Is there still a role for alveolar recruitment maneuvers in acute respiratory distress syndrome?
An alveolar recruitment maneuver (ARM) involves the transient application of high transpulmonary pressures to achieve threshold opening pressures in collapsed peripheral airways and alveoli. The expectation of ARMs is to improve oxygenation, lung compliance and potentially facilitate alveolar fluid clearance (1,2). Although the origins of ARM as a clinical tool were for treating intra- or post-operative atelectasis (3), beginning in the 1990’s ARM became a central feature of “open-lung ventilation” (OLV), a lung-protective ventilation strategy for managing patients with acute respiratory distress syndrome (ARDS) (4).
The physiologic rationale for utilizing ARM is that ARDS is characterized by decreased functional residual capacity from (I) underinflated alveoli that are vulnerable to collapse, and (II) atelectatic or “de-recruited” alveoli and peripheral airways (5). Severe ARDS often reflects the contribution of enormous compressive forces emanating from reduced chest wall compliance (6). Moreover, the primary basis for OLV is the belief that “atelectrauma” from repetitive collapse and reopening of peripheral airspaces is one of the main drivers of ventilator-induced lung injury (VILI) in severe ARDS.
Since OLV was first described numerous publications on ARM have appeared in the medical literature (7). Fully recruiting dorsal-caudal lung regions in ARDS requires the transient application of inspiratory plateau pressures (Pplat) ranging between 40–60 cmH2O (Pplat being a correlate of peak alveolar pressure, and therefore threshold opening pressure). Although the physiologic principles underpinning the efficacy of ARM are well established, its application in ARDS remains controversial. This is because ARDS presents uniquely in different patients, and the patterns of lung injury are heterogeneous within individual patients. Most importantly it is difficult clinically to ascertain how much lung tissue is potentially recruitable versus consolidated. Phrased differently: do we cause more harm from stretch-related injury by using ARM than we gain from reducing shear-related injury? Another unsolved issue is whether “atelectrauma” contributes substantially to lung injury and mortality in ARDS when relatively higher levels of PEEP are used?
The recently published study by the ART investigators is the largest prospective randomized-controlled trial ever (having enrolled 1,010 subjects) to examine the role of ARM in ARDS (8). The study compared OLV to the ventilator protocol from the seminal “ARMA” trial by the NIH ARDS Network (9). Randomization occurred within 72 h onset of moderate or severe ARDS.
The OLV strategy included an initial ARM using brief (1–2 min) step-wise application of positive end-expiratory pressures (PEEP) of 25, 35 and 45 cmH2O with a driving pressure (∆P) of 15 cmH2O to achieve a maximal Pplat of 60 cmH2O. Afterwards, end-expiratory pressure was determined using a decremental PEEP trial based on best compliance. This was followed by a second ARM before resuming low tidal volume (VT) ventilation of 6 mL/kg.
The OLV strategy resulted in higher mortality compared to the ARMA strategy both at study day 28 (55.3 vs. 49.3% respectively, P=0.041) and at hospital discharge (63.8 vs. 59.3% respectively, P=0.15). This contradicted the results of both a recent pilot study demonstrating no mortality difference between OLV and ARMA strategies (29 vs. 33% respectively, P=0.18) (10), as well as a recent meta-analysis that concluded (albeit with limited confidence due to study heterogeneity) that OLV reduces mortality risk by approximately 20% (7).
Evaluating the ART study results will require further evaluation of more granular data that has not yet been made public. The study appears to have been well designed, and baseline characteristics of each arm were well balanced. Of note, data on the incidence of protocol violations between study arms were not reported. Certain aspects of the study, however, raise questions related to comorbidities and overall mortality, the impact of ARM-related serious adverse events on additional mortality, the possibility of subtler deleterious effects of ARM on mortality in general, and a re-evaluation on the degree to which atelectrauma contributes to VILI in the era of lung protective ventilation.
