The effect of continuous positive airway pressure on pulmonary function may depend on the basal level of forced expiratory volume in 1 second
Introduction
Chronic obstructive pulmonary disease (COPD) and obstructive sleep apnea (OSA) represent two of the most prevalent chronic respiratory disorders, affecting respectively 10% and 5% of the adult population (1).
The health burden associated with the coexistence of both disorders, also referred to as overlap syndrome (OS) (2), is far greater than the sum of the two components in terms of morbidity, incidence of COPD exacerbations and mortality (2-4).
Treatment with continuous positive airway pressure (CPAP) has proven to significantly decrease the rate of COPD exacerbations and mortality in patients with OS, lowering the risk of both conditions to levels associated with COPD alone (5). Notably, less is known about the effect of CPAP treatment on pulmonary function, and the scarce existing data are conflicting (6,7). Furthermore, studies on this topic are small and, consequently, their results are inconclusive.
A previous study documented a deterioration in forced expiratory volume in 1 second (FEV1) in the 2 years following the introduction of CPAP therapy (6) with a linear correlation between the average hours of daily CPAP use and the percent decrease in FEV1. Another study (8) found significant improvements in arterial blood gases (ABGs) and pulmonary function tests at 6 and 18 months after the beginning of CPAP therapy. Finally, Toraldo and colleagues (7), in line with the last reported study, demonstrated a significant increase in FEV1 and FEV1/forced vital capacity (FVC) ratio compared to baseline values even after 24 months of CPAP treatment.
The primary aim of our study was to evaluate the effect of 1 year of CPAP treatment on ABGs and respiratory function tests in a series of patients with OS and to relate the treatment results to the severity of baseline airway obstruction.
The secondary aim was to search for predictors of changes in the evaluated parameters.
Methods
Patients
We retrospectively collected data of patients admitted to the Pulmonary Rehabilitation Unit of the Istituti Clinici Scientifici Maugeri in Pavia (Italy) from January 2013 to January 2016 who met all of the following criteria: a diagnosis of overlap between COPD and OSA, clinical stability for at least 3–4 weeks (as assessed by the absence of any worsening of symptoms and stability in ABGs (no acidosis, defined as pH ≤7.35), a prescription of domiciliary CPAP, and a follow-up in our hospital for at least 1 year after CPAP prescription.
Patients were excluded from the study if they had other sleep or respiratory disorders (e.g., resless leg syndrome, insomnia), an average use <4 hours per night (assessed 3 months after CPAP prescription by the analysis of the memory card of the ventilator) and if 1-year follow-up data were lacking or incomplete. Other exclusion criteria were major uncontrolled medical or psychiatric conditions; cancer; surgery of the upper airways, nose, sinuses or middle ears in the 3 months before enrollment; signs or symptoms of acute exacerbation of COPD; or an ABG pH ≤7.35.
Measurements
Spirometry and lung volumes were measured with the subjects sitting in a body plethysmograph following the American Thoracic Society/European Respiratory Society (ATS/ERS) recommendations (9,10). The reference values reported in the Gold Guidelines were used (11). The diagnosis and severity of COPD were established if an irreversible obstructive functional defect (FEV1/FVC ratio ≤70%) together with a past history of cigarette smoking were present, in accordance with the Global Initiative for Chronic Obstructive Lung Disease (GOLD) (11).
ABG samples were taken from the radial artery with the spontaneously breathing awake patient in the sitting position. The samples were analyzed with a blood gas analyzer (ABL 520-Radiometer, Copenhagen, Denmark).
A diagnosis of OSA was made according to standard criteria (12): all patients underwent standard sleep evaluation and full polysomnography, analyzed according to the American Academy of Sleep Medicine (AASM) 2007 Guidelines (13). A diagnosis of OS was made when COPD patients presented clinical symptoms and an apnea-hypopnea index (AHI) of 10 or more.
