach high serum concentration and tissue concentration (7-9). Previous research has demonstrated that A. baumannii had strong ability to form biofilms (10). Under the protection of biofilms, A. baumannii can survive under adverse circumstances and further causes severe infections in various tissues and organs (11). Therefore, it is necessary and urgent to explore a novel strategy against biofilm-associated infections.
N-acetylcysteine (NAC) is a powerful thiol-containing antioxidant, which serves as a precursor of glutathione synthesis (12). In addition, NAC has been demonstrated as an alternative drug to control biofilm growth of several important clinical bacteria, including Pseudomonas aeruginosa (13), Streptococcus pneumoniae (14), Staphylococcus epidermidis (15,16), Escherichia coli (7), and Enterococcus faecalis (17,18). Tigecycline (TGC) is a broad-spectrum antibiotic with activity against some difficult-to-treat pathogens, such as methicillin-resistant Staphylococcus aureus, vancomycin-resistant Enterococcus spp. and A. baumannii (19). The combination of NAC and TGC has been shown to synergistically inhibit biofilm-associated bacterial infections, including methicillin-resistant Staphylococcus aureus and Staphylococcus epidermidis (20). However, the effects of NAC and TGC co-treatment on A. baumannii have been rarely studied. The effects of NAC and TGC co-treatment on planktonic cells and biofilms of A. baumannii are still not clear.
In this study, we examined the effects of NAC and TGC against planktonic cells and biofilm formation of 25 A. baumannii clinical isolates in vitro. The combined effects of NAC and TGC on biofilm-embedded bacteria were also tested on 15 biofilm-producing isolates. We found that NAC alone or combined with TGC significantly inhibited planktonic cells and biofilms of A. baumannii, which provided a potential therapeutic strategy for biofilm-associated A. baumannii infections.
Methods
Bacterial strains
Twenty-five clinical strains of A. baumannii were isolated from patients at the First Affiliated Hospital of Sun Yat-sen University (Guangzhou, China) in 2011. A. baumannii ATCC17978 and Escherichia coli ATCC25922 were used as the reference strains. Isolates were identified as A. baumannii by VITEK 2 System (BioMérieux Inc., France).
Biofilm production
Twenty-five A. baumannii clinical isolates were used for the evaluation of biofilm production. Biofilm production was quantified by the spectrophotometry as previously described (10). Overnight cultures of A. baumannii in fresh Luria-Bertani broth (LB broth, Oxoid Ltd., Basingstoke, UK) were standardized to a 0.5 MacFarland standard (1.5×108 CFU/mL). Aliquots of standardized inoculums were added to sterile 96-well culture plates (Thermo Fisher Scientific, USA) and incubated at 37 °C for 72 h. After stained with crystal violet (Sigma, USA) 0.01% (w/v) for 20 min, biofilms were washed and extracted with 33% (v/v) glacial acetic acid (Sigma, USA). The amount of biofilm was quantified by measuring the optical density at 570 nm (OD570) of dissolved crystal violet. Fresh sterile LB broth was used as negative control. All measurements were performed in triplicate and independently repeated for three times. The cut-off OD (ODC) was defined as three standard deviations (SD) above the mean OD of negative control. Criteria of explanation of biofilm production are as follows: negative biofilm producer (OD ≤ODc), weak biofilm producer (ODc < OD ≤2× ODc), moderate biofilm producer (2× ODc < OD ≤4× ODc), strong biofilm producer (OD >4× ODc) (21).
Determination of the minimum inhibitory concentration (MIC) and MIC in biofilm (MIC-b) of NAC
MICs and MIC-bs of NAC (Fluimucil; Zambon S.p.A, Italy) for A. baumannii ATCC17978 and 25 clinical isolates were tested in these experiments. The MICs of NAC were determined by the broth microdilution method, according to Clinical and Laboratory Standards Institute (CLSI) guidelines [2016]. The MIC-bs of NAC were detected as previously described (22). Briefly, aliquots of the standardized inoculums (1.5×108 CFU/mL) were incubated in 96-well microplates at 37 °C for 72 h. The wells were washed with PBS (Thermo Fisher Scientific, USA) and NAC at two-fold dilutions (0.5–128 mg/mL) was added to the preformed biofilms. After an overnight incubation, MIC-b was determined as the lowest concentration of NAC in which a planktonic bacterial population could not be established by shedding of bacteria from the biofilms. A. baumannii ATCC17978 was used as quality control. Sterility control and NAC-free control were included in all experiments.