First, for subjects eligible for enrollment into a randomized controlled clinical trial there was a strikingly high mortality in both treatment arms. The exclusion criteria used in the ART study suggests a primary focus on contraindications to the safe performance of ARM (e.g., hemodynamic instability, presence of barotrauma, and intracranial hypertension). In contrast, similarly large mechanical ventilation trials in ARDS (9,11) excluded those with substantial mortality risks (e.g., severe chronic liver failure, bone marrow transplant, metastatic disease, severe burns, etc.). If subjects with these comorbidities were allowed to be enrolled into ART, it might explain the overall higher mortality rate and enhanced mortality risk seen in the OLV arm.
Second, halfway through the study, the ARM procedure was amended to lower the targeted Pplat from 60 to 50 cmH2O, because of 3 cardiac arrests associated with the procedure. During the first 7 days there were 30 more deaths in the OLV group, wherein the only significant differences between the groups were the development of barotrauma and the initiation/acceleration of vasopressors. The incidence of barotrauma during the first week was 28 (5.6%) vs. 8 (1.6%) subjects for OLV and ARMA treatment arms respectively, and the associated mortality was 7 vs. 0 respectively. Over the course of the trial, hemodynamic instability caused 75% of the incidences resulting in premature ARM termination.
The finding of no significant difference in “treatment effects” before and after ARM modification suggests a more subtle impact of OLV on mortality was present. Approximately 63% of the OLV group had direct lung injury (predominantly pneumonia), which is believed to be less amenable to recruitment because lung consolidation is the primary lesion (12). When applying ARM there is a point at which increasing Pplat produces only minor improvements in recruitment. This likely comes at the cost of increased risk of stretch-related injury. A curious side note is that the ART study found no difference in the need for rescue therapies between treatment arms; as might be expected if ARMs effected substantial recruitment and alveolar stability. This particular result is at odds with the findings of the recent meta-analysis (7).
The above noted complications in the OLV group are consistent with classic signs of PEEP-induced alveolar overdistension: decreased compliance and decreased cardiac output. Moreover, a study of severe ARDS reported that increasing Pplat from 50 to 60 cmH2O caused the amount of non-aerated lung tissue to decrease from a marginal 10% to 5% (13) (Figure 1). Thus, one can question the wisdom of an ARM strategy wherein a Pplat >50 cmH2O is the primary treatment target (e.g., rather than a protocolized, secondary target when the initial maneuver is found to be ineffective).
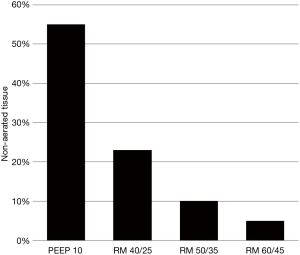
Of particular concern is the seemingly high number of subjects (N=46, 9.2%) in the OLV arm who received multiple, additional ARMs (≥3) in a study cohort characterized by direct lung injury (63%) and septic shock (66%). Over time, repetitive ARMs may have exacerbated the hyperinflammatory state by potentially causing regional lung overdistension, bacterial translocation and repeated gastrointestinal ischemia/reperfusion injury (14,15).
In animal models of pneumonia, bacterial translocation occurs irrespective of the particular recruitment strategy employed (14). Persistent depression of mesenteric perfusion has also been reported despite the return of systemic blood pressure towards pre-recruitment levels (15). In regard to the latter, it’s worth noting that a significantly higher percentage of subjects randomized to the OLV arm required either initiation or increase in vasopressor support within 1 h of randomization (34.8% vs. 28.3%, P=0.03).
It bears mentioning that increased mortality in the OLV group is unlikely attributable to the higher mean PEEP levels compared to the ARMA group as it was only 3–4 cmH2O; whereas mean PEEP differences of 6–8 cmH2O were reported in 3 previous major lung protective ventilation studies of higher PEEP (EXPRESS, ALVEOLI, and LOVS). In these studies, the mortality was not different between treatment arms and was substantially lower than that reported in the ART study (11,16,17).
Interpreting mortality in the ART study is difficult. The most reasonable approach is to compare outcomes with the experimental arms in similar trials (11,16-18) (Table 1). It appears that those in the ART study had somewhat worse pulmonary function at enrollment characterized by a lower mean arterial oxygen tension-to-inspired oxygen fraction (PaO2/FiO2) on a modestly higher PEEP level. However, following enrollment, subjects in the ART study had a higher PaO2/FiO2 on a similar PEEP and lower VT, Pplat, and ∆P compared to those previous trials. These findings invite the expectation of improved (or at least equivalent) outcomes with previous trials (19). Yet in those trials the 28-day or intensive care unit mortality ranged between 25–32% while mortality at hospital discharge (or at 60–180 days after enrollment) was 25% to 36%. Again, this suggests that differences in exclusion criteria and illness severity (particularly the presence of septic shock) may partly explain the higher mortality in the ART study.