After the diagnosis of OSA, patients received CPAP adaptation and treatment. A full-night manual CPAP titration in a laboratory was performed according to AASM Guidelines (14) to identify the best level of pressure to treat the sleep disorder.
Treatment efficacy was confirmed by a further overnight polygraphy and, once a good level of tolerance was reached (defined as an average use of at least four hours/night, as indicated by the memory card of the device), a final prescription of treatment was made. In accordance with the Italian health system, when a domiciliary CPAP is prescribed, the policy is to rotate among different models and manufacturers. All of these models are invariably equipped with software that enables us to record and analyze data.
Patients were followed up 3 months later to check for adherence to the therapy and possible technical or clinical problems. One year later, a full polysomnography, as well as pulmonary functional tests and ABG measurements, were repeated.
Patients with a more severe functional defect received an intermediate pulmonary function test evaluation 6 months after CPAP prescription and discharge. This intermediate test was not considered in this study.
Study design
Demographic data, functional tests, ABGs, full polysomnography, comorbidities (1 or more among arterial hypertension, ischemic cardiopathy and arrhythmia, diabetes and renal failure) recorded at baseline and 1 year later were collected.
The study was approved by the Ethics Committee of the Istituti Clinici Scientifici Maugeri (Number 844 CEC).
Statistical analysis
The distributions of categorical variables were described by counts and frequencies (%). Quantitative variable distributions were described by the median (25th–75th percentiles). The presence of statistically significant differences in terms of quantitative variables characterized by a continuous distribution between binary independent variables was tested by the Wilcoxon rank sum test. The presence of statistically significant variations in terms of quantitative variables between time points was tested by the Wilcoxon signed rank test. The association between categorical variables was tested either by Fisher’s exact test or Pearson’s chi-squared test with Yates’ continuity correction as appropriate. Multivariate logistic regression coupled with a stepwise features selection procedure was used to identify the most informative set of variables associated with binary outcomes. Informative thresholds were identified by a classification tree-based approach. Linear regression was used to estimate the correlation between quantitative variables and to quantify the impact of potential confounders. The significance threshold was set at P<0.05. All statistical analyses were performed using R statistical Software (www.r-project.org).
Results
A total of 92 patients (74 males) who were hospitalized during the observation period met our inclusion criteria. The median age was 66 (60.2–74.0). Patients’ demographic and clinical data at baseline are reported in Table 1. The median FEV1% value was 62.1% (46.8–79.5%). According to the GOLD classification, 23 patients (25.0%) were distributed in class 1, 43 (46.7%) in class 2, 20 (21.7%) in class 3 and 6 (6.5%) in class 4.
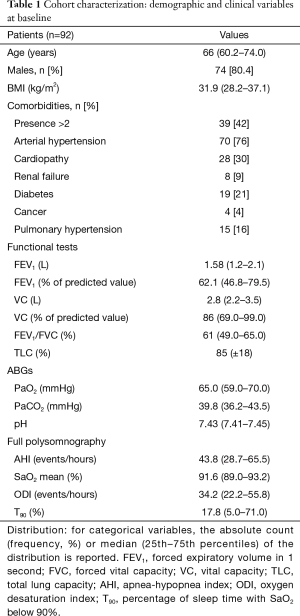
Full table
The median AHI was 43.8 (28.7–65.5) events per hour with a median nocturnal saturation of 91.6% (89.0–93.2%); 17 of 92 patients (18.47%) showed a pCO2 >45 mmHg.
A comparison between hypercapnic and normocapnic patients showed that the two populations were not statistically different in terms of anthropometric variables and comorbidities. However, hypercapnic patients had more severe airway obstruction, more severe hypoxemia at baseline and worse AHI and nocturnal gas exchanges, as recorded during polysomnography (Table 2).