Effects of NAC on biofilm formation and preformed biofilms of biofilm-producing A. baumannii
Ten A. baumannii clinical isolates, which were biofilm producers (mean OD > ODC), were chosen randomly to evaluate the effects of NAC on biofilm formation and preformed biofilms, as previously described (23). Standardized planktonic suspensions (1.5×108 CFU/mL) were treated with NAC (0.5–64 mg/mL) at 37 °C for 24 h. The extent of biofilm formation was quantified by measuring OD570 of dissolved crystal violet. To test the effect of NAC on preformed biofilms, aliquots of standardized inoculums (1.5×108 CFU/mL) were incubation at 37 °C for 72 h and washed with PBS to eliminate planktonic bacteria. Followed with a 24 h exposure of NAC (0.5–128 mg/mL), biofilms were quantified by measuring OD570 of dissolved crystal violet. NAC-free controls were included in all experiments.
Scanning electron microscopy (SEM) analysis was also used to observe the effect of NAC on preformed biofilms, as previously described (24). Briefly, the biofilms were formed on cover slips in sterile, flat-bottomed 6-well culture plates (Thermo Fisher Scientific, USA) at 37 °C for 72 h. The cover slips were then washed with sterile PBS, and NAC (from 4 to 0.5 mg/mL) was added to the wells. After 24 h incubation at 37 °C, the cover slips were rinsed with PBS and fixed with 2.5% v/v glutaraldehyde (Sigma, USA) for at least 4 h. After washed with PBS, the samples were then dehydrated in aqueous ethanol solutions. The specimens were mounted on aluminum stubs, allowed to dry for 3 h, and then coated with 15 nm Au film. Samples were observed with a Philips XL30CP scanning electron microscope in the high-vacuum mode at 15 kV.
Interactive effects of NAC and TGC in vitro
According to assessed MIC of NAC and TGC (Hisun Pharma, China) for each isolates, standardized suspensions (1.5×108 CFU/mL) were incubated with NAC (0.5–32 mg/mL) and TGC (0.0625–4 µg/mL) at 37 °C for 16–20 h. The antibacterial effects of NAC and TGC were evaluated by checkerboard MIC assays (25). Positive and negative controls (no antibiotic and no inoculum, respectively) were included in all plates.
The fractional inhibitory concentration index (FICI) was calculated using the following formula: FICI = FICNAC + FICTGC, where FICNAC = MIC of NAC in combination/MIC of NAC alone, and FICTGC = MIC of TGC in combination/MIC of TGC alone. The antibacterial effect was defined as follows: synergism (FICI ≤0.5), partial synergism (0.5< FICI <1), additivity (FICI =1), indifference (1< FICI <4), and antagonism (FICI ≥4) (26,27).
Combined effect of NAC and TGC on biofilm-embedded bacteria
As previously described (22), combined effect of NAC and TGC on A. baumannii biofilm-embedded bacteria was tested on 15 randomly selected strains within biofilm producers. Briefly, NAC (0, 4, 8, 16, 32 mg/mL) in combination with TGC (0, 0.5, 1, 2, 4 µg/mL) were added to preformed biofilms of the selected strains. After 24 h exposure, the wells were washed and scraped with sterile cotton swabs. Swabs were transferred into tubes containing PBS buffer and sonicated for 15 mins. The number of viable cells was evaluated by plating serial dilutions of these suspensions on LB agar plates.
Statistical analysis
Results were expressed as the mean ± SD. One-way analysis of variance was used to detect the existence of differences among different groups, followed by Dunnett’s test for multiple comparisons. All P values were based on two-tailed tests of significance, and a significance level of 0.05 or 0.01 was used. Statistical analysis of results was conducted with SPSS 17.0 software (IBM, USA).
Results
MICs and MIC-bs of NAC
Among 25 A. baumannii clinical isolates, MICs of NAC ranged from 16 to 128 mg/mL. The 50% MIC (MIC50) of NAC was 32 mg/mL, and the 90% MIC (MIC90) of NAC was 128 mg/mL. The MIC-bs of NAC ranged from 32 to 256 mg/mL. The 50% MIC-b (MIC50-b) of NAC was 64 mg/mL, and the 90% MIC-b (MIC90-b) of NAC was 256 mg/mL.