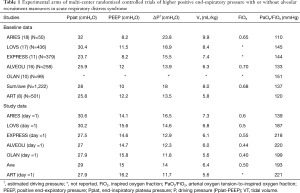
Full table
We would emphasize that 63% of subjects in the ART study received only 2 RMs on the day of enrollment (lasting a total of 6 min). Given our understanding of VILI and the accumulated science on ARM, it seems unlikely that such a brief exposure for those who tolerated the initial ARMs would have contributed to the excess mortality.
Prior studies in patients with ARDS have demonstrated that exposure to 6 h of an injurious ventilatory pattern (i.e., VT of 12 mL/kg, ∆P of 26 cmH2O, PEEP of 5 cmH2O) causes increased plasma levels of proinflammatory mediators (measured at 1 h) which subsequently decrease 6 hours after the return to lung-protective ventilation (20). The data also implied that the simultaneous rise in alveolar proinflammatory mediators estimated over the first 1 h was negligible. Mechanistically this suggests that VILI (and hence VILI-associated mortality) is more likely related to a sustained exposure to an injurious pattern in those with a hyper-inflammatory state. Notwithstanding, this evidence does not preclude the possibility that in those receiving multiple ARMs, repeated bursts in plasma proinflammatory mediators coupled with (or related to) mesenteric hypoperfusion/bacterial translocation may have contributed to excess mortality in the ART study.
This leaves three questions to be answered. First, is there something uniquely damaging in performing an ARM at pressures of 50–60 cmH2O that has not previously been recognized? Although we have argued that this seems unlikely (at least in those who only received the initial ARMs without apparent harm), it behooves the study investigators to perform a thorough post hoc analysis to elucidate whether all or a subset of subjects were at increased mortality risk from OLV.
Second, does atelectrauma truly pose a substantial risk for developing VILI in most patients with moderate or severe ARDS? The answer requires discussing issues related to epithelial cell wounding, as well as the distribution and magnitude of opening and closing pressures. Stress injury to epithelial cells tends to be caused by movement and/or rupture of liquid bridges and liquid plugs that cross the epithelial surface. Thus, interventions like OLV which are designed to minimize airspace fluid and plug formation are generally lung protective (21).
By increasing end-expired lung volume and transalveolar pressures, OLV reduces the probability of airspace occlusion by liquid bridges, while also promoting the translocation of edema fluid from airspaces to the interstitium (21). If lung compliance also improves (signifying increased alveolar surface area to accommodate tidal ventilation), reduced tidal stress is an additional benefit. Over a period of several days, however, damage from interfacial stress may no longer be of major concern as airspace fluid will have largely solidified. Thus, a sustained risk from this aspect of shear-injury subsumed under the rubric of atelectrauma may not be as great as previously supposed.
In addition, threshold opening pressures in ARDS appears to follow a bimodal distribution with most lung units requiring a Pplat of only 20–35 cmH2O to achieve recruitment (22). It has also been demonstrated that following recruitment, subsequent, consequential derecruitment mostly seems to occur when PEEP is ≤10 cmH2O (23). Therefore, as a general guide setting PEEP between 10–16 cmH2O along with a VT producing driving pressures (∆P) ≤15 cmH2O, is probably sufficient to manage most ARDS cases. The caveat being that it also reduces prolonged exposure to severe hyperoxia (e.g., FiO2 >0.70). This approach was illustrated in a study whereby ARDS subjects ventilated at a 6 mL/kg VT had their PEEP raised by 4 cmH2O above the lower inflection point (i.e., from 11 to 16 cmH2O) (24). This caused an increase in Pplat from 28 to 32 cmH2O that was associated with substantial increases in mean end-expiratory lung volume and improved oxygenation.