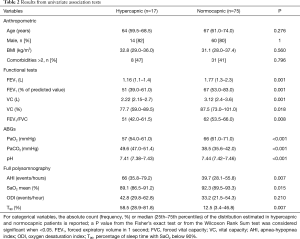
Full table
A multivariate logistic regression analysis showed that AHI [OR =1.08 (1.02–1.16), P=0.012], the percentage of sleep time with SaO2 below 90% (T90) [OR =1.03 (1.01–1.06), P=0.029], vital capacity (VC) [OR =0.20 (0.05–0.57), P=0.008] and FEV1/FVC [OR =0.91 (0.83–0.97), P=0.01] at baseline were the strongest predictors of hypercapnia. Baseline characteristics (age, gender and comorbidities) did not significantly influence the probability of being hypercapnic (P>0.30); therefore, these were not included in the multivariate model.
After 1 year of treatment, we observed a significant improvement in pO2 [median value 65.0 (59.0–70.0) vs. 71 (64.8–77.1) mmHg, at baseline and after 1 year, respectively, P=5.6×10−9] and pCO2 [39.8 (36.2–43.5) vs. 38.3 (32.3–44.2) mmHg respectively, P=0.0001] in the general population (Figure 1).
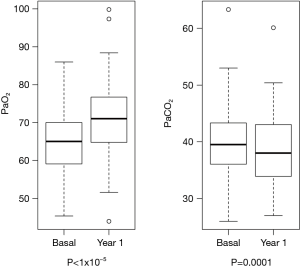
Both improvements appeared significantly greater in the subgroup of hypercapnic patients compared to the normocapnic ones [median pO2 57 (54–61.05) vs. 71 (63.0–78.0) mmHg, P=0.0008, median pCO2 49.6 (47.0–51.4) vs. 43.8 (38.0–45.0) mmHg, P=0.005 at baseline and after one year, respectively] (Figure 2).
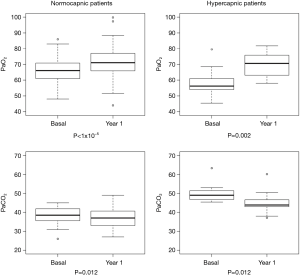
In contrast, FEV1, VC and FEV1/VC did not show a significant change (P>0.05) in the general population, except for the subgroup of hypercapnic patients in which there was a significant improvement in FEV1 after 1 year [70 (−70 to 200) mL, P=0.02]. No significant variation was detected in body mass index (BMI) [31.9 (28.2–37.1) vs. 31.7 (28.2–34.9) kg/m2 at baseline and after 1 year, respectively, P>0.05], smoking habits, or in the inhaled therapy taken, after 1 year of CPAP treatment.
Linear regression analysis revealed a significant inverse correlation between the change in FEV1 after 1 year, expressed as an absolute value, and the basal FEV1 expressed as a percentage of the predicted value (Figure 3).
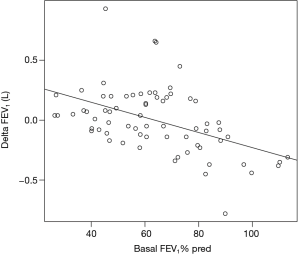
A conditional inference tree-based approach identified an FEV1 baseline threshold of 79.1% of the predicted value under and above which the FEV1 change after 1 year was significantly different (P=1.22×10−6) (Figure 4). Multivariate linear regression showed that the difference observed in FEV1 variation was not influenced by potential confounders represented by age, gender and comorbidities (P=5.5×10−6).
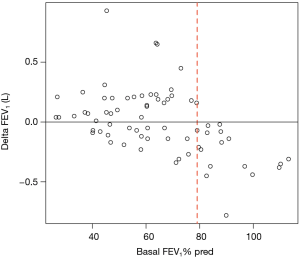
In particular, in patients with an FEV1 at baseline below that threshold, we found a statistically significant improvement in FEV1 after 1 year of CPAP [median value 70 (−70 to 200) mL, P=0.001]. In contrast, in patients with a baseline FEV1 above the same threshold, we observed a statistically significant decrease in FEV1 after 1 year [median value −270 (−370 to −130) mL, P=3.05×10−5].