Biofilm production and effects of NAC on biofilm formation and preformed biofilms
Biofilm producing abilities of 25 A. baumannii clinical strains are as follows: three (12%) strains were negative biofilm producers, seven (28%) strains were weak biofilm producers, 9 (36%) strains were moderate biofilm producers, and 6 (24%) strains were strong biofilm producers.
As shown in Figure 1, NAC dose-dependently inhibited biofilm formation and removed mature biofilms of A. baumannii. In addition, SEM analysis was performed on one A. baumannii clinical isolate with strong biofilm-producing ability and relatively low MIC of NAC (16 mg/mL). Compared with control, NAC significantly reduced viable cell counts, attenuated bacterial aggregation and decreased amount of biofilm mass (Figure 2). NAC at 4 mg/mL induced a scarce distribution of bacteria and prevented colonies and biofilm formation (Figure 2E).
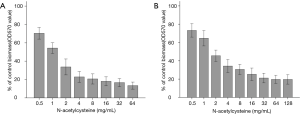
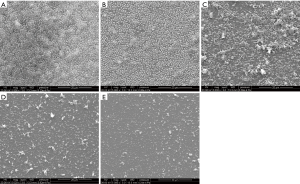
Combined effects of NAC and TGC on planktonic cells and biofilm-embedded bacteria
In 25 A. baumannii clinical isolates, combined effects of NAC and TGC on planktonic cells are listed as follows: synergism for 2 strains (8%; 1 negative and 1 moderate biofilm producers); partial synergism for 15 strains (60%; 1 negative, 6 weak, 5 moderate and 3 strong biofilm producers); additivity for 7 strains (28%; 1 weak, 3 moderate and 3 strong biofilm producers); indifference for 1 strain (4%; 1 negative biofilm producer). No antagonism was observed.
Viable cell counts of biofilm-embedded bacteria treated with NAC and TGC are shown in Table 1. Compared with control, 16 mg/mL NAC alone or 2 µg/mL TGC alone induced significant bactericidal effects (P<0.01 and P<0.05, respectively). Co-treatment of NAC and TGC consistently reduced viable cell counts in biofilm; synergistic bactericidal effect was found at 4 mg/mL NAC and 0.5 µg/mL TGC (P<0.01).
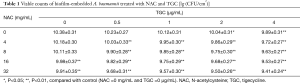
Full table
Discussion
In this study, antibacterial and anti-biofilm effects of NAC alone and combined with TGC against A. baumanni were analyzed. Our results revealed that NAC alone significantly inhibited biofilm formation of A. baumannii. The combination of NAC and TGC induced partial synergistic effect against planktonic cells and synergistic effect against biofilm-embedded A. baumannii.
Bacteria that grow in biofilms are extremely difficult to eradicate because of the intrinsic resistance provided by the biofilms to multiple antimicrobial agents and products of the immune system. In these circumstances, MICs are unrelated with the treatment outcomes (8). The utilization of NAC has been considered as an alternative approach to control bacterial biofilms in human diseases. Previous studies have shown that NAC inhibited biofilm formation and disrupted mature biofilms of various bacteria, including E. coli, Staphylococcus epidermidis, etc. (7,15). In the current study, we found that 22 out of 25 strains of A. baumannii were capable to produce biofilms. And ODs of biofilms ranged from 0.850 to 4.637, indicating that the activities to form biofilms were different among isolates. We demonstrated that NAC inhibited biofilm formation and removed mature biofilms of A. baumannii in a concentration-dependent manner, which was further confirmed by SEM analysis. Besides, NAC can act as an independent anti-microbial agent on biofilm-embedded A. baumannii at 16 mg/mL (P<0.01). The concentrations of NAC in this study were higher than those reach in serum under intravenous or oral administration. Even so, useful concentrations to disrupt biofilms may be obtained by aerosol inhalation.
TGC was considered as a last-resort option against severe infections caused by multi-drug resistant A. baumannii (28). And recent studies have shown that TGC significantly inhibited biofilm formation of A. baumannii (29). In this study, MICs of TGC for the isolates ranged from 0.125 to 2 µg/mL. In clinical practice, the serum concentration of TGC is about 0.72 µg/mL (30). Although concentration in alveolar cells reaches about 3.7 times higher than that in serum, it is still lower than the highest concentration used in our study, which means achievable serum concentration and tissue concentration are included in this study. Interestingly, though TGC is concentrated in tissues, the maximum concentration in epithelial lining fluid is only 0.37 µg/mL (30), which makes co-treatment of TGC and other antimicrobial agents necessary.