Moreover, others have reported that only about 25% of subjects require threshold opening pressures >50 cmH2O for “full alveolar recruitment” (22). Therefore, in answer to the second question, atelectrauma is unlikely to be a significant problem when the targeted Pplat doesn’t reach these threshold pressures on a breath-to-breath basis. Hence, the derecruited lung tissue in these “recalcitrant” dorsal-caudal regions is functionally protected from shear injury. Historically, atelectrauma was likely a frequent occurrence in ARDS, and major contributor to VILI, when a large VT/ low PEEP strategy was pervasive; but not necessarily in the era of lung protective ventilation.
A final question raised by the ART investigation is when and in whom do the benefits of OLV outweigh the risks? To categorically reject the use of ARM in ARDS would be unwise as there is a minority of severe ARDS cases wherein high PEEP, prone positioning and even extracorporeal membrane oxygenation are insufficient. In our experience, this tends to occur when severe ARDS is complicated by substantial alterations in chest wall compliance and/or when lobar collapse is superimposed upon diffuse inflammation.
As an example, when ARDS is complicated by intra-abdominal hypertension related to injury, infection or morbid obesity, intra-abdominal pressures transmitted to the chest cavity may exceed 25 mmHg (34 cmH2O) (25). These situations usually occur in “extra-pulmonary ARDS” wherein the lungs are often amenable to recruitment. OLV using ARM threshold opening pressures of 50–60 cmH2O may be necessary to overcome: (I) superimposed pressures from increased chest wall elastance and overlying edematous lung/mediastinal contents acting on the dorsal-caudal lung regions; and (II) high surface tension forces and increased viscosity of airway lining fluid/edema.
Given common “provocations” for derecruitment associated with routine care (e.g., intra-hospital transports, procedures, etc.), ARM may continue to play an important role in limiting exposure to hypoxemia, hyperoxia and increased tidal stress, even in ARDS patients. Further analysis of the ART study data as well as additional studies is needed to help inform the use of ARM in ARDS. Until further evidence suggests otherwise, OLV should still be considered on a case-by-case basis as a rescue therapy.
Acknowledgements
Mr. Kallet has previously received research support from Nihon Kohden for work on capnography.
Footnote
Conflicts of Interest: Mr. Kallet is on the board of directors of the Asthma & Allergy Prevention Company and has served as a critical care consultant for the Getinge Group. The other author has no conflicts of interest to declare.
References
- Suzumura EA, Amato MB, Cavalcanti AB. Understanding recruitment maneuvers. Intensive Care Med 2016;42:908-11. [Crossref] [PubMed]
- Constantin JM, Cayot-Constantin S, Roszyk L, et al. Response to recruitment maneuver influences net alveolar fluid clearance in acute respiratory distress syndrome. Anesthesiology 2007;106:944-51. [Crossref] [PubMed]
- Bendixen HH, Hedley-Whyte J, Laver MB. Impaired oxygenation in surgical patients during general anesthesia with controlled ventilation. N Engl J Med 1963;269:991-6. [Crossref] [PubMed]
- Lachmann B. Open up the lung and keep the lung open. Intensive Care Med 1992;18:319-21. [Crossref] [PubMed]
- Katz JA, Ozanne GM, Zinn SE, et al. Time course and mechanism of lung-volume increases with PEEP in acute pulmonary failure. Anesthesiology 1981;54:9-16. [Crossref] [PubMed]
- Ranieri VM, Brienza N, Santostasi S, et al. Impairment of lung and chest wall mechanics in patients with acute respiratory distress syndrome: The role of abdominal distension. Am J Respir Crit Care Med 1997;156:1082-91. [Crossref] [PubMed]
- Goligher EC, Hodgson CL, Adhikari NKJ, et al. Lung recruitment maneuvers for adult patients with acute respiratory distress syndrome. Ann Am Thorac Soc 2017;14:S304-11. [Crossref] [PubMed]