No difference in the median CPAP level used in patients with a basal FEV1 under or above the threshold of 79.1% was found [11 (10.0–14.0) and 11 (8.5–12.5) respectively, P=0.489]. Median CPAP levels according to patient’s functional class are reported in more detail in Table 3.
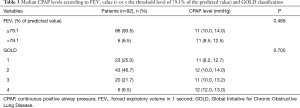
Full table
Discussion
The main findings of this observational study of patients with OS can be summarized as follows: after 1 year of CPAP treatment, (I) ABG values significantly improved, particularly in patients with basal pCO2 >45 mmHg, and (II) no significant change in respiratory function emerged in the whole population. However, notably, there was (III) a significant change in FEV1 in hypercapnic patients and (IV) a significant change in FEV1 that was inversely proportional to its basal value expressed as the percentage of the predicted value (FEV1%) in the whole population. In particular, a lower basal FEV1% led to a greater increase in FEV1 after 1 year. Above a FEV1% of 79.1%, there was an inversion of this trend.
Data on lung function changes after CPAP treatment in OS are heterogeneous in the literature. In 10 patients able to tolerate CPAP for at least 3 months, Mansfield and colleagues (15) found a significant improvement in FEV1 after a minimum of 8 months of CPAP treatment. Toraldo and colleagues (7) described an increase in FEV1 of 15% (P<0.0015) at 24 months compared to baseline values.
In contrast, O’Brien and Whitman (6) reported a significant decrease in FEV1 and FVC in overlap patients who used CPAP for more than 4 hours/day compared to non-compliant patients. Moreover, they found a strong correlation between the average daily hours of CPAP use and the percent decrease in FEV1. Similarly to O’Brien and Whitman (6), in our whole patient population, we found no differences in pulmonary function tests after 1 year of treatment. However, in contrast with previous studies, we stratified patients according to the degree of basal airflow obstruction. This stratification demonstrated a different effect of CPAP on respiratory function according to the basal FEV1% values.
Notably, the improvement and the decrease we observed under and above the FEV1% threshold, respectively, were independent from BMI, smoking habits and inhaled therapy variations after 1 year. They were also independent of the CPAP level used.
A possible explanation for the observed FEV1 changes could lie in the natural history of the functional decline of COPD patients which, according to recent evidence, appears to be more accelerated and more relevant in patients in the early stages than in the late stages (16). In fact, as shown by Tantucci and Modina (16), the average annual decline in FEV1 in GOLD stages II and III ranged between 47 and 79 mL/year, respectively, and this decline was less than 35 mL/year in GOLD stage IV. However, no clear data are available on the rate of decline in GOLD stage I.
Therefore, in our population, the CPAP effect may have been sufficient to overcome the natural evolution of lung function in patients with worse pulmonary function, whereas in patients in stage I with probable greater intrinsic annual functional decline, treatment may have been unable to counter the natural evolution of the disease.
A further possible explanation of our findings may lie in airway inflammation exacerbation related to OSA in COPD patients (17), which naturally affects lung function. Wang and coworkers (17) showed a significantly higher percentage of neutrophils, TNFα and IL-8 levels in the bronchoalveolar lavage fluid of overlap patients compared to patients with only COPD; this difference was proportional to OSA severity. The authors also found that the inflammation response significantly decreased after CPAP treatment (17). However, these data were only demonstrated in overlap patients with a moderate to severe functional defect. No data on the level of inflammation induced by OSA in patients with mild COPD are available. Therefore, we may hypothesize that a lower level of airway inflammation in patients with mild COPD and OSA could explain the absence of a positive effect of CPAP on respiratory function.
In line with previous studies, we found an improvement in ABGs after 1 year of CPAP treatment. In the study by de Miguel et al. (8), statistically significant increases in PaO2 and decreases in PaCO2 were observed 6 months after starting CPAP therapy, and the improvement in ABGs was greater in the group of patients with hypercapnia at baseline. Mansfield et al. (15) also found a significant improvement in ABGs in patients who were compliant with nasal CPAP.