The combination of NAC with TGC induced partial synergistic effects for most of A. baumannii strains collected in our study. The MIC50, MIC90 and MICrange (data not shown) of NAC and TGC co-treatment against the A. baumannii strains were obviously lower than that of NAC or TGC alone. Using the same method as previous described (13), we demonstrated that combination of NAC and TGC at lower concentrations also significantly decreased the viable cell counts in biofilms. So the NAC-TGC combination may be a considerable option in biofilm-related A. baumannii infections.
The screening for the effectiveness of clinical drugs may provide clinical strategy to improve treatment outcomes of biofilm related infections and reduce hospitalization days. Our study demonstrated that the synergistic effects of combination of NAC and TGC against planktonic and biofilm-embedded A. baumannii. In addition, we found that NAC alone prevent biofilm related infections of A. baumannii. Still, experiments on the antimicrobial mechanism of NAC may provide deeper insight into the treatment of A. baumannii infections and interactive effects of NAC and TGC in vivo need to be addressed in the further study. Overall, this study is the first to demonstrate the synergistic effects of NAC and TGC co-treatment, which provides a clinically relevant argument for using NAC and TGC combination against A. baumannii infections.
Acknowledgements
Funding: This work was supported by grants from the National Natural Science Foundation of China (81570008 to Y Zhou), the Natural Science Foundation of Guangdong Province of China (2014A030313052 to Y Zhou), and Science and Technology Program of Guangzhou, China (2014J4100132 to Y Zhou).
Footnote
Conflicts of Interest: The authors have no conflicts of interest to declare.
References
- Dijkshoorn L, Nemec A, Seifert H. An increasing threat in hospitals: multidrug-resistant Acinetobacter baumannii. Nat Rev Microbiol 2007;5:939-51. [Crossref] [PubMed]
- Maragakis LL, Perl TM. Acinetobacter baumannii: epidemiology, antimicrobial resistance, and treatment options. Clin Infect Dis 2008;46:1254-63. [Crossref] [PubMed]
- Qi L, Li H, Zhang C, et al. Relationship between Antibiotic Resistance, Biofilm Formation, and Biofilm-Specific Resistance in Acinetobacter baumannii. Front Microbiol 2016;7:483. [Crossref] [PubMed]
- He X, Lu F, Yuan F, et al. Biofilm Formation Caused by Clinical Acinetobacter baumannii Isolates Is Associated with Overexpression of the AdeFGH Efflux Pump. Antimicrob Agents Chemother 2015;59:4817-25. [Crossref] [PubMed]
- Mah TF, O'Toole GA. Mechanisms of biofilm resistance to antimicrobial agents. Trends Microbiol 2001;9:34-9. [Crossref] [PubMed]
- Mah TF. Biofilm-specific antibiotic resistance. Future Microbiol 2012;7:1061-72. [Crossref] [PubMed]
- Marchese A, Bozzolasco M, Gualco L, et al. Effect of fosfomycin alone and in combination with N-acetylcysteine on E. coli biofilms. Int J Antimicrob Agents 2003;22 Suppl 2:95-100. [Crossref] [PubMed]
- Kim HA, Ryu SY, Seo I, et al. Biofilm Formation and Colistin Susceptibility of Acinetobacter baumannii Isolated from Korean Nosocomial Samples. Microb Drug Resist 2015;21:452-7. [Crossref] [PubMed]
- Yang YS, Lee Y, Tseng KC, et al. In Vivo and In Vitro Efficacy of Minocycline-Based Combination Therapy for Minocycline-Resistant Acinetobacter baumannii. Antimicrob Agents Chemother 2016;60:4047-54. [Crossref] [PubMed]
- Rodríguez-Baño J, Martí S, Soto S, et al. Biofilm formation in Acinetobacter baumannii: associated features and clinical implications. Clin Microbiol Infect 2008;14:276-8. [Crossref] [PubMed]
- Jefferson KK. What drives bacteria to produce a biofilm? FEMS Microbiol Lett 2004;236:163-73. [Crossref] [PubMed]