- Writing Group for the Alveolar Recruitment for Acute Respiratory Distress Syndrome Trial (ART) Investigators, Cavalcanti AB, Suzumura ÉA, et al. Effect of Lung Recruitment and Titrated Positive End-Expiratory Pressure (PEEP) vs Low PEEP on Mortality in Patients With Acute Respiratory Distress Syndrome: A Randomized Clinical Trial. JAMA 2017;318:1335-45. [Crossref] [PubMed]
- Acute Respiratory Distress Syndrome Network, Brower RG, Matthay MA, et al. Ventilation with lower tidal volumes as compared with traditional tidal volumes for acute lung injury and the acute respiratory distress syndrome. N Engl J Med 2000;342:1301-8. [Crossref] [PubMed]
- Kacmarek RM, Villar J. Open lung approach for acute respiratory distress syndrome: a pilot, randomized controlled trial. Crit Care Med 2016;44:32-42. [Crossref] [PubMed]
- Mercat A, Richard JC, Vielle B, et al. Positive end-expiratory pressure setting in adults with acute lung injury and acute respiratory distress syndrome. A randomized controlled trial JAMA 2008;299:646-55. [Crossref] [PubMed]
- Gattinoni L, Pelosi P, Suter PM, et al. Acute respiratory distress syndrome caused by pulmonary and extrapulmonary disease. Different syndromes?. Am J Respir Crit Care Med 1998;158:3-11. [Crossref] [PubMed]
- deMatos GF, Stanzani F, Passos RH, et al. How large is the lung recruitability in early acute respiratory distress syndrome: a prospective case series of patients monitored by computed tomography. Crit Care 2012;16:R4. [Crossref] [PubMed]
- Özcan PE, Akıncı ÖI, Edipoğlu I, et al. Effects of different recruitment maneuvers on bacterial translocation and ventilator-induced lung injury. Ulus Travma Acil Cerrahi Derg 2016;22:127-33. [PubMed]
- Claesson J, Lehtipalo S, Winsö O. Do lung recruitment maneuvers decrease gastric mucosal perfusion. Intensive Care Med 2003;29:1314-21. [Crossref] [PubMed]
- Brower RG, Lanken PN, MacIntyre N, et al. Higher versus lower positive end-expiratory pressures in patients with acute respiratory distress syndrome. N Engl J Med 2004;351:327-36. [Crossref] [PubMed]
- Meade MO, Cook DJ, Guyatt GH, et al. Ventilation strategy using low tidal volumes, recruitment maneuvers, and high positive end-expiratory pressure for acute lung injury and acute respiratory distress syndrome. A randomized controlled trial. JAMA 2008;299:637-45. [Crossref] [PubMed]
- Villar J, Kacmarek RM, Perez-Mendez L, et al. A high positive end-expiratory pressure, low tidal volume ventilatory strategy improves outcomes in persistent acute respiratory distress syndrome: a randomized controlled trial. Crit Care Med 2006;34:1311-8. [Crossref] [PubMed]
- Amato MBP, Meade MO, Slutsky AS, et al. Driving pressure and survival in acute respiratory distress syndrome. N Engl J Med 2015;372:747-55. [Crossref] [PubMed]
- Stuber F, Wrigge H, Schroeder S, et al. Kinetic and reversibility of mechanical ventilation-asociated pulmonary and systemic inflammatory response in patients with acute lung injury. Intensive Care Med 2002;28:834-41. [Crossref] [PubMed]
- Hubmayr RD, Kallet RH. Understanding pulmonary stress-strain relationships in severe ARDS and its implications for designing a safer approach to setting the ventilator. Respir Care 2018;63:219-26. [Crossref] [PubMed]
- Borges JB, Okamato VN, Matos GFJ, et al. Reversibility of lung collapse and hypoxemia in early acute respiratory distress syndrome. Am J Respir Crit Care Med 2006;174:268-78. [Crossref] [PubMed]
- Crotti S, Masheroni D, Caironi P, et al. Recruitment and derecruitment during acute respiratory failure: a clinical study. Am J Respir Crit Care Med 2001;164:131-40. [Crossref] [PubMed]
- Richard JC, Maggiore SM, Jonson B, et al. Influence of tidal volume on alveolar recruitment. Am J Respir Crit Care Med 2001;163:1609-13. [Crossref] [PubMed]
- Malbrain ML, Cheatham ML, Kirkpatrick A, et al. Results from the international conference of experts on intra-abdominal hypertension and abdominal compartment syndrome. I Definitions. Intensive Care Med 2006;32:1722-32. [Crossref] [PubMed]