In our study, hypercapnic patients appeared to benefit more from the ventilator treatment than the normocapnic patients, both in terms of gas exchanges and respiratory function.
This result underlines an important issue in the clinical practice: hypercapnic respiratory failure in COPD patients must be investigated for the presence of OSA, and a first attempt at using CPAP therapy should be made.
The improvements in function and ABG may be related to some CPAP effects. In fact, CPAP can reduce inspiratory effort during sleep in patients with COPD, as documented by significant reductions in the tidal excursions of esophageal pressure (18). Furthermore, CPAP may lead to an improvement in the ventilation/perfusion ratio. In fact, by correcting obstructive events and limiting the consequent increase in the work of breathing, CPAP acts on alveolar hypoventilation and corrects nocturnal hypoxemia and hypercapnia. The reversal of nocturnal blood gases and the resetting of chemoreceptor sensitivity are then responsible for the improvements in diurnal ABGs (19). Finally, the normalization of arterial pCO2 in patients who were hypercapnic at baseline may reduce intramural bronchial edema, which may be determined by hypercapnia-induced renal vasoconstriction (20). This may explain the significant improvement of FEV1 in the subgroup of hypercapnic overlap patients.
Our study has some limitations. Firstly, this is a retrospective study, and data on body plethysmography at 1 year are thus not available for all patients.
In addition, data concerning acute exacerbations of COPD are lacking, and therefore the effect of CPAP treatment on their incidence in the different functional stages is also unknown. As we surmised that the improvement in pulmonary function may be related to a decrease in the number of exacerbations, a prospective study exploring this issue could be interesting.
Regarding the limited sample size potentially affecting this study, post hoc calculations were performed to estimate the statistical power reached for the variations observed in terms of pO2 and pCO2 from baseline to year 1 measurements. Calculations were also performed to assess the differences in terms of FEV1 variations between subjects with baseline FEV1 ≤79.1% and the rest of the cohort. The findings provide reassurance of the robustness of these results, showing sufficient statistical power (≥0.96) for the three tests.
Finally, the follow-up of our patients lasted only 1 year, as data on the subsequent years are lacking. It would have been interesting to continue to monitor both patients, with functional improvements and those with functional losses, to gain insights into their functional evolution.
Conclusions
In conclusion, our retrospective study of patients affected with OSA and COPD found that despite a significant improvement in gas exchanges in the whole population, after 1 year of CPAP treatment the pattern of respiratory function was different according to the basal degree of airway obstruction. In patients with an FEV1 lower than 79.1%, there was a significant improvement in FEV1 that was inversely proportional to the basal value, whereas patients with an FEV1 higher than 79.1% experienced a significant worsening of their respiratory function. Further observational studies are needed to confirm these findings.
Acknowledgements
None.
Footnote
Conflicts of Interest: The authors have no conflicts of interest to declare.
Ethical Statement: The study was approved by the Ethics Committee of the Istituti Clinici Scientifici Maugeri (Number 844 CEC) and written informed consent was obtained from all patients.