- Kaplan M, Atakan IH, Aydogdu N, et al. Influence of N-acetylcysteine on renal toxicity of cadmium in rats. Pediatr Nephrol 2008;23:233-41. [Crossref] [PubMed]
- Zhao T, Liu Y. N-acetylcysteine inhibit biofilms produced by Pseudomonas aeruginosa. BMC Microbiol 2010;10:140. [Crossref] [PubMed]
- del Prado G, Ruiz V, Naves P, et al. Biofilm formation by Streptococcus pneumoniae strains and effects of human serum albumin, ibuprofen, N-acetyl-l-cysteine, amoxicillin, erythromycin, and levofloxacin. Diagn Microbiol Infect Dis 2010;67:311-8. [Crossref] [PubMed]
- Pérez-Giraldo C, Rodriguez-Benito A, Moran FJ, et al. Influence of N-acetylcysteine on the formation of biofilm by Staphylococcus epidermidis. J Antimicrob Chemother 1997;39:643-6. [Crossref] [PubMed]
- Leite B, Gomes F, Teixeira P, et al. Combined effect of linezolid and N-acetylcysteine against Staphylococcus epidermidis biofilms. Enferm Infecc Microbiol Clin 2013;31:655-9. [Crossref] [PubMed]
- Silveira LF, Baca P, Arias-Moliz MT, et al. Antimicrobial activity of alexidine alone and associated with N-acetylcysteine against Enterococcus faecalis biofilm. Int J Oral Sci 2013;5:146-9. [Crossref] [PubMed]
- Quah SY, Wu S, Lui JN, et al. N-acetylcysteine inhibits growth and eradicates biofilm of Enterococcus faecalis. J Endod 2012;38:81-5. [Crossref] [PubMed]
- Stein GE, Babinchak T. Tigecycline: an update. Diagn Microbiol Infect Dis 2013;75:331-6. [Crossref] [PubMed]
- Aslam S, Trautner BW, Ramanathan V, et al. Combination of tigecycline and N-acetylcysteine reduces biofilm-embedded bacteria on vascular catheters. Antimicrob Agents Chemother 2007;51:1556-8. [Crossref] [PubMed]
- Stepanović S, Vukovic D, Hola V, et al. Quantification of biofilm in microtiter plates: overview of testing conditions and practical recommendations for assessment of biofilm production by staphylococci. APMIS 2007;115:891-9. [Crossref] [PubMed]
- Passerini de Rossi B, Garcia C, Calenda M, et al. Activity of levofloxacin and ciprofloxacin on biofilms and planktonic cells of Stenotrophomonas maltophilia isolates from patients with device-associated infections. Int J Antimicrob Agents 2009;34:260-4. [Crossref] [PubMed]
- Pitts B, Hamilton MA, Zelver N, et al. A microtiter-plate screening method for biofilm disinfection and removal. J Microbiol Methods 2003;54:269-76. [Crossref] [PubMed]
- Di Bonaventura G, Spedicato I, D'Antonio D, et al. Biofilm formation by Stenotrophomonas maltophilia: modulation by quinolones, trimethoprim-sulfamethoxazole, and ceftazidime. Antimicrob Agents Chemother 2004;48:151-60. [Crossref] [PubMed]
- Rand KH, Houck HJ, Brown P, et al. Reproducibility of the microdilution checkerboard method for antibiotic synergy. Antimicrob Agents Chemother 1993;37:613-5. [Crossref] [PubMed]
- Bai Y, Liu B, Wang T, et al. In Vitro activities of combinations of rifampin with other antimicrobials against multidrug-resistant Acinetobacter baumannii. Antimicrob Agents Chemother 2015;59:1466-71. [Crossref] [PubMed]
- Sader HS, Huynh HK, Jones RN. Contemporary in vitro synergy rates for aztreonam combined with newer fluoroquinolones and beta-lactams tested against gram-negative bacilli. Diagn Microbiol Infect Dis 2003;47:547-50. [Crossref] [PubMed]
- Michalopoulos A, Falagas ME. Treatment of Acinetobacter infections. Expert Opin Pharmacother 2010;11:779-88. [Crossref] [PubMed]
- Song JY, Cheong HJ, Noh JY, et al. In vitro Comparison of Anti-Biofilm Effects against Carbapenem-Resistant Acinetobacter baumannii: Imipenem, Colistin, Tigecycline, Rifampicin and Combinations. Infect Chemother 2015;47:27-32. [Crossref] [PubMed]
- Conte JE Jr, Golden JA, Kelly MG, et al. Steady-state serum and intrapulmonary pharmacokinetics and pharmacodynamics of tigecycline. Int J Antimicrob Agents 2005;25:523-9. [Crossref] [PubMed]