References
- Lee R, McNicholas WT. Obstructive sleep apnea in chronic obstructive pulmonary disease patients. Curr Opin Pulm Med 2011;17:79-83. [Crossref] [PubMed]
- Flenley DC. Sleep in chronic obstructive lung disease. Clin Chest Med 1985;6:651-61. [PubMed]
- Ioachimescu OC, Teodorescu M. Integrating the overlap of obstructive lung disease and obstructive sleep apnoea: OLDOSA syndrome. Respirology 2013;18:421-31. [Crossref] [PubMed]
- Owens RL, Malhotra A. Sleep-Disordered Breathing and COPD: The Overlap Syndrome. Respir Care 2010;55:1333-44. [PubMed]
- Marin JM, Soriano JB, Carrizo SJ, et al. Outcomes in patients with chronic obstructive pulmonary disease and obstructive sleep apnea: the overlap syndrome. Am J Respir Crit Care Med 2010;182:325-31. [Crossref] [PubMed]
- O’Brien A, Whitman K. Lack of Benefit of Continuous Positive Airway Pressure on Lung Function in Patients with Overlap Syndrome. Lung 2005;183:389-404. [Crossref] [PubMed]
- Toraldo DM, De Nuccio F, Nicolardi G. Fixed-pressure nCPAP in patients with obstructive sleep apnea (OSA) syndrome and chronic obstructive pulmonary disease (COPD): a 24-month follow-up study. Sleep Breath 2010;14:115-23. [Crossref] [PubMed]
- de Miguel J, Cabello J, Sánchez-Alarcos JM, et al. Long-Term Effects of Treatment with Nasal Continuous Positive Airway Pressure on Lung Function in Patients with Overlap Syndrome. Sleep Breath 2002;6:3-10. [Crossref] [PubMed]
- Wanger J, Clausen JL, Coates A, et al. Standardisation of the measurement of lung volumes. Eur Respir J 2005;26:511-22. [Crossref] [PubMed]
- Miller MR, Hankinson J, Brusasco V, et al. Standardisation of spirometry. Eur Respir J 2005;26:319-38. [Crossref] [PubMed]
- Vogelmeier CF, Criner GJ, Martinez FJ, et al. Global Initiative for Chronic Obstructive Lung Disease Global strategy for the diagnosis, management, and prevention of chronic obstructive pulmonary disease 2017 Report: GOLD executive summary. Am J Respir Crit Care Med 2017;195:557-82. [Crossref] [PubMed]
- American Academy of Sleep Medicine. International Classification of Sleep Disorders 2nd edition: Diagnostic and coding manual. American Academy of Sleep Medicine, 2005. American Academy of Sleep Medicine International Classification of Sleep Disorders 3rd edition. Darien, IL: American Academy of Sleep Medicine, 2014.
- Berry RB, Brooks R, Gamaldo CE, et al. for the American Academy of Sleep Medicine. The AASM Manual for the Scoring of Sleep and Associated Events: Rules, Terminology and Technical Specifications. Version 2.4. Darien, IL: American Academy of Sleep Medicine, 2017.
- Kushida CA, Chediak A, Berry RB, et al. Positive Airway Pressure Titrsation Task Force; American Academy of Sleep Medicine. Clinical Guidelines for the manual titration of positive airway pressure in patients with obstructive sleep apnea. J Clin Sleep Med 2008;4:157-71. [PubMed]
- Mansfield D, Naughton MT. Effects of continuous positive airway pressure on lung function in patients with chronic obstructive pulmonary disease and sleep disordered breathing. Respirology 1999;4:365-70. [Crossref] [PubMed]
- Tantucci C, Modina D. Lung function decline in COPD. Int J Chron Obstruct Pulmon Dis 2012;7:95-9. [Crossref] [PubMed]
- Wang Y, Hu K, Liu K, et al. Obstructive sleep apnea exacerbates airway inflammation in patients with chronic obstructive pulmonary disease. Sleep Med 2015;16:1123-30. [Crossref] [PubMed]
- Petrof BJ, Kimoff RJ, Levy RD, et al. Nasal continuous positive airway pressure facilitates respiratory muscle function during sleep in severe chronic obstructive pulmonary disease. Am Rev Respir Dis 1991;143:928-35. [Crossref] [PubMed]
- Rossi A, Santos C, Roca J, et al. Effects of PEEP on VA/Q mismatching in ventilated patients with chronic airflow obstruction. Am J Respir Crit Care Med 1994;149:1077-84. [Crossref] [PubMed]
- Hemlin M, Ljungman S, Carlson J, et al. The effects of hypoxia and hypercapnia on renal and heart function, haemodynamics and plasma hormone levels in stable COPD patients. Clin Respir J 2007;1:80-90. [Crossref] [PubMed]